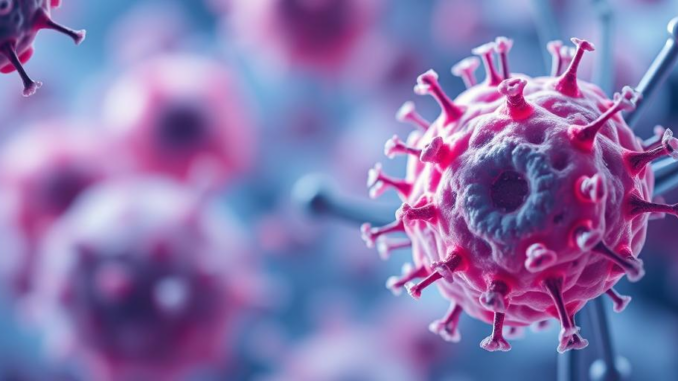
Abstract
Immunosuppression is the cornerstone of preventing allograft rejection in solid organ and cell transplantation. However, the current reliance on broad-spectrum immunosuppressive agents is associated with significant morbidity, including increased susceptibility to infection, malignancy, and metabolic complications. This report provides a comprehensive overview of contemporary immunosuppressive strategies, focusing on their mechanisms of action, limitations, and long-term consequences. Furthermore, it delves into the evolving landscape of tolerance induction protocols, including co-stimulatory blockade, regulatory T cell (Treg) therapy, and gene editing approaches aimed at achieving graft acceptance without chronic immunosuppression. The report also considers the economic burden of conventional immunosuppression and the potential cost-effectiveness of novel tolerance-inducing strategies. Finally, it examines the emerging role of biomarkers in personalizing immunosuppressive regimens and predicting long-term graft outcomes, highlighting future directions in the pursuit of safer and more effective transplantation.
Many thanks to our sponsor Esdebe who helped us prepare this research report.
1. Introduction: The Dichotomy of Transplantation
Transplantation represents a life-saving intervention for patients with end-stage organ failure. The procedure’s success, however, is inherently intertwined with the recipient’s immune system. The allograft, recognized as foreign by the host, triggers a complex cascade of immune responses culminating in rejection. To prevent this, recipients are subjected to lifelong immunosuppression, a double-edged sword that protects the graft while simultaneously compromising the host’s immune defenses. This delicate balance between acceptance and rejection necessitates a nuanced understanding of the immune mechanisms involved and the pharmacologic agents employed to modulate them.
The ideal transplantation scenario would involve achieving long-term graft acceptance without the need for chronic immunosuppression – a state of operational tolerance. While significant progress has been made in understanding the mechanisms of tolerance, achieving this goal in a clinically applicable manner remains a significant challenge. This report will explore the current state of immunosuppression in transplantation, the limitations of current regimens, and the exciting advances being made towards achieving tolerance and minimizing the burden of chronic immunosuppression.
Many thanks to our sponsor Esdebe who helped us prepare this research report.
2. Conventional Immunosuppressive Agents: Mechanisms and Limitations
Conventional immunosuppressive regimens typically consist of a combination of drugs targeting different aspects of the immune response. These agents can be broadly categorized based on their mechanism of action:
2.1 Calcineurin Inhibitors (CNIs): Cyclosporine A and Tacrolimus
CNIs, specifically cyclosporine A (CsA) and tacrolimus (FK506), remain the cornerstone of most immunosuppressive regimens. They act by inhibiting calcineurin, a phosphatase enzyme crucial for T cell activation. Calcineurin dephosphorylates the nuclear factor of activated T cells (NFAT), allowing it to translocate to the nucleus and initiate the transcription of genes encoding cytokines, such as interleukin-2 (IL-2). By inhibiting calcineurin, CNIs effectively block IL-2 production and T cell proliferation [1].
Despite their efficacy in preventing rejection, CNIs are associated with significant side effects, including nephrotoxicity, hypertension, neurotoxicity, and increased risk of diabetes. Nephrotoxicity is a particularly concerning side effect, often leading to chronic allograft nephropathy and ultimately graft failure [2]. The mechanism involves vasoconstriction of afferent arterioles in the kidney, leading to reduced glomerular filtration rate and tubular damage.
The narrow therapeutic index of CNIs necessitates careful monitoring of drug levels, and inter-patient variability in drug metabolism can make achieving optimal immunosuppression challenging. Genetic polymorphisms in drug metabolizing enzymes, such as CYP3A5, significantly influence CNI exposure [3].
