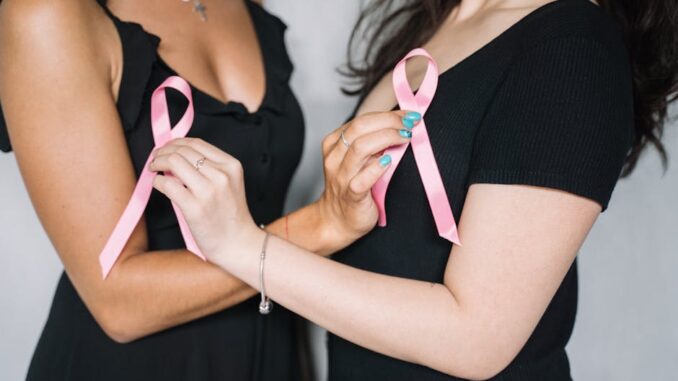
Abstract
Cancer remains a leading cause of morbidity and mortality worldwide, despite significant advancements in therapeutic strategies. This research report provides a comprehensive overview of the current state of cancer therapies, encompassing conventional modalities like chemotherapy and radiotherapy, as well as targeted therapies and immunotherapies. We delve into the complexities of drug delivery to tumors, highlighting the challenges posed by the tumor microenvironment (TME) and mechanisms of drug resistance. The report further explores the evolving landscape of personalized medicine in oncology, emphasizing the role of molecular profiling and biomarker-driven treatment decisions. A crucial aspect of the review is the examination of imaging techniques in cancer diagnosis, treatment monitoring, and response assessment. We also discuss the potential transformative impact of novel imaging techniques that are poised to improve cancer outcomes. Finally, the ethical implications of cancer treatment, including access to care, informed consent, and the responsible use of advanced technologies, are addressed. This report aims to provide a state-of-the-art perspective for experts in the field, highlighting current limitations, future directions, and crucial ethical considerations in the ongoing battle against cancer.
Many thanks to our sponsor Esdebe who helped us prepare this research report.
1. Introduction
Cancer is a complex and heterogeneous group of diseases characterized by uncontrolled cell growth and the potential to invade and metastasize to other parts of the body. The global burden of cancer continues to rise, with an estimated 19.3 million new cases and nearly 10 million cancer-related deaths occurring in 2020 (Sung et al., 2021). This alarming statistic underscores the urgent need for more effective and innovative strategies for cancer prevention, diagnosis, and treatment.
Over the past few decades, significant progress has been made in understanding the molecular basis of cancer and developing novel therapeutic approaches. Conventional therapies such as surgery, chemotherapy, and radiation therapy have remained cornerstones of cancer treatment. However, these modalities often lack specificity and can cause significant side effects due to their effects on normal cells. The emergence of targeted therapies and immunotherapies has revolutionized cancer treatment, offering more selective and personalized approaches with the potential to improve patient outcomes. Nevertheless, challenges persist, including the development of drug resistance, the complexity of the tumor microenvironment (TME), and the need for improved diagnostic and monitoring tools.
This research report aims to provide a comprehensive overview of the current state of cancer therapeutics, highlighting the challenges and opportunities in this rapidly evolving field. We will explore the various therapeutic modalities, including conventional and targeted therapies, as well as immunotherapies. We will also discuss the complexities of drug delivery to tumors, the role of imaging in cancer management, and the ethical considerations associated with cancer treatment.
Many thanks to our sponsor Esdebe who helped us prepare this research report.
2. Current Landscape of Cancer Therapies
2.1. Conventional Therapies
-
Surgery: Surgical resection remains the primary treatment for many solid tumors, particularly in early stages. Advances in surgical techniques, such as minimally invasive surgery and robotic surgery, have improved patient outcomes by reducing morbidity and recovery time. However, surgery may not be feasible for tumors that are located in difficult-to-access areas or that have metastasized to distant sites.
-
Chemotherapy: Chemotherapy involves the use of cytotoxic drugs to kill cancer cells. These drugs typically target rapidly dividing cells, but they can also affect normal cells, leading to a range of side effects, including nausea, vomiting, hair loss, and fatigue. Chemotherapy is often used in combination with other therapies, such as surgery and radiation therapy. Traditional chemotherapeutics work broadly, disrupting DNA replication or cell division in a non-specific manner. Newer chemotherapeutic agents often have more specific targets, such as topoisomerases or microtubules, offering slightly improved specificity.
