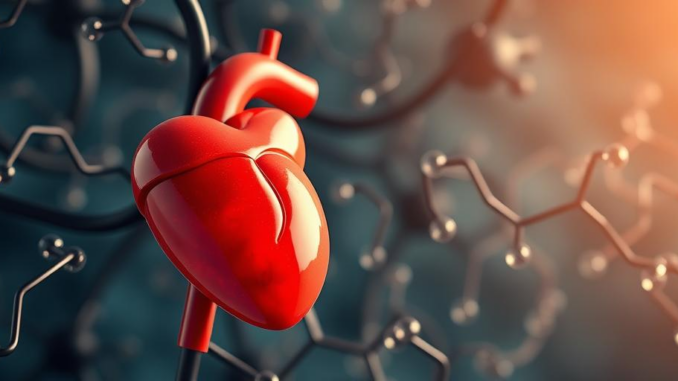
Abstract
Triglycerides (TGs) are the primary form of fat storage in the body and play a crucial role in energy homeostasis. While essential for normal physiological function, elevated TG levels, or hypertriglyceridemia (HTG), are a significant risk factor for cardiovascular disease (CVD), pancreatitis, and other metabolic disorders. This research report provides a comprehensive overview of TG metabolism, exploring the complex interplay of enzymes, apolipoproteins, and cellular processes involved in TG synthesis, transport, and breakdown. We delve into the pathophysiology of HTG, examining the genetic and environmental factors that contribute to its development, with particular emphasis on the extreme form seen in Familial Chylomicronemia Syndrome (FCS). Furthermore, we critically evaluate current and emerging therapeutic strategies for managing TG levels, encompassing dietary interventions, lifestyle modifications, and pharmacological approaches. The report also highlights the intricate relationship between TGs and other metabolic disorders, such as insulin resistance, obesity, and non-alcoholic fatty liver disease (NAFLD). Finally, we identify key areas for future research, focusing on personalized medicine approaches and the development of novel therapies that target specific pathways in TG metabolism.
Many thanks to our sponsor Esdebe who helped us prepare this research report.
1. Introduction
Triglycerides (TGs), composed of a glycerol backbone esterified with three fatty acids, represent the predominant form of dietary and stored fat. Their primary function is to provide a readily available energy source for cells and tissues. TGs are synthesized in the liver and intestine and are transported throughout the body via lipoproteins, primarily chylomicrons (CMs) and very-low-density lipoproteins (VLDL). The regulation of TG metabolism is a complex process involving numerous enzymes, apolipoproteins, and hormonal signals. Disruptions in this tightly controlled system can lead to hypertriglyceridemia (HTG), a common metabolic disorder characterized by elevated TG levels in the blood. HTG is a well-established risk factor for CVD, acute pancreatitis, and other metabolic complications. The severity of HTG varies, ranging from mild to severe, and can be caused by a combination of genetic predisposition and environmental factors, including diet, lifestyle, and underlying medical conditions. One extreme manifestation of HTG is Familial Chylomicronemia Syndrome (FCS), a rare genetic disorder characterized by extremely high TG levels and a high risk of recurrent pancreatitis. Understanding the intricacies of TG metabolism and the factors that contribute to HTG is crucial for developing effective strategies for prevention and treatment. This report aims to provide a comprehensive overview of TG metabolism, its dysregulation in HTG, and current and emerging therapeutic approaches.
Many thanks to our sponsor Esdebe who helped us prepare this research report.
2. Triglyceride Metabolism
2.1. TG Synthesis
TG synthesis occurs primarily in the endoplasmic reticulum (ER) of hepatocytes, enterocytes, and adipocytes. The process begins with the formation of glycerol-3-phosphate, either from glucose via glycolysis or from glycerol via glycerol kinase (primarily in the liver). Fatty acids are then activated by coenzyme A (CoA) to form fatty acyl-CoA. Subsequently, acyltransferases catalyze the sequential esterification of fatty acyl-CoA molecules to glycerol-3-phosphate, resulting in the formation of monoacylglycerol (MAG), diacylglycerol (DAG), and finally, TG. Key enzymes involved in TG synthesis include glycerol-3-phosphate acyltransferase (GPAT), acylglycerol-phosphate acyltransferase (AGPAT), and diacylglycerol acyltransferase (DGAT). Two isoforms of DGAT, DGAT1 and DGAT2, have been identified, each playing a distinct role in TG synthesis. DGAT1 is ubiquitously expressed, while DGAT2 is predominantly expressed in the liver and adipose tissue. Variations in the expression and activity of these enzymes can significantly impact TG synthesis rates and contribute to the development of HTG.