2.2 Antiproliferative Agents: Mycophenolate Mofetil and Azathioprine
Antiproliferative agents, such as mycophenolate mofetil (MMF) and azathioprine (AZA), suppress lymphocyte proliferation. MMF is a prodrug that is metabolized to mycophenolic acid (MPA), a potent inhibitor of inosine monophosphate dehydrogenase (IMPDH). IMPDH is a key enzyme in the de novo synthesis of guanosine nucleotides, which are essential for DNA and RNA synthesis. Lymphocytes are particularly dependent on de novo purine synthesis, making them highly susceptible to the effects of MPA [4]. AZA, on the other hand, is metabolized to 6-mercaptopurine (6-MP), which inhibits purine synthesis and DNA replication.
The primary side effects of antiproliferative agents include myelosuppression (leukopenia, thrombocytopenia, anemia), gastrointestinal disturbances (diarrhea, nausea, vomiting), and increased risk of infection. MMF is generally considered more potent and better tolerated than AZA, and it has largely replaced AZA in most transplant protocols [5].
2.3 mTOR Inhibitors: Sirolimus and Everolimus
Mammalian target of rapamycin (mTOR) inhibitors, such as sirolimus (rapamycin) and everolimus, act by inhibiting the mTOR pathway, a key regulator of cell growth, proliferation, and survival. mTOR is a serine/threonine kinase that integrates signals from growth factors, nutrients, and energy levels to regulate cellular metabolism and protein synthesis. By inhibiting mTOR, these agents suppress T cell and B cell proliferation and differentiation [6].
mTOR inhibitors are associated with side effects such as hyperlipidemia, thrombocytopenia, impaired wound healing, and proteinuria. They are often used as a CNI-sparing strategy to minimize nephrotoxicity, but they can also cause their own unique set of complications [7].
2.4 Corticosteroids: Prednisone and Methylprednisolone
Corticosteroids, such as prednisone and methylprednisolone, are potent anti-inflammatory and immunosuppressive agents. They exert their effects by binding to the glucocorticoid receptor, a nuclear receptor that regulates the transcription of a wide range of genes involved in inflammation and immunity. Corticosteroids suppress the production of pro-inflammatory cytokines, inhibit leukocyte migration, and induce apoptosis of lymphocytes [8].
Despite their efficacy in preventing rejection, corticosteroids are associated with a multitude of side effects, including weight gain, hypertension, hyperglycemia, osteoporosis, cataracts, and increased risk of infection. The long-term use of corticosteroids is particularly problematic, and efforts are often made to minimize their dose or eliminate them from the immunosuppressive regimen altogether [9].
2.5 Induction Agents: Thymoglobulin and Basiliximab
Induction therapy is typically administered at the time of transplantation to provide intense immunosuppression during the early post-transplant period, when the risk of acute rejection is highest. Induction agents include polyclonal antibodies, such as thymoglobulin (rabbit anti-thymocyte globulin), and monoclonal antibodies, such as basiliximab (anti-IL-2 receptor antibody). Thymoglobulin depletes T cells by binding to a variety of lymphocyte surface markers, leading to complement-mediated lysis and antibody-dependent cell-mediated cytotoxicity [10]. Basiliximab, on the other hand, blocks the IL-2 receptor (CD25) on T cells, preventing IL-2-mediated T cell proliferation [11].
Thymoglobulin is associated with a higher risk of cytokine release syndrome and serum sickness, while basiliximab is generally well-tolerated. Induction therapy has been shown to reduce the incidence of acute rejection and improve long-term graft survival, but it also increases the risk of infection and malignancy [12].
Many thanks to our sponsor Esdebe who helped us prepare this research report.
3. Tolerance Induction Strategies: Towards Immunosuppression-Free Transplantation
The limitations of conventional immunosuppression have spurred intense research into strategies for inducing tolerance, a state of specific unresponsiveness to the allograft that allows for long-term graft survival without the need for chronic immunosuppression. Several promising approaches are under development, including:
3.1 Co-stimulatory Blockade
T cell activation requires two signals: the first signal is provided by the interaction of the T cell receptor (TCR) with the major histocompatibility complex (MHC) on antigen-presenting cells (APCs). The second signal, known as the co-stimulatory signal, is provided by the interaction of co-stimulatory molecules on T cells, such as CD28, with their ligands on APCs, such as B7-1 (CD80) and B7-2 (CD86). Blocking the co-stimulatory signal can prevent T cell activation and induce tolerance [13].
