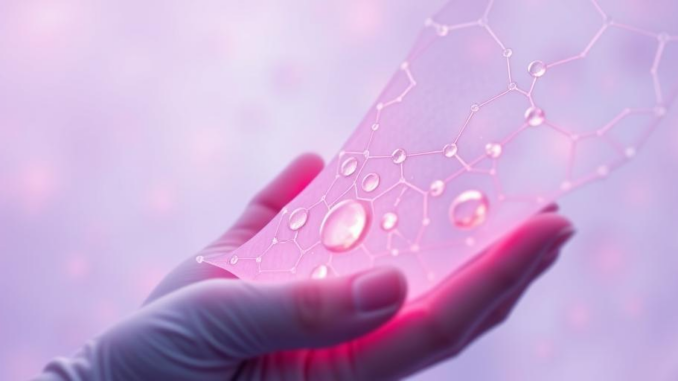
Abstract
Ablation, the targeted destruction of tissue to achieve a specific therapeutic outcome, has emerged as a cornerstone treatment modality across diverse medical specialties. While often associated with cardiac electrophysiology, its applications extend far beyond, encompassing oncology, neurology, ophthalmology, and cosmetic surgery. This review provides a comprehensive overview of ablation technologies, encompassing radiofrequency ablation (RFA), cryoablation, microwave ablation (MWA), high-intensity focused ultrasound (HIFU), pulsed field ablation (PFA), laser ablation, and chemical ablation. We delve into the underlying mechanisms of action, efficacy rates, potential complications, and patient selection criteria for each modality. Further, we explore advancements in ablation technology, including image guidance, robotic assistance, and personalized ablation strategies, and discuss future directions in the field, focusing on minimizing collateral damage, improving long-term outcomes, and expanding the scope of ablation therapies.
Many thanks to our sponsor Esdebe who helped us prepare this research report.
1. Introduction
Ablation, derived from the Latin word ablatio meaning ‘removal’, is a medical procedure used to remove or destroy abnormal tissue. The technique has rapidly evolved from its initial applications in cardiac electrophysiology to a versatile tool utilized across numerous medical disciplines. The core principle involves the delivery of energy, either thermal (heat or cold), electrical, or chemical, to induce cellular necrosis or apoptosis within the targeted tissue volume. The success of ablation relies on precise targeting, effective energy delivery, and minimization of damage to surrounding healthy tissues. This review aims to provide a detailed exploration of different ablation modalities, their mechanisms of action, clinical applications, associated challenges, and future prospects.
Many thanks to our sponsor Esdebe who helped us prepare this research report.
2. Ablation Modalities: Mechanisms and Characteristics
2.1 Radiofrequency Ablation (RFA)
RFA is one of the most widely used ablation techniques, particularly in cardiac and oncological applications. It utilizes alternating current in the radiofrequency range (typically 350-500 kHz) to generate heat within the target tissue. The heat causes coagulative necrosis, resulting in cell death. RFA can be delivered via unipolar or bipolar electrodes. Unipolar RFA requires a grounding pad to complete the circuit, while bipolar RFA has both electrodes located within the ablation probe, offering more localized energy delivery.
Mechanism of Action: The high-frequency current induces ionic agitation within the tissue, leading to frictional heating. As the temperature rises above 60°C, protein denaturation and cellular destruction occur. Above 100°C, tissue vaporization can occur, potentially leading to charring and impedance rise, which can limit the effectiveness of the ablation.
Clinical Applications: RFA is extensively used for the treatment of cardiac arrhythmias, liver tumors, kidney tumors, lung tumors, and bone metastases. It is also employed in pain management for facet joint denervation and nerve blocks.
Advantages: Well-established technique with extensive clinical experience, relatively inexpensive compared to other modalities, and can achieve large ablation volumes with multiple electrode deployments.
Disadvantages: Risk of thermal damage to surrounding structures, potential for charring and impedance rise, and limitations in ablating tissues adjacent to large blood vessels due to the heat sink effect.
2.2 Cryoablation
Cryoablation employs extreme cold to induce cell death. It utilizes cryoprobes that circulate cryogenic agents, such as liquid nitrogen or argon gas, to rapidly cool the targeted tissue to temperatures as low as -40°C to -80°C. This extreme cold causes intracellular ice crystal formation, leading to cell rupture and necrosis.