2.2. Lipoprotein Assembly and Secretion
TGs are hydrophobic molecules and cannot be directly transported in the aqueous environment of the blood. Therefore, they are packaged into lipoprotein particles, which are spherical structures composed of a core of TGs and cholesterol esters surrounded by a phospholipid monolayer and apolipoproteins. The major TG-rich lipoproteins are chylomicrons (CMs) and very-low-density lipoproteins (VLDL). CMs are synthesized in the enterocytes of the small intestine following the absorption of dietary fat. They transport TGs from the intestine to peripheral tissues, such as adipose tissue and muscle. VLDL are synthesized in the liver and transport TGs from the liver to peripheral tissues. The assembly of CMs and VLDL is a complex process involving several steps, including the incorporation of apolipoproteins, such as apoB-48 (for CMs) and apoB-100 (for VLDL), and the transfer of TGs into the lipoprotein core. Microsomal triglyceride transfer protein (MTP) plays a critical role in this process by facilitating the transfer of TGs and other lipids to apoB within the ER lumen. Deficiencies in MTP lead to abetalipoproteinemia, a rare genetic disorder characterized by the inability to synthesize CMs and VLDL.
2.3. Lipoprotein Lipase and TG Hydrolysis
Lipoprotein lipase (LPL) is a key enzyme responsible for hydrolyzing TGs in CMs and VLDL, releasing fatty acids that can be taken up by cells for energy production or storage. LPL is synthesized primarily in adipose tissue and muscle and is translocated to the luminal surface of capillaries, where it binds to heparan sulfate proteoglycans. Apolipoprotein C-II (apoC-II) acts as a cofactor for LPL, enhancing its activity. Upon binding to LPL, CMs and VLDL are hydrolyzed, releasing fatty acids and glycerol. The fatty acids are then taken up by cells via fatty acid transport proteins (FATPs) and either oxidized for energy or re-esterified to form TGs for storage. Following TG hydrolysis, CMs are converted to CM remnants, and VLDL are converted to intermediate-density lipoproteins (IDL). CM remnants are taken up by the liver via receptor-mediated endocytosis, while IDL can be either taken up by the liver or further converted to low-density lipoproteins (LDL).
2.4. Regulation of TG Metabolism
The regulation of TG metabolism is a complex process involving hormonal signals, transcriptional factors, and post-translational modifications. Insulin plays a central role in regulating TG metabolism by stimulating TG synthesis in the liver and adipose tissue and by inhibiting lipolysis in adipose tissue. Insulin also increases the expression and activity of LPL in adipose tissue, promoting TG uptake. Glucagon, on the other hand, promotes lipolysis in adipose tissue, releasing fatty acids into the circulation. Peroxisome proliferator-activated receptors (PPARs) are a family of nuclear receptors that play a crucial role in regulating lipid metabolism. PPARα is primarily expressed in the liver and regulates the expression of genes involved in fatty acid oxidation and lipoprotein metabolism. PPARγ is primarily expressed in adipose tissue and regulates the expression of genes involved in adipogenesis and insulin sensitivity. Sterol regulatory element-binding proteins (SREBPs) are transcription factors that regulate the expression of genes involved in lipid synthesis. SREBP-1c is the primary isoform involved in regulating TG synthesis in the liver. These regulatory mechanisms ensure that TG levels are maintained within a narrow physiological range, adapting to changes in energy intake and expenditure.
Many thanks to our sponsor Esdebe who helped us prepare this research report.