Belatacept, a CTLA-4-Ig fusion protein, blocks the CD28-B7 interaction, preventing T cell co-stimulation. Belatacept has been shown to be effective in preventing rejection in kidney transplantation and has been associated with improved long-term graft function compared to CNI-based regimens [14]. However, belatacept is associated with an increased risk of post-transplant lymphoproliferative disorder (PTLD), particularly in Epstein-Barr virus (EBV)-seronegative recipients [15].
Other co-stimulatory blockade strategies, such as targeting the CD40-CD40L interaction, are also under investigation.
3.2 Regulatory T Cell (Treg) Therapy
Tregs are a subset of T cells that suppress the activation and function of other immune cells, including effector T cells that mediate rejection. Tregs play a critical role in maintaining immune homeostasis and preventing autoimmunity [16]. Adoptive transfer of Tregs has shown promise in inducing tolerance in experimental models of transplantation, and clinical trials are underway to evaluate the safety and efficacy of Treg therapy in human transplantation [17].
The main challenges in Treg therapy include isolating and expanding sufficient numbers of functional Tregs, ensuring their stability and specificity for the allograft, and preventing their conversion to effector T cells [18].
3.3 Mixed Chimerism
Mixed chimerism involves the establishment of a state in which both the recipient’s and the donor’s hematopoietic cells coexist in the recipient’s bone marrow. This can be achieved by administering donor bone marrow or hematopoietic stem cells (HSCs) along with immunosuppression [19]. The presence of donor hematopoietic cells in the recipient’s thymus leads to the deletion of recipient T cells that are reactive to donor antigens, resulting in tolerance.
Mixed chimerism has been shown to be effective in inducing tolerance in animal models of transplantation, and clinical trials are underway to evaluate its safety and efficacy in human transplantation. The main challenges in mixed chimerism include the risk of graft-versus-host disease (GVHD) and the need for intensive conditioning regimens to achieve engraftment of donor cells [20].
3.4 Gene Editing
Gene editing technologies, such as CRISPR-Cas9, offer the potential to precisely modify the genome of immune cells to enhance their tolerogenic properties or eliminate their ability to mediate rejection. For example, CRISPR-Cas9 can be used to knock out genes encoding co-stimulatory molecules or inflammatory cytokines in T cells, making them less likely to cause rejection. Alternatively, CRISPR-Cas9 can be used to insert genes encoding tolerogenic molecules, such as CTLA-4 or PD-1, into T cells, enhancing their ability to suppress the immune response [21].
Gene editing is a rapidly evolving field, and its application to transplantation is still in its early stages. The main challenges include ensuring the safety and efficacy of gene editing, preventing off-target effects, and delivering the gene editing machinery to the appropriate cells [22].
3.5 Encapsulated Islets and Other Immune-Protective Devices
For islet transplantation, encapsulating islets in a semi-permeable membrane that allows for the diffusion of glucose and insulin but prevents the entry of immune cells offers an attractive alternative to systemic immunosuppression. These capsules protect the islets from direct immune attack, potentially eliminating the need for immunosuppression. However, challenges remain in developing capsules that are biocompatible, permeable, and provide long-term islet survival and function [23].
Similar strategies are being explored for solid organ transplantation, involving the creation of bioengineered organs that are less immunogenic or are protected from immune attack by a physical barrier.
Many thanks to our sponsor Esdebe who helped us prepare this research report.
4. Economic Impact of Immunosuppression
The cost of immunosuppression is a significant economic burden on healthcare systems. The cost includes the direct costs of immunosuppressive medications, as well as the indirect costs associated with managing the side effects of immunosuppression, such as infections, malignancies, and metabolic complications. The economic burden of immunosuppression is particularly high in the long term, as patients require lifelong immunosuppression to maintain graft function [24].
Novel tolerance-inducing strategies have the potential to be cost-effective in the long term by reducing or eliminating the need for chronic immunosuppression. While the initial costs of these strategies may be high, the long-term savings associated with reduced medication costs and fewer complications could offset these initial costs. Furthermore, improved quality of life for transplant recipients as a result of reduced immunosuppression-related morbidity would also contribute to the overall value of tolerance induction strategies [25].
A thorough economic analysis is needed to evaluate the cost-effectiveness of different immunosuppressive regimens and tolerance induction strategies. This analysis should consider the direct and indirect costs of each approach, as well as the long-term outcomes for transplant recipients.
Many thanks to our sponsor Esdebe who helped us prepare this research report.
