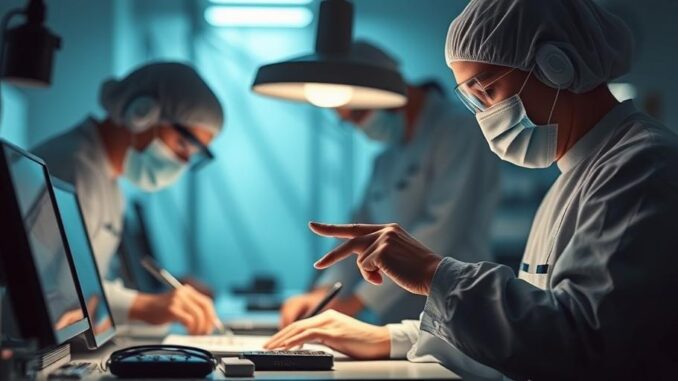
Hemostasis: A Comprehensive Review of Mechanisms, Technologies, Market Dynamics, and Future Directions
Many thanks to our sponsor Esdebe who helped us prepare this research report.
Abstract
Hemostasis, the intricate physiological process of arresting bleeding, is crucial for maintaining vascular integrity and preventing exsanguination. This review provides a comprehensive analysis of hemostasis, encompassing its underlying mechanisms, including primary hemostasis, secondary hemostasis (coagulation cascade), and tertiary hemostasis (fibrinolysis). Furthermore, it delves into the diverse array of hemostatic technologies, categorized into surgical, pharmacological, and device-based approaches. The report examines the global hemostasis market, highlighting key players, market size, growth forecasts, and emerging trends. We also address the challenges and complications associated with hemostasis procedures and explore various clinical applications across different medical specialties. Finally, we offer insights into future research directions, including the development of novel hemostatic agents, personalized hemostasis management, and advanced diagnostic tools. This review aims to provide experts in the field with an updated and insightful overview of the multifaceted landscape of hemostasis.
Many thanks to our sponsor Esdebe who helped us prepare this research report.
1. Introduction
Hemostasis, derived from the Greek words “haimo” (blood) and “stasis” (stopping), is the essential physiological process that prevents blood loss following vascular injury. Its complexity is underscored by the delicate balance between promoting clot formation to stop bleeding and preventing excessive thrombosis that could lead to vascular occlusion and ischemic events. Dysregulation of hemostasis can manifest as either bleeding disorders (e.g., hemophilia, thrombocytopenia) or thrombotic conditions (e.g., deep vein thrombosis, pulmonary embolism), both posing significant clinical challenges. The hemostasis system is a finely tuned regulatory network involving blood vessels, platelets, coagulation factors, natural anticoagulants, and fibrinolytic proteins [1].
Understanding the intricacies of hemostasis is paramount for developing effective therapeutic strategies to manage bleeding and thrombotic disorders. Over the years, significant advancements have been made in hemostatic technologies, ranging from traditional surgical techniques to sophisticated pharmacological agents and innovative device-based solutions. The acquisition activities within the hemostasis market, as highlighted by Merit Medical’s expansion, signal the dynamic nature and commercial importance of this field. This review aims to provide a comprehensive overview of hemostasis, encompassing its physiological mechanisms, therapeutic interventions, market trends, and future directions, with a focus on aspects of relevance to experts in the field.
Many thanks to our sponsor Esdebe who helped us prepare this research report.
2. Physiological Mechanisms of Hemostasis
Hemostasis is a complex cascade of events that can be broadly divided into three overlapping stages: primary hemostasis, secondary hemostasis (coagulation cascade), and tertiary hemostasis (fibrinolysis). This section will elaborate on these stages and the key components involved.
2.1 Primary Hemostasis
Primary hemostasis is the immediate response to vascular injury and involves vasoconstriction and platelet plug formation. When a blood vessel is damaged, the initial response is vasoconstriction, which reduces blood flow to the injured site. This vasoconstriction is mediated by local factors such as endothelin released from damaged endothelial cells, and neural reflexes [2].