-
Radiation Therapy: Radiation therapy uses high-energy rays to damage the DNA of cancer cells, leading to their death. Radiation therapy can be delivered externally, using a machine that directs radiation beams to the tumor, or internally, using radioactive materials placed directly into or near the tumor. Side effects of radiation therapy depend on the location and dose of radiation, and may include skin irritation, fatigue, and nausea. Advances in radiation therapy techniques, such as intensity-modulated radiation therapy (IMRT) and stereotactic body radiation therapy (SBRT), have allowed for more precise delivery of radiation to tumors, minimizing damage to surrounding tissues.
2.2. Targeted Therapies
Targeted therapies are drugs that specifically target molecules involved in cancer cell growth and survival. These therapies are designed to be more selective than traditional chemotherapy drugs, with the goal of reducing side effects and improving efficacy. Targeted therapies can be broadly classified into small molecule inhibitors and monoclonal antibodies.
-
Small Molecule Inhibitors: These drugs are typically designed to inhibit the activity of specific enzymes or proteins that are essential for cancer cell growth and survival. Examples include tyrosine kinase inhibitors (TKIs), which target receptor tyrosine kinases (RTKs) involved in cell signaling, and proteasome inhibitors, which block the degradation of proteins, leading to cancer cell death. For example, imatinib revolutionized the treatment of chronic myeloid leukemia (CML) by specifically inhibiting the BCR-ABL tyrosine kinase. The rise of resistance to small molecule inhibitors, however, is a major challenge.
-
Monoclonal Antibodies: These are antibodies that are designed to recognize and bind to specific antigens on cancer cells. Monoclonal antibodies can work through several mechanisms, including blocking the activity of growth factors or receptors, triggering antibody-dependent cell-mediated cytotoxicity (ADCC), or delivering cytotoxic drugs or radiation directly to cancer cells. Trastuzumab, for instance, targets the HER2 receptor in breast cancer and has significantly improved outcomes for patients with HER2-positive tumors.
2.3. Immunotherapies
Immunotherapy harnesses the power of the immune system to fight cancer. These therapies aim to stimulate the immune system to recognize and attack cancer cells. Several types of immunotherapies are currently available, including immune checkpoint inhibitors, adoptive cell transfer, and cancer vaccines.
-
Immune Checkpoint Inhibitors: Immune checkpoints are molecules that regulate the activity of the immune system, preventing it from attacking healthy cells. Cancer cells can exploit these checkpoints to evade immune surveillance. Immune checkpoint inhibitors block the activity of these checkpoints, allowing the immune system to recognize and attack cancer cells. Examples include anti-CTLA-4 antibodies (e.g., ipilimumab) and anti-PD-1/PD-L1 antibodies (e.g., pembrolizumab, nivolumab). These agents have shown remarkable efficacy in treating a variety of cancers, including melanoma, lung cancer, and kidney cancer. However, immune checkpoint inhibitors can also cause immune-related adverse events, such as autoimmune disorders.
-
Adoptive Cell Transfer: Adoptive cell transfer involves collecting immune cells from a patient, modifying them in the laboratory to enhance their ability to recognize and attack cancer cells, and then infusing them back into the patient. Chimeric antigen receptor (CAR) T-cell therapy is a type of adoptive cell transfer that has shown remarkable success in treating hematologic malignancies, such as leukemia and lymphoma. In CAR T-cell therapy, T cells are genetically engineered to express a CAR that recognizes a specific antigen on cancer cells. However, CAR T-cell therapy can be associated with significant toxicities, such as cytokine release syndrome (CRS) and neurotoxicity.
-
Cancer Vaccines: Cancer vaccines aim to stimulate the immune system to recognize and attack cancer cells. These vaccines can be designed to target specific antigens on cancer cells or to boost the overall immune response. Cancer vaccines have shown some success in treating certain cancers, such as prostate cancer and melanoma, but more research is needed to develop more effective cancer vaccines.
Many thanks to our sponsor Esdebe who helped us prepare this research report.