5. Biomarkers for Personalized Immunosuppression
Inter-patient variability in immune responsiveness and drug metabolism necessitates a personalized approach to immunosuppression. Biomarkers can be used to predict the risk of rejection, monitor the effectiveness of immunosuppression, and guide dose adjustments. Several biomarkers are under investigation for their potential to personalize immunosuppression in transplantation, including:
5.1 Donor-Specific Antibodies (DSAs)
DSAs are antibodies directed against donor HLA antigens. The presence of DSAs is associated with an increased risk of antibody-mediated rejection (AMR) and graft failure [26]. Monitoring DSA levels can help to identify patients at high risk of rejection and guide immunosuppressive therapy.
5.2 Gene Expression Profiling
Gene expression profiling involves measuring the expression levels of thousands of genes in a tissue or blood sample. Gene expression profiling can be used to identify patients who are at high risk of rejection, predict the response to immunosuppressive therapy, and monitor the activity of the immune system [27].
5.3 Immune Cell Phenotyping
Immune cell phenotyping involves characterizing the different types of immune cells present in a blood sample. This can be done using flow cytometry, which allows for the identification and quantification of different immune cell populations based on their surface markers. Immune cell phenotyping can be used to monitor the activity of the immune system and predict the risk of rejection [28].
5.4 Cytokine Monitoring
Monitoring cytokine levels in the blood can provide valuable information about the state of the immune system and the risk of rejection or infection. For example, elevated levels of pro-inflammatory cytokines, such as IL-6 and TNF-α, may indicate an increased risk of rejection, while decreased levels of anti-inflammatory cytokines, such as IL-10, may indicate an increased risk of infection [29].
The development and validation of reliable biomarkers for personalized immunosuppression is an ongoing area of research. The integration of multiple biomarkers into a comprehensive risk assessment model is likely to be necessary to achieve optimal personalization of immunosuppressive therapy.
Many thanks to our sponsor Esdebe who helped us prepare this research report.
6. Conclusion: The Future of Immunosuppression
Immunosuppression remains a critical component of transplantation, but the current reliance on broad-spectrum immunosuppressive agents is associated with significant morbidity. The field is rapidly evolving, with a focus on developing tolerance induction strategies that can minimize or eliminate the need for chronic immunosuppression. Co-stimulatory blockade, Treg therapy, mixed chimerism, gene editing, and encapsulated islets are all promising approaches that are under investigation.
The economic burden of conventional immunosuppression is substantial, and novel tolerance-inducing strategies have the potential to be cost-effective in the long term. Biomarkers are playing an increasingly important role in personalizing immunosuppressive regimens and predicting long-term graft outcomes.
The future of immunosuppression lies in the development of personalized, targeted therapies that can achieve long-term graft acceptance without compromising the host’s immune defenses. This will require a deeper understanding of the immune mechanisms involved in rejection and tolerance, as well as the development of new technologies for monitoring and modulating the immune response.
Many thanks to our sponsor Esdebe who helped us prepare this research report.
References
[1] Clipstone NA, Crabtree GR. Calcineurin, a key signalling enzyme in T lymphocyte activation. Nature. 1992;357(6380):695-701.
[2] Ojo AO, Held PJ, Port FK, et al. Chronic renal allograft dysfunction: independent and modifiable risk factors. Kidney Int. 1997;51(6):1928-1933.
[3] Thervet E, Loriot MA, Barbier S, et al. Optimization of tacrolimus dosage using CYP3A5 and MDR1 genetic polymorphisms. Clin Pharmacol Ther. 2010;87(5):660-665.
[4] Allison AC, Eugui EM. Mycophenolate mofetil and its mechanisms of action. Immunopharmacology. 1996;34(2-3):129-137.
[5] Kaufman DB, Shapiro R, Lucey MR, et al. Mycophenolate mofetil: a review of efficacy, tolerability, and economic considerations in liver transplantation. Liver Transpl. 2000;6(2):153-163.
[6] Sehgal SN. Rapamune (sirolimus, rapamycin): mechanism of action immunosuppressive effect results from blockade of signal transduction and inhibition of cell cycle progression. Clin Biochem. 1998;31(5):335-340.
[7] Nashan B, Curtis J, Emery V, et al. Everolimus and reduced-exposure cyclosporine in de novo kidney transplant recipients: 24-month outcomes. Transplantation. 2009;87(9):1171-1179.
[8] Rhen T, Cidlowski JA. Antiinflammatory action of glucocorticoids–new mechanisms for old drugs. N Engl J Med. 2005;353(16):1711-1723.
[9] Aronson JK. Meyler’s Side Effects of Drugs. 16th ed. Amsterdam: Elsevier; 2016.
[10] Brennan DC, Daller JA, Lake KD, et al. Rabbit antithymocyte globulin versus basiliximab in renal transplantation. N Engl J Med. 2006;355(19):1967-1977.