Mechanism of Action: Cryoablation induces cell death through multiple mechanisms: intracellular ice crystal formation, cellular dehydration, vascular stasis and thrombosis, and denaturation of cellular proteins and enzymes. The formation of an ice ball allows for visualization and monitoring of the ablation zone.
Clinical Applications: Cryoablation is used in the treatment of prostate cancer, kidney tumors, lung tumors, breast fibroadenomas, and cardiac arrhythmias. It is also used in pain management for nerve blocks.
Advantages: Preservation of collagen matrix, better visualization of the ablation zone through ice ball formation, less pain compared to RFA, and lower risk of damage to surrounding structures due to the cryoprotective effect of cold.
Disadvantages: Slower ablation times compared to RFA, potential for nerve damage, and risk of cryoshock if large volumes of tissue are ablated rapidly.
2.3 Microwave Ablation (MWA)
MWA utilizes electromagnetic waves in the microwave frequency range (typically 915 MHz or 2.45 GHz) to generate heat within the target tissue. MWA offers faster ablation times and the ability to achieve higher temperatures compared to RFA.
Mechanism of Action: Microwave energy causes rapid oscillation of water molecules within the tissue, leading to frictional heating and coagulative necrosis. MWA is less susceptible to the heat sink effect compared to RFA due to its ability to generate higher temperatures and larger ablation volumes.
Clinical Applications: MWA is used in the treatment of liver tumors, lung tumors, kidney tumors, and bone metastases. It is also being explored for the treatment of thyroid nodules and soft tissue tumors.
Advantages: Faster ablation times compared to RFA, less susceptible to the heat sink effect, and can achieve larger ablation volumes with a single probe insertion.
Disadvantages: Risk of thermal damage to surrounding structures, potential for charring and impedance rise, and higher cost compared to RFA.
2.4 High-Intensity Focused Ultrasound (HIFU)
HIFU utilizes focused ultrasound waves to generate heat within a targeted tissue volume without damaging intervening tissues. The ultrasound waves are focused to a specific point, where the energy is converted into heat, leading to coagulative necrosis.
Mechanism of Action: The focused ultrasound waves induce rapid temperature elevation at the focal point, resulting in protein denaturation and cell death. The surrounding tissues remain relatively unaffected due to the focused nature of the energy delivery.
Clinical Applications: HIFU is used in the treatment of prostate cancer, uterine fibroids, kidney tumors, and bone metastases. It is also being explored for the treatment of liver tumors and breast cancer.
Advantages: Non-invasive, precise targeting, and minimal damage to surrounding tissues.
Disadvantages: Limited penetration depth, potential for skin burns, and difficulty in treating tissues adjacent to bone or air-filled structures.
2.5 Pulsed Field Ablation (PFA)
PFA is a relatively novel ablation technique that utilizes short, high-voltage electrical pulses to induce cell death. Unlike thermal ablation techniques, PFA is considered non-thermal and is believed to selectively target cardiomyocytes while sparing other tissues, such as the esophagus and nerves.
Mechanism of Action: PFA induces cell death through electroporation, which involves the formation of pores in the cell membrane due to the application of high-voltage electrical pulses. This disrupts cellular homeostasis and leads to cell death. The selectivity of PFA for cardiomyocytes is attributed to their unique electrical properties and larger size compared to other cells.
Clinical Applications: PFA is primarily being developed for the treatment of atrial fibrillation. Early clinical trials have shown promising results in terms of efficacy and safety.
Advantages: Potential for selective ablation of cardiomyocytes, reduced risk of thermal damage to surrounding structures, and faster ablation times compared to thermal ablation techniques.
Disadvantages: Relatively new technology with limited long-term clinical data, potential for muscle stimulation, and risk of esophageal injury if not performed correctly.
2.6 Laser Ablation
Laser ablation utilizes focused laser beams to generate heat and induce tissue destruction. Different types of lasers, such as Nd:YAG, argon, and CO2 lasers, can be used depending on the specific application. The wavelength of the laser determines its absorption characteristics in different tissues.
Mechanism of Action: Laser energy is absorbed by the tissue, leading to rapid temperature elevation and coagulative necrosis. The depth of penetration and the extent of tissue damage depend on the laser wavelength, power, and pulse duration.