3. Pathophysiology of Hypertriglyceridemia
3.1. Genetic Factors
Genetic factors play a significant role in determining an individual’s susceptibility to HTG. Several genes have been identified that are associated with elevated TG levels, including genes encoding LPL, apoC-II, apoA-V, GPIHBP1, and LMF1. Mutations in these genes can lead to decreased LPL activity or impaired lipoprotein metabolism, resulting in HTG. Familial Chylomicronemia Syndrome (FCS) is a rare genetic disorder characterized by extremely high TG levels and recurrent pancreatitis. FCS is typically caused by homozygous or compound heterozygous mutations in one of the genes involved in LPL pathway, most commonly in LPL itself. These mutations result in a severe deficiency of LPL activity, leading to a dramatic accumulation of CMs in the blood. Other genetic variants, such as single nucleotide polymorphisms (SNPs), can also contribute to HTG, albeit to a lesser extent. Genome-wide association studies (GWAS) have identified numerous SNPs that are associated with TG levels, providing further insights into the genetic architecture of HTG.
3.2. Environmental Factors
Environmental factors, such as diet, lifestyle, and underlying medical conditions, also play a crucial role in the development of HTG. A diet high in saturated fat, trans fat, and simple carbohydrates can increase TG levels by promoting TG synthesis in the liver and by reducing LPL activity. Excessive alcohol consumption can also increase TG levels by inhibiting fatty acid oxidation and by stimulating TG synthesis. Sedentary lifestyle and obesity are also associated with HTG, as they can lead to insulin resistance and impaired lipoprotein metabolism. Certain medical conditions, such as diabetes, hypothyroidism, and kidney disease, can also contribute to HTG. Medications, such as beta-blockers, thiazide diuretics, and estrogen, can also increase TG levels. The interaction between genetic and environmental factors determines an individual’s overall risk of developing HTG. Individuals with a genetic predisposition to HTG may be more susceptible to the effects of environmental factors, and vice versa.
3.3. Mechanisms of TG-Induced Harm
While TGs are essential for energy storage, elevated TG levels can be detrimental to health. HTG is a well-established risk factor for CVD, pancreatitis, and other metabolic complications. The mechanisms by which TGs contribute to these conditions are complex and multifactorial. In the context of CVD, HTG is associated with increased levels of small, dense LDL particles, which are more atherogenic than larger, buoyant LDL particles. TGs can also promote inflammation and endothelial dysfunction, contributing to the development of atherosclerosis. In the context of pancreatitis, elevated TG levels can lead to the formation of free fatty acids in the pancreas, which can damage pancreatic cells and trigger an inflammatory cascade. The exact threshold at which TGs cause pancreatitis is variable and depends on individual susceptibility. Other metabolic complications associated with HTG include non-alcoholic fatty liver disease (NAFLD), insulin resistance, and increased risk of type 2 diabetes. The mechanisms by which TGs contribute to NAFLD are not fully understood, but they likely involve increased hepatic TG synthesis and impaired TG export. TGs can also impair insulin signaling, leading to insulin resistance and increased risk of type 2 diabetes.
Many thanks to our sponsor Esdebe who helped us prepare this research report.
4. Therapeutic Strategies for Managing Triglyceride Levels
4.1. Dietary Interventions
Dietary interventions are a cornerstone of TG management. Reducing the intake of saturated fat, trans fat, and simple carbohydrates can significantly lower TG levels. Replacing saturated fat with unsaturated fat, particularly polyunsaturated fatty acids (PUFAs), can also be beneficial. Omega-3 fatty acids, such as eicosapentaenoic acid (EPA) and docosahexaenoic acid (DHA), have been shown to lower TG levels by inhibiting TG synthesis in the liver and by increasing LPL activity. Increasing fiber intake can also help lower TG levels by reducing the absorption of dietary fat and cholesterol. Limiting alcohol consumption is also important, as alcohol can significantly increase TG levels. A heart-healthy diet, such as the Mediterranean diet, which is rich in fruits, vegetables, whole grains, and healthy fats, can be an effective strategy for managing TG levels.
4.2. Lifestyle Modifications
Lifestyle modifications, such as regular physical activity and weight loss, are also important for managing TG levels. Regular exercise can increase LPL activity and promote fatty acid oxidation, leading to lower TG levels. Weight loss, even modest weight loss, can significantly reduce TG levels by reducing TG synthesis in the liver and adipose tissue. Smoking cessation is also important, as smoking can increase TG levels and impair lipoprotein metabolism. A comprehensive lifestyle modification program that includes dietary changes, exercise, and smoking cessation can be highly effective in managing TG levels.