Platelets, small anucleate cell fragments derived from megakaryocytes, play a crucial role in primary hemostasis. Following endothelial damage, subendothelial collagen is exposed, which triggers platelet adhesion. Von Willebrand factor (vWF), a large multimeric glycoprotein, acts as a bridge between collagen and platelet glycoprotein Ib-IX-V receptor (GPIb-IX-V). Upon adhesion, platelets undergo activation, which involves shape change, granule release, and aggregation. Activation is mediated by various agonists, including adenosine diphosphate (ADP), thromboxane A2 (TXA2), and thrombin. Activated platelets release granules containing substances like ADP and TXA2, which further amplify platelet activation and aggregation. Platelets also express the glycoprotein IIb/IIIa (GPIIb/IIIa) receptor, which, upon activation, binds fibrinogen, leading to platelet cross-linking and the formation of a platelet plug. This plug provides a temporary seal to stop bleeding [3].
2.2 Secondary Hemostasis: The Coagulation Cascade
The coagulation cascade is a series of enzymatic reactions that ultimately lead to the formation of a fibrin clot, which strengthens the initial platelet plug. The coagulation cascade involves a complex interplay of coagulation factors, most of which are serine proteases. Historically, the coagulation cascade was described as two separate pathways: the intrinsic and extrinsic pathways, converging on the common pathway. However, the cell-based model of coagulation is now the accepted model, which more accurately reflects the in vivo processes [4].
The cell-based model consists of three overlapping phases: initiation, amplification, and propagation. Initiation occurs when tissue factor (TF), a transmembrane protein exposed on cells outside the vasculature, binds to factor VIIa (VIIa). The TF-VIIa complex activates factors IX and X, initiating the coagulation cascade. Amplification involves the activation of platelets by thrombin. Activated platelets provide a surface for the assembly of coagulation factor complexes. Propagation occurs on the platelet surface, where factor Xa (Xa) forms a complex with factor Va (Va), prothrombinase complex. The prothrombinase complex converts prothrombin to thrombin, which is the key enzyme in the coagulation cascade. Thrombin converts fibrinogen to fibrin, which polymerizes to form a fibrin clot. Factor XIIIa, activated by thrombin, cross-links fibrin polymers, further stabilizing the clot [5].
2.3 Tertiary Hemostasis: Fibrinolysis
Fibrinolysis is the process of breaking down the fibrin clot once the vascular injury has been repaired. Plasminogen, an inactive zymogen, is converted to plasmin, an active serine protease, by plasminogen activators, such as tissue plasminogen activator (tPA) and urokinase plasminogen activator (uPA). tPA is released from endothelial cells and is most active when bound to fibrin. Plasmin degrades fibrin into fibrin degradation products (FDPs), including D-dimer, which can be measured in the blood to assess fibrinolysis [6]. The fibrinolytic system is tightly regulated by inhibitors, such as plasminogen activator inhibitor-1 (PAI-1), which inhibits tPA and uPA, and alpha-2-antiplasmin, which inhibits plasmin. This regulatory mechanism ensures that fibrinolysis is localized to the site of the clot and prevents excessive clot breakdown.
Many thanks to our sponsor Esdebe who helped us prepare this research report.
3. Hemostatic Technologies
Hemostatic technologies encompass a broad range of methods designed to control bleeding, categorized into surgical, pharmacological, and device-based approaches. The selection of a specific hemostatic technique depends on factors such as the severity and location of the bleeding, patient-specific considerations, and the surgeon’s preference.
3.1 Surgical Hemostasis
Surgical hemostasis involves direct physical methods to control bleeding, often employed in open surgical procedures. Traditional surgical techniques include direct pressure, ligation (tying off blood vessels), and electrocautery (using heat to seal blood vessels). Direct pressure is a simple yet effective method for controlling superficial bleeding. Ligation involves tying off blood vessels with sutures to prevent blood flow. Electrocautery is a widely used technique that utilizes electrical current to generate heat, which coagulates blood and seals blood vessels. Variations of electrocautery include monopolar and bipolar cautery, each with specific advantages and disadvantages [7].