3. Challenges in Drug Delivery to Tumors
Effective drug delivery to tumors is a major challenge in cancer therapy. Several factors can limit the amount of drug that reaches the tumor, including the tumor microenvironment (TME), drug resistance mechanisms, and systemic barriers.
3.1. Tumor Microenvironment (TME)
The TME is a complex and dynamic environment that surrounds the tumor and plays a critical role in cancer development and progression. The TME includes various cell types, such as fibroblasts, immune cells, and endothelial cells, as well as extracellular matrix (ECM) components, growth factors, and cytokines. The TME can create a barrier to drug delivery by increasing interstitial fluid pressure, decreasing blood vessel permeability, and promoting drug degradation. Furthermore, the TME can contribute to drug resistance by promoting the expression of drug efflux pumps and inhibiting apoptosis.
The TME can also influence the efficacy of immunotherapy. For example, immunosuppressive cells in the TME, such as myeloid-derived suppressor cells (MDSCs) and regulatory T cells (Tregs), can inhibit the activity of cytotoxic T cells, preventing them from attacking cancer cells. Strategies to modulate the TME, such as targeting immunosuppressive cells or disrupting ECM components, may improve drug delivery and enhance the efficacy of cancer therapies. The inherent hypoxia within many solid tumors also contributes to radioresistance and can alter drug metabolism pathways, often reducing the efficacy of traditional chemotherapeutics.
3.2. Drug Resistance
Drug resistance is a major obstacle to successful cancer therapy. Cancer cells can develop resistance to drugs through several mechanisms, including: enhanced drug efflux, mutations in drug targets, activation of alternative signaling pathways, and evasion of apoptosis. Multidrug resistance (MDR) is a common mechanism of drug resistance in cancer cells. MDR involves the overexpression of drug efflux pumps, such as P-glycoprotein (P-gp), which pump drugs out of the cell, reducing their intracellular concentration. Strategies to overcome drug resistance include the use of drug efflux pump inhibitors, the development of drugs that are not substrates for efflux pumps, and the use of combination therapies.
3.3. Systemic Barriers
Systemic barriers, such as the blood-brain barrier (BBB) and the blood-tumor barrier (BTB), can also limit drug delivery to tumors. The BBB is a highly selective barrier that protects the brain from harmful substances in the bloodstream. The BTB is similar to the BBB, but it is located within the tumor microenvironment. The BBB and BTB can prevent many drugs from reaching brain tumors, making them difficult to treat. Strategies to overcome these barriers include the use of focused ultrasound, nanoparticles, and receptor-mediated transport.
Many thanks to our sponsor Esdebe who helped us prepare this research report.
4. Targeted Therapies: A Deeper Dive
While Section 2.2 provided an overview of targeted therapies, this section will delve deeper into specific examples and their mechanisms of action, along with the challenges and future directions in this field.
4.1. Tyrosine Kinase Inhibitors (TKIs)
TKIs represent a significant advancement in targeted therapy. These drugs target receptor tyrosine kinases (RTKs), enzymes crucial for cell signaling and proliferation. RTKs are frequently mutated or overexpressed in various cancers, making them attractive therapeutic targets.
-
EGFR Inhibitors: Epidermal growth factor receptor (EGFR) inhibitors, such as gefitinib, erlotinib, and osimertinib, are used to treat non-small cell lung cancer (NSCLC) with EGFR-activating mutations. Osimertinib, a third-generation EGFR inhibitor, is particularly effective against tumors harboring the T790M resistance mutation, a common mechanism of acquired resistance to first-generation EGFR inhibitors. However, resistance to osimertinib can also develop, highlighting the need for continued research into novel EGFR inhibitors and combination therapies.
-
VEGFR Inhibitors: Vascular endothelial growth factor receptor (VEGFR) inhibitors, such as bevacizumab and sorafenib, target angiogenesis, the formation of new blood vessels that supply tumors with nutrients and oxygen. These drugs are used to treat various cancers, including colorectal cancer, kidney cancer, and hepatocellular carcinoma. Bevacizumab is a monoclonal antibody that binds to VEGF, preventing it from binding to its receptor. Sorafenib is a small molecule inhibitor that targets multiple kinases, including VEGFR and RAF kinases. While VEGFR inhibitors can be effective, they can also cause significant side effects, such as hypertension, proteinuria, and wound-healing complications.