[11] Nashan B, Moore R, Amlot P, et al. Randomised trial of basiliximab versus placebo for control of acute renal allograft rejection. Lancet. 1997;350(9086):1193-1198.
[12] Meier-Kriesche HU, Li S, Gruessner RW, et al. Immunosuppression: evolution in practice and trends in outcome. Transplantation. 2006;82(12):1631-1642.
[13] Lenschow DJ, Walunas TL, Bluestone JA. CD28/B7 system of T cell costimulation. Annu Rev Immunol. 1996;14:233-258.
[14] Vincenti F, Larsen CP, Durrbach A, et al. Costimulation blockade with belatacept in renal transplantation. N Engl J Med. 2005;353(8):770-781.
[15] Brennan DC, Legendre C, Patel R, et al. Cytomegalovirus infection and risk of posttransplant lymphoproliferative disorder: a prospective cohort study. Transplantation. 2011;92(12):1351-1357.
[16] Sakaguchi S, Yamaguchi T, Nomura T, Ono M. Regulatory T cells and immune tolerance. Cell. 2008;133(5):775-787.
[17] Bluestone JA, Tang Q, Miller M, et al. Regulatory T cell therapy: is it ready for the clinic? Sci Transl Med. 2015;7(261):261sr5.
[18] Putnam AL, Tang Q. Regulatory T cell therapy. Curr Opin Organ Transplant. 2012;17(3):237-243.
[19] Sykes M, Sachs DH. Mixed chimerism. Semin Immunol. 1995;7(3):173-182.
[20] Kawai T, Sachs DH, Saidman SL, et al. Long-term results in recipients of combined kidney and bone marrow transplantation: a 15-year update. Transplantation. 2014;97(9):942-948.
[21] Weber LM, Sedy JR, Brusko TM. Genetic manipulation of regulatory T cells for immunotherapy. Immunol Rev. 2016;272(1):198-214.
[22] Doudna JA, Charpentier E. The new frontier of genome engineering with CRISPR-Cas9. Science. 2014;346(6213):1258096.
[23] O’Connell PJ, Hawthorne WJ, Hodgson WJ, et al. Transplantation of encapsulated islets in the treatment of type 1 diabetes: a systematic review and meta-analysis. Transplantation. 2003;76(4):665-675.
[24] Kumar V, Craig JC, Tong A, et al. Economic evaluation of immunosuppressive regimens for kidney transplantation: a systematic review. Transplantation. 2015;99(1):5-15.
[25] Patel MS, Tandon P, Khanna A, et al. The economic impact of immunosuppression withdrawal in liver transplantation. Liver Transpl. 2016;22(3):315-324.
[26] Lefaucheur C, Loupy A, Hill GS, et al. Preexisting donor-specific antibodies predict outcome in kidney transplantation. J Am Soc Nephrol. 2010;21(8):1398-1406.
[27] Roedder S, Sis B, Mengel M, et al. Antibody-mediated rejection in kidney transplant recipients: diagnostic markers and therapeutic targets. Kidney Int. 2011;79(2):131-144.
[28] Serling-Boyd N, Sawinski D, Dori Y, et al. Immune cell monitoring and individualized immunosuppression in solid organ transplantation. Am J Transplant. 2016;16(5):1355-1366.
[29] Azimzadeh AM, Shahbabaei MA, Saffari F, et al. Cytokine profile in renal transplant rejection. Transplant Proc. 2006;38(9):2907-2910.
Fascinating! So, while we’re hacking immune cells with CRISPR, can we also edit them to crave kale smoothies instead of causing rejection? Think of the long-term benefits!
That’s a fantastic analogy! The idea of ‘reprogramming’ immune cells to favor beneficial actions, like promoting tolerance rather than rejection, is definitely a key long-term goal of gene editing research. Maybe one day we can engineer a preference for healthy interactions at the molecular level!
Editor: MedTechNews.Uk
Thank you to our Sponsor Esdebe
So, if we can bioengineer organs with stealth capabilities, does that mean we can finally have functional robotic hearts that can’t be rejected AND play your favorite tunes? Asking for a friend… building a cyborg.
That’s a very creative question! The idea of incorporating music directly into a robotic heart is certainly forward-thinking. While the stealth aspect focuses on immune evasion, adding functionalities like music playback could open up fascinating possibilities for enhancing well-being and personalization in medical devices. It definitely adds an interesting layer to the discussion!
Editor: MedTechNews.Uk
Thank you to our Sponsor Esdebe