More advanced surgical hemostatic techniques include ultrasonic coagulation and argon beam coagulation. Ultrasonic coagulation uses high-frequency sound waves to generate heat, which coagulates blood and seals blood vessels. Argon beam coagulation uses argon gas to deliver electrical current to the bleeding site, resulting in rapid coagulation with minimal tissue damage [8]. The advent of minimally invasive surgical techniques, such as laparoscopy and robotic surgery, has led to the development of specialized surgical hemostatic devices designed for use through small incisions.
3.2 Pharmacological Hemostasis
Pharmacological hemostasis involves the use of drugs to promote blood clotting. These agents can be broadly classified into systemic and topical hemostatics. Systemic hemostatics are administered intravenously or orally to affect the coagulation cascade throughout the body. Topical hemostatics are applied directly to the bleeding site to promote local clot formation.
Systemic hemostatic agents include vitamin K, which is essential for the synthesis of several coagulation factors, and desmopressin (DDAVP), a synthetic analog of vasopressin, which increases the release of vWF and factor VIII. Recombinant factor VIIa (rFVIIa) is a potent procoagulant that can bypass deficiencies in other coagulation factors and is used in patients with hemophilia and acquired bleeding disorders [9]. Antifibrinolytic agents, such as tranexamic acid and aminocaproic acid, inhibit plasminogen activation, thereby preventing clot breakdown. These agents are widely used to reduce bleeding in surgical procedures and to manage bleeding disorders.
Topical hemostatic agents include thrombin, collagen-based products, fibrin sealants, and polysaccharide-based products. Thrombin directly converts fibrinogen to fibrin, promoting clot formation. Collagen-based products provide a matrix for platelet adhesion and aggregation. Fibrin sealants contain fibrinogen and thrombin, which, when mixed, form a fibrin clot. Polysaccharide-based products, such as chitosan and oxidized regenerated cellulose (ORC), absorb fluid and promote platelet aggregation [10].
3.3 Device-Based Hemostasis
Device-based hemostasis encompasses a range of technologies that use mechanical or physical methods to control bleeding. These devices include compression devices, vascular closure devices, and hemostatic powders and sprays.
Compression devices apply external pressure to the bleeding site to reduce blood flow and promote clot formation. These devices are commonly used in post-operative settings to prevent hematoma formation. Vascular closure devices are used to seal arterial punctures after percutaneous procedures, such as angiography and angioplasty. These devices include sutures, clips, and collagen plugs. Hemostatic powders and sprays are applied to the bleeding site to promote clot formation. These products often contain materials such as zeolite, kaolin, or synthetic polymers that absorb fluid and activate the coagulation cascade [11].
Many thanks to our sponsor Esdebe who helped us prepare this research report.
4. Global Hemostasis Market Analysis
The global hemostasis market is a substantial and growing segment of the medical device and pharmaceutical industries. The market is driven by factors such as the increasing prevalence of surgical procedures, the rising incidence of trauma and bleeding disorders, and the growing demand for advanced hemostatic products. Market reports consistently show growth trends, with projections often reaching billions of dollars in the next five to ten years. This growth is fueled by both technological advancements and an aging global population requiring more medical interventions.
4.1 Market Size and Growth Forecasts
Various market research reports estimate the global hemostasis market size and project future growth. While figures vary slightly depending on the source and methodology, a consistent trend emerges: the market is experiencing steady growth. Factors driving this growth include an increase in surgical procedures, a rising geriatric population more susceptible to bleeding disorders, and technological advancements in hemostatic products. Projections often cite a compound annual growth rate (CAGR) of 5-7% over the next several years [12]. This growth is further fueled by the increasing adoption of minimally invasive surgical techniques, which require specialized hemostatic devices.
4.2 Key Players in the Industry
The hemostasis market is characterized by a mix of large multinational corporations and smaller specialized companies. Key players in the industry include:
- Johnson & Johnson: A major player with a broad portfolio of surgical and hemostatic products.