Clinical Applications: Laser ablation is used in dermatology for the treatment of skin lesions, in ophthalmology for the treatment of retinal diseases, and in surgery for the removal of tumors and vascular malformations.
Advantages: Precise targeting, minimal damage to surrounding tissues, and can be used in open, laparoscopic, or percutaneous procedures.
Disadvantages: Risk of thermal damage to surrounding structures, potential for scarring, and higher cost compared to other ablation techniques.
2.7 Chemical Ablation
Chemical ablation involves the injection of a cytotoxic agent directly into the target tissue to induce cell death. Common chemical ablation agents include ethanol, acetic acid, and bleomycin.
Mechanism of Action: The cytotoxic agent disrupts cellular metabolism and causes cell necrosis. The mechanism of action varies depending on the specific agent used. Ethanol causes cellular dehydration and protein denaturation, while acetic acid causes cellular acidification and protein coagulation. Bleomycin inhibits DNA synthesis and induces cell apoptosis.
Clinical Applications: Chemical ablation is used in the treatment of liver tumors, thyroid nodules, and arteriovenous malformations. It is also used in cosmetic surgery for the treatment of wrinkles and skin lesions.
Advantages: Relatively inexpensive, can be used in areas that are difficult to access with other ablation techniques, and can be performed percutaneously.
Disadvantages: Risk of systemic toxicity, potential for local complications such as pain, inflammation, and infection, and less precise targeting compared to other ablation techniques.
Many thanks to our sponsor Esdebe who helped us prepare this research report.
3. Advancements in Ablation Technology
The field of ablation technology is constantly evolving, with ongoing advancements aimed at improving efficacy, safety, and precision. Some of the key advancements include:
3.1 Image Guidance
Image guidance plays a crucial role in ensuring accurate targeting and monitoring of ablation procedures. Various imaging modalities, such as ultrasound, CT, MRI, and PET/CT, can be used to guide ablation probes and assess the extent of tissue destruction. Real-time imaging allows for adjustments during the procedure to optimize ablation outcomes.
3.2 Robotic Assistance
Robotic systems are increasingly being used to assist in ablation procedures. These systems offer enhanced precision, stability, and dexterity, allowing for more complex and challenging ablations to be performed with greater accuracy. Robotic assistance can also reduce operator fatigue and improve workflow efficiency.
3.3 Personalized Ablation Strategies
Personalized ablation strategies involve tailoring the ablation technique and parameters to the individual patient’s anatomy, physiology, and disease characteristics. This approach aims to optimize ablation outcomes while minimizing the risk of complications. Factors such as tumor size, location, and proximity to critical structures are considered when developing a personalized ablation plan.
3.4 Integrated Systems
Integrated ablation systems combine multiple technologies, such as imaging, navigation, and energy delivery, into a single platform. These systems streamline the ablation workflow and provide comprehensive information to the operator, enabling more efficient and effective ablation procedures.
Many thanks to our sponsor Esdebe who helped us prepare this research report.
4. Challenges and Future Directions
Despite the significant advancements in ablation technology, several challenges remain. These include:
- Minimizing Collateral Damage: Ensuring that only the target tissue is ablated while sparing surrounding healthy tissues remains a key challenge. Improving targeting precision, developing more selective ablation techniques, and utilizing tissue-sparing energy delivery methods are crucial for minimizing collateral damage.
- Improving Long-Term Outcomes: While ablation can effectively destroy targeted tissue, recurrence of disease remains a concern. Developing strategies to enhance the durability of ablation outcomes, such as combining ablation with adjuvant therapies, is essential for improving long-term outcomes.
- Expanding the Scope of Ablation Therapies: Exploring the potential of ablation for treating a wider range of diseases and conditions is an ongoing area of research. This includes investigating the use of ablation for treating neurological disorders, autoimmune diseases, and infectious diseases.
- Real-time Monitoring and Feedback: Further development of real-time monitoring technologies that provide feedback on the effectiveness of the ablation and potential damage to surrounding tissues. This would allow for immediate adjustments during the procedure, improving safety and efficacy.