4.3. Pharmacological Approaches
Pharmacological approaches are often necessary for individuals with severe HTG or those who do not respond adequately to dietary and lifestyle interventions. Several classes of drugs are available for lowering TG levels, including fibrates, omega-3 fatty acids, niacin, and statins. Fibrates are PPARα agonists that increase LPL activity and reduce TG synthesis in the liver. They are highly effective in lowering TG levels and are often used as first-line therapy for severe HTG. Omega-3 fatty acids, particularly high-dose EPA and DHA, can lower TG levels by inhibiting TG synthesis in the liver and by increasing LPL activity. Niacin (nicotinic acid) lowers TG levels by inhibiting lipolysis in adipose tissue and by reducing VLDL secretion from the liver. Statins are primarily used to lower LDL cholesterol, but they can also modestly lower TG levels by inhibiting cholesterol synthesis in the liver. Volanesorsen is an antisense oligonucleotide that inhibits the production of apoC-III, a protein that inhibits LPL activity. Volanesorsen has been approved for the treatment of FCS and is highly effective in lowering TG levels in these patients. Pemafibrate is a selective PPARα modulator (SPPARM) with a distinct pharmacological profile compared to conventional fibrates. It has shown promise in lowering TG levels and improving other lipid parameters, while potentially exhibiting a better safety profile than traditional fibrates. The choice of medication depends on the severity of HTG, the presence of other risk factors, and the individual’s response to treatment.
Many thanks to our sponsor Esdebe who helped us prepare this research report.
5. Triglycerides and Other Metabolic Disorders
5.1. Insulin Resistance and Type 2 Diabetes
HTG is closely linked to insulin resistance and type 2 diabetes. Elevated TG levels can impair insulin signaling, leading to insulin resistance. Insulin resistance, in turn, can exacerbate HTG by increasing TG synthesis in the liver and by reducing LPL activity. This creates a vicious cycle that promotes both HTG and insulin resistance. Individuals with type 2 diabetes often have elevated TG levels, and HTG is a major risk factor for CVD in these patients. Managing TG levels is an important component of diabetes management, as it can help reduce the risk of CVD and other complications.
5.2. Obesity and NAFLD
Obesity and non-alcoholic fatty liver disease (NAFLD) are also closely associated with HTG. Obesity is characterized by an excess of adipose tissue, which can lead to increased TG synthesis and release into the circulation. NAFLD is characterized by the accumulation of TGs in the liver, which can lead to inflammation, liver damage, and eventually cirrhosis. HTG is a major risk factor for NAFLD, and the severity of NAFLD is often correlated with TG levels. Managing TG levels is an important component of managing obesity and NAFLD, as it can help reduce the risk of liver damage and other complications.
5.3. Metabolic Syndrome
HTG is a key component of the metabolic syndrome, a cluster of metabolic abnormalities that increase the risk of CVD, type 2 diabetes, and other chronic diseases. The metabolic syndrome is typically defined as the presence of three or more of the following criteria: abdominal obesity, elevated TG levels, low HDL cholesterol levels, elevated blood pressure, and elevated fasting glucose levels. Individuals with the metabolic syndrome are at increased risk of developing HTG, and vice versa. Managing TG levels is an important component of managing the metabolic syndrome, as it can help reduce the risk of CVD and other complications.
Many thanks to our sponsor Esdebe who helped us prepare this research report.
6. Future Directions
Future research should focus on several key areas to improve the management of HTG and reduce the risk of associated complications. Personalized medicine approaches that take into account an individual’s genetic background, lifestyle, and other risk factors are needed to tailor treatment strategies to each patient. Further research is needed to identify novel therapeutic targets for lowering TG levels, particularly targets that address the underlying causes of HTG. The development of new drugs that target specific pathways in TG metabolism, such as TG synthesis or LPL activity, is a promising area of research. Clinical trials are needed to evaluate the efficacy and safety of these new drugs. Further research is also needed to understand the complex interplay between TGs and other metabolic disorders, such as insulin resistance, obesity, and NAFLD. Understanding these interactions is crucial for developing effective strategies for preventing and treating these conditions. Finally, more research is needed to evaluate the long-term effects of different TG-lowering strategies on CVD risk and other health outcomes.