- Baxter International: Offers a range of hemostatic and sealant products.
- Medtronic: Provides surgical solutions and advanced energy devices for hemostasis.
- CSL Behring: Specializes in plasma-derived and recombinant therapies, including coagulation factors.
- Haemonetics: Focuses on blood management solutions and hemostasis analyzers.
- Merit Medical: Expanding its presence in the hemostasis market through acquisitions, focusing on device-based solutions.
- Teleflex Incorporated: Developing and manufacturing innovative surgical instruments, devices and hemostats for various medical procedures.
These companies compete based on factors such as product innovation, pricing, distribution networks, and strategic partnerships. The competitive landscape is constantly evolving due to mergers, acquisitions, and the entry of new players with innovative technologies.
4.3 Emerging Technologies
Several emerging technologies are poised to reshape the hemostasis market. These include:
- Next-generation hemostatic agents: Development of novel agents with improved efficacy, biocompatibility, and ease of use. This includes research into biomimetic materials that mimic the natural coagulation process.
- Point-of-care (POC) hemostasis testing: Development of rapid, portable diagnostic devices that can assess a patient’s coagulation status at the point of care. POC testing allows for more timely and personalized hemostasis management.
- Artificial intelligence (AI) and machine learning (ML): Application of AI/ML algorithms to analyze hemostasis data and predict bleeding risk. These technologies can also be used to optimize hemostasis protocols and personalize treatment strategies.
- Nanotechnology: Development of nanoscale hemostatic agents that can target specific sites of bleeding and promote rapid clot formation.
- Gene Therapy: Gene therapy approaches are showing promise for treating inherited bleeding disorders, such as hemophilia, by delivering functional genes to produce missing coagulation factors [13].
Many thanks to our sponsor Esdebe who helped us prepare this research report.
5. Challenges and Complications of Hemostasis Procedures
While hemostasis procedures are generally safe and effective, they are not without potential challenges and complications. Understanding these risks is crucial for optimizing patient outcomes.
5.1 Thrombotic Complications
Excessive clot formation can lead to thrombotic complications, such as deep vein thrombosis (DVT), pulmonary embolism (PE), and arterial thrombosis. These complications can be life-threatening and require prompt treatment. Factors that increase the risk of thrombotic complications include patient-specific factors (e.g., hypercoagulable state, obesity, immobility) and procedure-related factors (e.g., prolonged surgery, extensive tissue damage). The use of prophylactic anticoagulation can help to reduce the risk of thrombotic complications, but it also increases the risk of bleeding.
5.2 Bleeding Complications
Paradoxically, hemostasis procedures can also lead to bleeding complications, particularly when anticoagulants or antiplatelet agents are used. Bleeding complications can range from minor bruising to life-threatening hemorrhage. Factors that increase the risk of bleeding complications include patient-specific factors (e.g., thrombocytopenia, coagulopathy, renal insufficiency) and procedure-related factors (e.g., complex surgery, use of multiple hemostatic agents). Careful patient selection and meticulous surgical technique are essential to minimize the risk of bleeding complications.
5.3 Infection
The use of hemostatic agents and devices can increase the risk of infection. Some hemostatic materials can provide a nidus for bacterial growth. Meticulous sterile technique and the use of prophylactic antibiotics can help to reduce the risk of infection.
5.4 Allergic Reactions
Some hemostatic agents, particularly those derived from animal sources (e.g., bovine thrombin, porcine collagen), can cause allergic reactions. Allergic reactions can range from mild skin rashes to severe anaphylaxis. Patients with known allergies to animal products should be carefully screened before the use of these agents.
Many thanks to our sponsor Esdebe who helped us prepare this research report.
6. Clinical Applications of Hemostasis
Hemostasis plays a crucial role in a wide range of clinical applications across different medical specialties. This section highlights some of the key areas where hemostatic techniques are employed.
