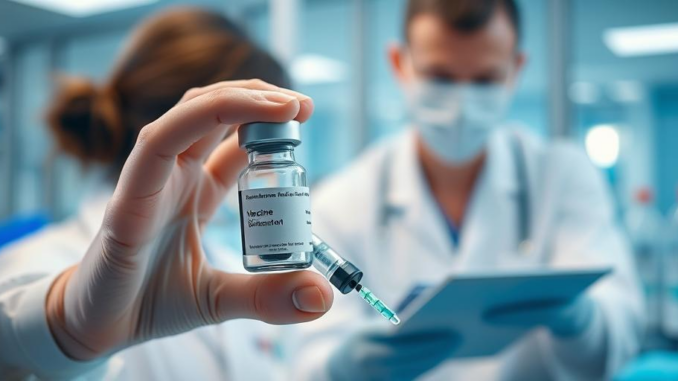
Abstract
Vaccines represent one of the most impactful achievements of modern medicine, playing a pivotal role in preventing infectious diseases and reducing global mortality rates. This research report provides a comprehensive overview of vaccine development, tracing its historical evolution from early empirical observations to the sophisticated biotechnological approaches of today. We delve into the diverse types of vaccines, including live-attenuated, inactivated, subunit, toxoid, and nucleic acid-based vaccines, examining their mechanisms of action, efficacy profiles, and potential limitations. A significant portion of the report is dedicated to the rapidly evolving field of vaccine delivery systems, with a comparative analysis of traditional intramuscular injections versus novel methods such as intranasal, transdermal, and oral administration. Furthermore, we explore the current research trends shaping the future of vaccinology, including personalized vaccines, mRNA vaccine technology, and strategies for overcoming vaccine hesitancy. This report aims to provide experts in the field with a nuanced understanding of the past, present, and future landscape of vaccine development.
Many thanks to our sponsor Esdebe who helped us prepare this research report.
1. Introduction
Vaccines are biological preparations designed to elicit a protective immune response against specific pathogens, thereby preventing or mitigating the severity of infectious diseases. The concept of vaccination dates back centuries, with early forms of variolation practiced in ancient China and India. However, Edward Jenner’s pioneering work in the late 18th century, using cowpox to protect against smallpox, is widely regarded as the foundation of modern vaccinology. The eradication of smallpox, a testament to the power of vaccination, stands as one of the greatest public health achievements in human history.
Since Jenner’s groundbreaking discovery, vaccine development has undergone a remarkable transformation, driven by advancements in immunology, microbiology, and biotechnology. The 20th and 21st centuries have witnessed the development of vaccines against a wide range of infectious diseases, including polio, measles, mumps, rubella, influenza, and human papillomavirus (HPV). These vaccines have significantly reduced the global burden of disease and improved public health outcomes.
However, vaccine development faces ongoing challenges. Emerging infectious diseases, such as the recent COVID-19 pandemic, demand rapid and effective vaccine responses. Furthermore, vaccine hesitancy, driven by misinformation and distrust, poses a significant threat to vaccination programs worldwide. This report aims to provide a comprehensive overview of vaccine development, from its historical roots to the cutting-edge technologies shaping its future, and to address the challenges and opportunities that lie ahead.
Many thanks to our sponsor Esdebe who helped us prepare this research report.
2. Types of Vaccines and Their Mechanisms of Action
Vaccines can be broadly classified into several categories based on their composition and mechanism of action:
2.1 Live-Attenuated Vaccines
Live-attenuated vaccines contain weakened versions of the pathogen. These vaccines are highly effective because they closely mimic a natural infection, stimulating both humoral and cell-mediated immunity. The attenuated pathogen replicates within the host, triggering a robust immune response that typically provides long-lasting protection. Examples of live-attenuated vaccines include those for measles, mumps, rubella (MMR), varicella (chickenpox), and yellow fever. While highly effective, live-attenuated vaccines are generally contraindicated in immunocompromised individuals and pregnant women due to the risk of causing symptomatic infection.
2.2 Inactivated Vaccines
Inactivated vaccines contain pathogens that have been killed or inactivated by chemical or physical means. These vaccines are generally safer than live-attenuated vaccines because they cannot replicate in the host. However, they typically induce a weaker immune response and require multiple doses (booster shots) to achieve long-lasting protection. Examples of inactivated vaccines include those for polio (IPV), influenza, hepatitis A, and rabies. The inactivation process can sometimes alter the antigenic structure of the pathogen, potentially reducing the efficacy of the vaccine.
2.3 Subunit Vaccines
Subunit vaccines contain only specific components of the pathogen, such as proteins, polysaccharides, or capsular antigens. These vaccines are highly safe and well-tolerated because they do not contain any live or inactivated pathogens. However, they often require adjuvants, substances that enhance the immune response, to achieve sufficient efficacy. Examples of subunit vaccines include those for hepatitis B, human papillomavirus (HPV), and pneumococcal disease. The selection of the appropriate subunit and adjuvant is crucial for optimizing the immunogenicity of these vaccines.