Many thanks to our sponsor Esdebe who helped us prepare this research report.
7. Conclusion
Triglycerides are essential for energy storage, but elevated TG levels can be detrimental to health. HTG is a common metabolic disorder that is associated with an increased risk of CVD, pancreatitis, and other metabolic complications. The regulation of TG metabolism is a complex process involving numerous enzymes, apolipoproteins, and hormonal signals. Disruptions in this tightly controlled system can lead to HTG. Genetic and environmental factors play a crucial role in the development of HTG. Therapeutic strategies for managing TG levels include dietary interventions, lifestyle modifications, and pharmacological approaches. Future research should focus on personalized medicine approaches and the development of novel therapies that target specific pathways in TG metabolism. A deeper understanding of TG metabolism and its dysregulation in HTG is crucial for developing effective strategies for prevention and treatment, ultimately improving the health and well-being of individuals with this common metabolic disorder.
Many thanks to our sponsor Esdebe who helped us prepare this research report.
References
- Goldberg, I. J. (1996). Lipoprotein lipase and lipoprotein metabolism. Current Opinion in Lipidology, 7(3), 125-134.
- Miller, M., Stone, N. J., Ballantyne, C. M., Bittner, V., Criqui, M. H., Ginsberg, H. N., … & American Heart Association Clinical Lipidology, Thrombosis, and Prevention Committee of the Council on Nutrition, Physical Activity, and Metabolism, Council on Clinical Cardiology, and Stroke Council. (2011). Triglycerides and cardiovascular disease: a scientific statement from the American Heart Association. Circulation, 123(20), 2292-2333.
- Brunzell, J. D., & Deeb, S. S. (2001). Familial lipoprotein lipase deficiency. GeneReviews® [Internet].
- Fruchart, J. C., Davignon, J., Hermans, M. P., de Graaf, J., Pettersson, J., Dallinga-Thie, G. M., … & European Atherosclerosis Society Consensus Panel. (2014). Residual cardiovascular risk in high-risk dyslipidaemic patients on statin treatment: a joint position paper from the International Atherosclerosis Society and the European Atherosclerosis Society. Atherosclerosis, 233(1), 1-13.
- Toth, P. P., Granowitz, C., Hull, M., & Gouni-Berthold, I. (2022). Pemafibrate: A Selective PPARα Modulator for Management of Hypertriglyceridemia and Residual Cardiovascular Risk. The American Journal of Cardiology, 167, 74-85.
- Davidson, M. H., Bays, H. E., Maki, K. C., Gစ္ouni-Berthold, I., Rizk, E., Toth, P. P., … & PROMINENT Investigators. (2023). Effects of Pemafibrate on Cardiovascular Outcomes in Patients With Type 2 Diabetes and Hypertriglyceridemia. Journal of the American College of Cardiology, 81(1), 1-13.
- Hegele, R. A., Ginsberg, H. N., Serfaty-Lacrosniere, C., Frohlich, J., Hֵerve, F., & Cases-Cordero, Y. (2014). The polygenic nature of hypertriglyceridaemia: implications for diagnosis and therapy. The Lancet Diabetes & Endocrinology, 2(8), 655-666.
- Taskinen, M. R., Boren, J., Yadav, D., & Abela, G. S. (2019). Triglycerides and cardiovascular disease: How do high serum triglycerides increase cardiovascular risk?. Atherosclerosis, 290, 124-131.
- Jensen, R. G. (2002). Lipases. In Advanced Dairy Chemistry—1 Lipids (pp. 229-304). Springer, Boston, MA.
- Reiner, Ž., De Backer, G., Fras, Z., Gasowski, J., Graaf, J. D., Graham, I., … & Wood, D. A. (2011). Clinical practice guidelines on the management of lipid disorders. European heart journal, 32(14), 1731-1776.
- Stefan, N., Haring, H. U., & Cusi, K. (2021). Non-alcoholic fatty liver disease: causes, metabolic consequences, and treatment strategies. The Lancet Diabetes & Endocrinology, 9(9), 664-676.
Be the first to comment