6.1 Surgical Procedures
Hemostasis is an integral part of virtually all surgical procedures. Surgeons use a variety of hemostatic techniques to control bleeding during surgery, ranging from traditional methods like ligation and electrocautery to more advanced techniques like ultrasonic coagulation and fibrin sealants. The choice of hemostatic technique depends on the type of surgery, the location and severity of the bleeding, and the surgeon’s preference. Effective hemostasis is essential for minimizing blood loss, improving surgical outcomes, and reducing the risk of complications.
6.2 Trauma Management
Trauma patients often present with severe bleeding, which can be life-threatening. Rapid and effective hemostasis is critical for stabilizing trauma patients and preventing exsanguination. Hemostatic techniques used in trauma management include direct pressure, tourniquets, wound packing with hemostatic agents, and surgical intervention. The development of advanced hemostatic agents and devices has significantly improved the outcomes of trauma patients with severe bleeding [14].
6.3 Management of Bleeding Disorders
Hemostasis is essential for managing patients with bleeding disorders, such as hemophilia, von Willebrand disease, and thrombocytopenia. Patients with these disorders are at increased risk of bleeding, even from minor injuries. Hemostatic treatments for bleeding disorders include replacement therapy with missing coagulation factors, desmopressin (DDAVP) to increase vWF and factor VIII levels, antifibrinolytic agents to prevent clot breakdown, and platelet transfusions to increase platelet count [15].
6.4 Interventional Cardiology
In interventional cardiology, hemostasis is essential for sealing arterial punctures after percutaneous procedures, such as angiography and angioplasty. Vascular closure devices are commonly used to seal arterial punctures and reduce the risk of bleeding complications. These devices include sutures, clips, and collagen plugs. The use of vascular closure devices has significantly reduced the time to hemostasis and ambulation after interventional cardiology procedures [16].
Many thanks to our sponsor Esdebe who helped us prepare this research report.
7. Future Directions and Research Areas
The field of hemostasis is continuously evolving, with ongoing research focused on developing novel hemostatic agents, improving diagnostic tools, and personalizing hemostasis management.
7.1 Novel Hemostatic Agents
Research is underway to develop new hemostatic agents with improved efficacy, biocompatibility, and ease of use. This includes research into biomimetic materials that mimic the natural coagulation process, as well as synthetic polymers that can promote rapid clot formation. The development of targeted hemostatic agents that can specifically bind to activated platelets or fibrin is also an area of active research.
7.2 Personalized Hemostasis Management
Personalized hemostasis management involves tailoring hemostatic treatments to the individual patient based on their specific coagulation profile, genetic background, and clinical characteristics. This approach requires the development of advanced diagnostic tools that can rapidly assess a patient’s coagulation status and predict their risk of bleeding or thrombosis. The use of AI and machine learning algorithms can help to analyze hemostasis data and optimize treatment strategies for individual patients.
7.3 Advanced Diagnostic Tools
Development of advanced diagnostic tools to assess coagulation status is crucial for improving hemostasis management. This includes the development of more sensitive and specific assays for measuring coagulation factors, platelet function, and fibrinolytic activity. Point-of-care (POC) hemostasis testing devices that can provide rapid results at the bedside are also an area of active development. These tools allow for more timely and informed decision-making regarding hemostatic interventions.
7.4 Gene Therapy for Bleeding Disorders
Gene therapy holds great promise for treating inherited bleeding disorders, such as hemophilia. Several gene therapy clinical trials have shown promising results in patients with hemophilia A and B, with sustained increases in factor VIII and factor IX levels [17]. Further research is needed to optimize gene therapy vectors and delivery methods and to ensure long-term safety and efficacy.
Many thanks to our sponsor Esdebe who helped us prepare this research report.