4.2. PARP Inhibitors
PARP (poly (ADP-ribose) polymerase) inhibitors, such as olaparib, rucaparib, and talazoparib, target DNA repair mechanisms in cancer cells. These drugs are particularly effective in tumors with defects in homologous recombination repair (HRR), such as BRCA1/2 mutations. PARP inhibitors work by inhibiting PARP, an enzyme involved in DNA repair, leading to the accumulation of DNA damage and cell death. PARP inhibitors have shown remarkable efficacy in treating ovarian cancer, breast cancer, and prostate cancer with BRCA1/2 mutations. However, resistance to PARP inhibitors can develop, highlighting the need for strategies to overcome resistance, such as combining PARP inhibitors with other therapies or targeting alternative DNA repair pathways.
4.3. Challenges and Future Directions
While targeted therapies have revolutionized cancer treatment, several challenges remain. One major challenge is the development of resistance to targeted therapies. Cancer cells can develop resistance through various mechanisms, including mutations in drug targets, activation of alternative signaling pathways, and bypass mechanisms. Another challenge is the heterogeneity of tumors. Even within the same tumor type, there can be significant differences in the molecular characteristics of cancer cells, making it difficult to develop targeted therapies that are effective for all patients.
Future directions in targeted therapy include the development of novel targeted therapies that target new pathways or overcome resistance mechanisms, the use of combination therapies to target multiple pathways simultaneously, and the development of personalized medicine approaches to select the most appropriate targeted therapy for each patient based on the molecular characteristics of their tumor.
Many thanks to our sponsor Esdebe who helped us prepare this research report.
5. Personalized Medicine Approaches in Oncology
Personalized medicine, also known as precision medicine, aims to tailor medical treatment to the individual characteristics of each patient. In oncology, personalized medicine involves using molecular profiling and biomarker-driven treatment decisions to select the most appropriate therapies for each patient based on the genetic and molecular characteristics of their tumor.
5.1. Molecular Profiling
Molecular profiling involves analyzing the DNA, RNA, and proteins of cancer cells to identify specific genetic and molecular alterations that are driving tumor growth and progression. Several techniques are used for molecular profiling, including next-generation sequencing (NGS), immunohistochemistry (IHC), and fluorescence in situ hybridization (FISH).
-
Next-Generation Sequencing (NGS): NGS allows for the rapid and comprehensive analysis of the entire genome or specific regions of the genome. NGS can be used to identify mutations, copy number variations, and structural rearrangements in cancer cells. NGS is increasingly being used to guide treatment decisions in oncology, allowing clinicians to select targeted therapies that are most likely to be effective for each patient. Furthermore, circulating tumor DNA (ctDNA) analysis, which utilizes NGS on blood samples, can provide a non-invasive way to monitor treatment response and detect early signs of recurrence.
-
Immunohistochemistry (IHC): IHC is a technique that uses antibodies to detect specific proteins in tissue samples. IHC can be used to identify the expression of specific biomarkers, such as HER2 in breast cancer or PD-L1 in lung cancer. IHC is often used in conjunction with NGS to guide treatment decisions.
-
Fluorescence In Situ Hybridization (FISH): FISH is a technique that uses fluorescent probes to detect specific DNA sequences in cells. FISH can be used to identify chromosomal abnormalities, such as gene amplifications or deletions. FISH is often used to diagnose and classify hematologic malignancies.
5.2. Biomarker-Driven Treatment Decisions
Biomarkers are measurable indicators of a biological state or condition. In oncology, biomarkers can be used to predict the response to treatment, monitor disease progression, and detect recurrence. Several biomarkers are currently used to guide treatment decisions in oncology, including:
-
ER/PR/HER2 in Breast Cancer: Estrogen receptor (ER), progesterone receptor (PR), and human epidermal growth factor receptor 2 (HER2) are biomarkers that are used to classify breast cancer and guide treatment decisions. ER-positive and PR-positive breast cancers are typically treated with hormone therapy, while HER2-positive breast cancers are treated with HER2-targeted therapies, such as trastuzumab and pertuzumab.
-
PD-L1 in Lung Cancer: Programmed death-ligand 1 (PD-L1) is a biomarker that is used to predict the response to immune checkpoint inhibitors in lung cancer. Patients with high PD-L1 expression are more likely to respond to immune checkpoint inhibitors.