- Integration of Artificial Intelligence (AI): Implementing AI algorithms for pre-operative planning and intra-operative decision-making. AI can assist in identifying optimal ablation parameters, predicting treatment outcomes, and adapting to unforeseen circumstances during the procedure.
The future of ablation technology is bright, with ongoing research and development efforts focused on addressing these challenges and expanding the scope of ablation therapies. By combining technological innovation with a personalized approach, ablation has the potential to become an even more powerful and versatile tool in the fight against disease.
Many thanks to our sponsor Esdebe who helped us prepare this research report.
5. Conclusion
Ablation technologies have revolutionized the treatment landscape across multiple medical specialties. From the established techniques of RFA and cryoablation to the emerging modalities of PFA and HIFU, ablation offers a minimally invasive approach to target and destroy diseased tissue. Advancements in image guidance, robotic assistance, and personalized strategies are further enhancing the precision and efficacy of ablation procedures. As the field continues to evolve, future research will focus on minimizing collateral damage, improving long-term outcomes, and expanding the scope of ablation therapies. The integration of AI and real-time monitoring will undoubtedly play a crucial role in shaping the future of ablation, leading to more precise, personalized, and effective treatments for a wide range of conditions. Ultimately, the ongoing development and refinement of ablation technologies hold immense promise for improving patient outcomes and quality of life.
Many thanks to our sponsor Esdebe who helped us prepare this research report.
References
- Nakagawa, H., & Stevenson, W. G. (2023). Pulsed field ablation for atrial fibrillation. Heart Rhythm, 20(12), 1767-1768.
- Ahmed, M., Brace, C. L., Curry, M. P., Dillon, M. B., Goldberg, S. N., Hahn, P. F., … & Rhim, H. (2011). Radiofrequency ablation: review of basic principles. Journal of Vascular and Interventional Radiology, 22(3), 259-269.
- Hinshaw, J. L., Lee, F. T., & Littrup, P. J. (2009). Cryoablation: theory and clinical applications. American Journal of Roentgenology, 193(6), 191-201.
- Simon, C. J., Dupuy, D. E., & Mayo-Smith, W. W. (2005). Microwave ablation: principles and applications. RadioGraphics, 25(suppl_1), S69-S83.
- Kennedy, J. E., Ter Haar, G. R., Cranston, D., Staruch, R., Gleeson, F., Shaw, G., & Field, S. B. (2000). High-intensity focused ultrasound: surgery of the future?. British Journal of Radiology, 73(875), 590-599.
- Neven, K., & Knecht, S. (2023). Pulsed Field Ablation (PFA) in Atrial Fibrillation: A Novel Tool with High Potential. Cardiovascular Innovation and Application, 8(1), 3-13.
- Anderson, R. R., & Parrish, J. A. (1983). Selective photothermolysis: precise microsurgery by selective absorption of pulsed radiation. Science, 220(4596), 524-527.
- Livraghi, T., Benedetti Rossi, P., Lazzaroni, S., Meloni, F., Gazzelle, G., & Veltri, A. (1995). Percutaneous ethanol injection in the treatment of hepatocellular carcinoma. Cancer, 76(3), 346-352.
- Woodrum, D. A., Khoshknabi, A., & McGreevy, K. S. (2016). Image-guided ablation: technology and techniques. Seminars in Interventional Radiology, 33(03), 187-198.
- Patel, M. R., White, C. J., Mahmud, E., Spertus, J. A., Cohen, D. J., Teirstein, P. S., … & Messenger, J. C. (2008). Robotic-assisted percutaneous coronary intervention. JACC: Cardiovascular Interventions, 1(6), 691-699.
Given the advancements in image guidance, could the integration of AI algorithms for real-time monitoring and adaptive adjustment of ablation parameters further minimize collateral damage and improve long-term outcomes?
That’s a great point! The potential for AI to analyze real-time imaging data and dynamically adjust ablation parameters is huge. This adaptive approach could lead to far more precise treatments, minimizing damage to surrounding healthy tissue and leading to better patient outcomes. Thanks for highlighting this important direction!
Editor: MedTechNews.Uk
Thank you to our Sponsor Esdebe
So, ablation’s moving beyond cardiac? Next, you’ll tell me my dermatologist’s laser is basically the same tech my cardiologist uses. Wonder if they offer frequent flyer miles for tissue removal?