8. Conclusion
Hemostasis is a complex and essential physiological process that is critical for maintaining vascular integrity and preventing blood loss. Significant advancements have been made in hemostatic technologies, ranging from traditional surgical techniques to sophisticated pharmacological agents and innovative device-based solutions. The global hemostasis market is a substantial and growing segment of the medical device and pharmaceutical industries, driven by factors such as the increasing prevalence of surgical procedures, the rising incidence of trauma and bleeding disorders, and the growing demand for advanced hemostatic products. While hemostasis procedures are generally safe and effective, they are not without potential challenges and complications. Ongoing research is focused on developing novel hemostatic agents, improving diagnostic tools, and personalizing hemostasis management. Future research directions include the development of targeted hemostatic agents, the application of AI and machine learning to hemostasis management, and the use of gene therapy for treating inherited bleeding disorders. A continued, comprehensive understanding of the underlying mechanisms and advancements in hemostatic techniques will drive further innovation in this critical field.
Many thanks to our sponsor Esdebe who helped us prepare this research report.
References
[1] Furie, B., & Furie, B. C. (2008). Mechanisms of disease: mechanisms of thrombus formation. New England Journal of Medicine, 359(9), 938-949.
[2] Pries, A. R., Secomb, T. W., & Gaehtgens, P. (1996). The endothelial cell lining as a regulator of microvascular function. American Journal of Physiology-Heart and Circulatory Physiology, 270(3), H745-H756.
[3] Ruggeri, Z. M. (2002). Platelets in thrombus formation. Current Opinion in Hematology, 9(5), 365-372.
[4] Hoffman, M., & Monroe III, D. M. (2001). A cell-based model of hemostasis. Thrombosis and Haemostasis, 85(6), 958-965.
[5] Davie, E. W., Fujikawa, K., & Kisiel, W. (1991). The coagulation cascade: initiation, maintenance, and regulation. Biochemistry, 30(43), 10363-10370.
[6] Collen, D. (1980). On the regulation and control of fibrinolysis. Thrombosis and Haemostasis, 43(2), 77-89.
[7] Farouk, S., Nathwani, S., Remzi, F. H., & Strong, S. A. (2015). Electrocautery in surgery. Surgical Technology International, 26, 19-28.
[8] Amaral, J. F. (1994). The experimental development of an argon beam coagulator. Surgical Clinics of North America, 74(6), 1277-1291.
[9] Franchini, M., Lippi, G., & Franchini, G. (2007). Recombinant activated factor VII: a critical review on safety. Blood, 110(8), 2717-2725.
[10] Spotnitz, W. D. (2010). Fibrin sealant and related hemostatic and adhesive products: mechanism of action, efficacy, and safety. Transfusion, 50(12), 2681-2698.
[11] McManus, J. G., O’Brien, J. G., & Wade, C. E. (2007). Tourniquet use in the prehospital environment. Journal of Trauma-Injury Infection and Critical Care, 63(1), S20-S23.
[12] Global Market Insights. (2024). Hemostasis Market Analysis Report By Product (Topical, Infusion, Advanced Hemostats), By Application, By End-use, Regional Outlook, Application Potential, Competitive Market Share & Forecast, 2023 – 2032. Retrieved from https://www.gminsights.com/industry-analysis/hemostasis-market
[13] George, L. A. (2021). Gene therapy for hemophilia. Haemophilia, 27 Suppl 6, 91-96.
[14] Eastridge, B. J., Mabry, R. L., Seguin, P., Cantrell, J., Tops, G., Uribe, P., … & Shackelford, S. (2012). Death on the battlefield (2001–2011): implications for the future of combat casualty care. The Journal of Trauma and Acute Care Surgery, 73(6), S431-S437.
[15] Berntorp, E., Shapiro, A. D., Key, N. S., & Castaman, G. (2015). Modern haemophilia care. The Lancet, 385(9982), 2173-2182.
[16] Koreny, M., Riedmann, M. J., Ulrich, S., Siostrzonek, P., & Müllner, M. (2004). Arterial puncture closure devices compared with manual compression after cardiac catheterization: a meta-analysis. American Heart Journal, 147(1), 16-23.
[17] Pipe, S. W., Leebeek, F. W. G., Recht, M., Key, N. S., Knobe, K., Baynes, P., … & Lillicrap, D. (2024). Valoctocogene Roxaparvovec Gene Therapy in Adults with Severe Hemophilia A. New England Journal of Medicine, 390, 297-307.
Be the first to comment