-
Microsatellite Instability (MSI) in Colorectal Cancer: Microsatellite instability (MSI) is a biomarker that is used to identify patients with colorectal cancer who are likely to benefit from immune checkpoint inhibitors. MSI-high tumors have a high mutation rate and are more likely to be recognized by the immune system.
5.3. Challenges and Future Directions
While personalized medicine has made significant progress in oncology, several challenges remain. One major challenge is the cost and complexity of molecular profiling. NGS can be expensive, and the interpretation of NGS data can be complex and time-consuming. Another challenge is the lack of clinical trials to validate the use of biomarkers to guide treatment decisions. Many biomarkers have been identified, but their clinical utility has not been fully established.
Future directions in personalized medicine include the development of more affordable and accessible molecular profiling technologies, the development of clinical trials to validate the use of biomarkers to guide treatment decisions, and the integration of molecular profiling data with other clinical and demographic data to create personalized treatment plans for each patient.
Many thanks to our sponsor Esdebe who helped us prepare this research report.
6. The Role of Imaging in Cancer Diagnosis and Treatment Monitoring
Imaging plays a crucial role in all aspects of cancer management, from diagnosis and staging to treatment planning and monitoring. Various imaging modalities are used in oncology, including:
-
X-ray: X-ray imaging is a quick and inexpensive way to visualize bones and other dense tissues. X-ray imaging is often used to screen for lung cancer and to detect bone metastases.
-
Computed Tomography (CT): CT scans provide detailed cross-sectional images of the body. CT scans are used to diagnose and stage cancer, to monitor treatment response, and to detect recurrence.
-
Magnetic Resonance Imaging (MRI): MRI uses magnetic fields and radio waves to create detailed images of soft tissues. MRI is used to diagnose and stage cancer, to monitor treatment response, and to detect recurrence. MRI is particularly useful for imaging the brain, spine, and liver.
-
Positron Emission Tomography (PET): PET scans use radioactive tracers to detect metabolic activity in the body. PET scans are used to diagnose and stage cancer, to monitor treatment response, and to detect recurrence. PET scans are particularly useful for detecting metastases.
-
Ultrasound: Ultrasound uses sound waves to create images of internal organs. Ultrasound is used to diagnose and stage cancer, to monitor treatment response, and to guide biopsies.
6.1. The Impact of New Imaging Techniques
Novel imaging techniques are constantly being developed to improve cancer diagnosis and treatment monitoring. Some of the most promising new imaging techniques include:
-
Multi-parametric MRI: Multi-parametric MRI combines multiple MRI techniques to provide more detailed information about the tumor microenvironment. Multi-parametric MRI can be used to assess tumor vascularity, cellularity, and metabolism.
-
Molecular Imaging: Molecular imaging uses radioactive tracers or contrast agents that bind to specific molecules in cancer cells. Molecular imaging can be used to detect cancer cells at an early stage and to monitor treatment response. An example of molecular imaging is PET imaging with radiolabeled antibodies that target specific tumor antigens.
-
Optical Imaging: Optical imaging uses light to image tissues. Optical imaging can be used to detect cancer cells at an early stage and to monitor treatment response. Optical imaging is often used in conjunction with endoscopy.
These advanced imaging techniques hold great promise for improving cancer outcomes by enabling earlier diagnosis, more accurate staging, and more personalized treatment strategies. They provide a wealth of information beyond simple anatomical structure, allowing for assessment of tumor biology and response to therapy on a molecular level.
Many thanks to our sponsor Esdebe who helped us prepare this research report.
7. Ethical Implications of Cancer Treatment
The treatment of cancer raises several important ethical considerations. These include access to care, informed consent, the responsible use of advanced technologies, and end-of-life care.
7.1. Access to Care
Access to cancer care is a major ethical issue. Many people around the world do not have access to the latest cancer treatments, either because they cannot afford them or because they are not available in their region. This creates a significant disparity in cancer outcomes between developed and developing countries. Efforts to improve access to cancer care include advocating for universal health coverage, promoting the development of affordable cancer drugs, and training healthcare professionals in low-resource settings.
7.2. Informed Consent
Informed consent is a fundamental ethical principle in medicine. Patients have the right to make informed decisions about their treatment, based on a clear understanding of the risks and benefits of each option. In cancer treatment, informed consent can be particularly challenging because of the complexity of the disease and the potential for serious side effects from treatment. It is essential that healthcare professionals provide patients with clear and unbiased information about their treatment options and that they respect the patient’s autonomy in making decisions.
7.3. Responsible Use of Advanced Technologies
Advanced technologies, such as targeted therapies, immunotherapies, and genomic testing, hold great promise for improving cancer outcomes. However, these technologies are often expensive and complex, and their use raises several ethical considerations. It is important to ensure that these technologies are used responsibly and equitably and that patients are not subjected to unnecessary risks. This includes conducting rigorous clinical trials to evaluate the safety and efficacy of new technologies, developing guidelines for their appropriate use, and providing adequate training for healthcare professionals.
7.4. End-of-Life Care
End-of-life care is a crucial aspect of cancer management. Many patients with advanced cancer experience significant pain and suffering, and it is important to provide them with compassionate and effective palliative care. Palliative care aims to relieve pain and other symptoms, improve quality of life, and provide emotional and spiritual support to patients and their families. Patients have the right to make decisions about their end-of-life care, including whether to continue treatment, to receive hospice care, or to pursue other options. It is essential that healthcare professionals respect the patient’s wishes and provide them with the best possible care at the end of life.
Many thanks to our sponsor Esdebe who helped us prepare this research report.
8. Conclusion
Cancer remains a formidable challenge, demanding continuous innovation and a multi-faceted approach. While conventional therapies continue to play a role, the advent of targeted therapies and immunotherapies has ushered in a new era of personalized medicine in oncology. The development and optimization of novel imaging techniques is also critical for improving diagnosis, treatment monitoring, and ultimately, patient outcomes. However, the complexities of drug delivery, the emergence of drug resistance, and the ever-evolving tumor microenvironment necessitate further research and development of innovative strategies.
Moreover, the ethical implications of cancer treatment, including access to care, informed consent, and the responsible use of advanced technologies, must be carefully considered to ensure that all patients receive the best possible care. By addressing these challenges and embracing new technologies while upholding ethical principles, we can continue to make progress in the fight against cancer and improve the lives of patients and their families. Future research should focus on developing combination therapies that target multiple pathways simultaneously, overcoming drug resistance mechanisms, and modulating the tumor microenvironment to enhance drug delivery and immune responses. Furthermore, increased efforts are needed to improve access to cancer care in low-resource settings and to ensure that all patients have the opportunity to benefit from the latest advances in cancer treatment.
Many thanks to our sponsor Esdebe who helped us prepare this research report.
References
-
Sung, H., Ferlay, J., Siegel, R. L., Laversanne, M., Soerjomataram, I., Jemal, A., & Bray, F. (2021). Global cancer statistics 2020: GLOBOCAN estimates of incidence and mortality worldwide for 36 cancers in 185 countries. CA: a cancer journal for clinicians, 71(3), 209-249.
-
Hanahan, D. (2022). Hallmarks of Cancer: New Dimensions. Cancer Discovery, 12(1), 31-46.
-
National Cancer Institute. (n.d.). Targeted Cancer Therapies. Retrieved from https://www.cancer.gov/about-cancer/treatment/types/targeted-therapies
-
American Cancer Society. (n.d.). How Chemotherapy Drugs Work. Retrieved from https://www.cancer.org/treatment/understanding-your-treatment/chemotherapy/how-chemotherapy-drugs-work.html
-
Couzin-Frankel, J. (2013). Cancer immunotherapy. Science, 342(6165), 1432-1433.
-
Sharma, P., & Allison, J. P. (2015). Immune checkpoint targeting in cancer therapy: paradigm shifts, challenges and opportunities. Cell, 161(2), 205-214.
The discussion of ethical implications, especially regarding access to care, is critical. How can we ensure equitable access to cutting-edge cancer treatments and diagnostics globally, particularly in underserved communities and developing nations?
Thanks for highlighting the ethical dimensions! The question of equitable access is so important. We need collaborative efforts involving governments, pharmaceutical companies, and global health organizations to explore tiered pricing models, technology transfer initiatives, and capacity building in underserved regions. Further discussion on these models would be welcome!
Editor: MedTechNews.Uk
Thank you to our Sponsor Esdebe