2.4 Toxoid Vaccines
Toxoid vaccines contain inactivated toxins produced by the pathogen. These vaccines are used to protect against diseases caused by bacterial toxins, such as tetanus and diphtheria. The toxoid induces an immune response that neutralizes the toxin, preventing it from causing harm. Toxoid vaccines are generally safe and effective, but they often require booster shots to maintain immunity.
2.5 Nucleic Acid Vaccines (DNA and mRNA)
Nucleic acid vaccines represent a revolutionary approach to vaccine development. These vaccines contain genetic material (DNA or mRNA) encoding for specific antigens of the pathogen. Upon injection, the host cells take up the genetic material and produce the antigens, which then stimulate an immune response. mRNA vaccines, in particular, have gained prominence due to their rapid development and high efficacy, as demonstrated by the COVID-19 vaccines developed by Pfizer-BioNTech and Moderna. Nucleic acid vaccines offer several advantages, including ease of manufacturing, rapid scalability, and the ability to induce both humoral and cell-mediated immunity. However, challenges remain in optimizing delivery methods and addressing potential safety concerns.
Many thanks to our sponsor Esdebe who helped us prepare this research report.
3. Vaccine Delivery Systems: A Comparative Analysis
Traditional vaccine delivery relies primarily on intramuscular (IM) or subcutaneous (SC) injections. However, these methods have limitations, including pain, needle phobia, and the need for trained healthcare professionals. Novel vaccine delivery systems are being developed to overcome these limitations and improve vaccine accessibility, efficacy, and patient compliance.
3.1 Intramuscular and Subcutaneous Injections
Intramuscular (IM) and subcutaneous (SC) injections are the most common routes of vaccine administration. IM injections deliver the vaccine into the muscle tissue, while SC injections deliver it into the subcutaneous tissue. Both routes elicit systemic immune responses, but IM injections may induce a stronger immune response due to the higher vascularity of muscle tissue. The efficacy of IM and SC injections depends on factors such as vaccine formulation, injection site, and individual immune response.
3.2 Intranasal Vaccines
Intranasal vaccines are administered through the nasal passages, targeting the mucosal immune system. The nasal mucosa is rich in immune cells, making it an ideal site for inducing both systemic and mucosal immunity. Intranasal vaccines can stimulate the production of IgA antibodies, which provide protection against pathogens at the site of entry. Furthermore, intranasal administration is non-invasive and painless, potentially improving patient compliance. The iNCOVACC vaccine discussed previously is an example of this.
However, intranasal vaccines face challenges such as mucociliary clearance, which can rapidly remove the vaccine from the nasal passages. Strategies to overcome this include using mucoadhesive formulations and nanoparticles to enhance vaccine retention.
3.3 Transdermal Vaccines
Transdermal vaccines are delivered through the skin using methods such as microneedles, patches, or jet injectors. These methods offer the advantage of being painless and non-invasive. Microneedles create microscopic pores in the skin, allowing the vaccine to penetrate the epidermis and dermis, where immune cells reside. Transdermal patches can provide sustained release of the vaccine over time, potentially enhancing the immune response. Jet injectors use high pressure to deliver the vaccine through the skin without the need for needles.
3.4 Oral Vaccines
Oral vaccines are administered through the mouth, targeting the gastrointestinal immune system. Oral vaccines offer the advantage of being easy to administer and potentially more acceptable to patients, especially children. However, oral vaccines face challenges such as degradation by stomach acid and enzymes, as well as the potential for immune tolerance. Strategies to overcome these challenges include encapsulating the vaccine in protective coatings and using adjuvants to enhance the immune response.
3.5 Comparative Analysis
| Delivery Method | Advantages | Disadvantages | Immune Response | Target Population |
|—|—|—|—|—|
| IM/SC Injection | Well-established, reliable | Painful, needle phobia, requires trained personnel | Systemic | General population |
| Intranasal | Non-invasive, painless, mucosal immunity | Mucociliary clearance, potential for local irritation | Systemic and mucosal | General population |
| Transdermal | Painless, non-invasive, sustained release | Requires specialized devices, potential for skin irritation | Systemic | General population |
| Oral | Easy to administer, potentially more acceptable | Degradation in GI tract, potential for immune tolerance | Mucosal | General population, especially children |
The choice of vaccine delivery method depends on factors such as the type of vaccine, the target population, and the desired immune response. Intranasal, transdermal, and oral vaccines offer promising alternatives to traditional injections, but further research is needed to optimize their efficacy and safety.
Many thanks to our sponsor Esdebe who helped us prepare this research report.
4. Current Research Trends in Vaccine Technology
The field of vaccinology is constantly evolving, with ongoing research focused on developing more effective, safer, and more accessible vaccines. Several key trends are shaping the future of vaccine technology:
4.1 Personalized Vaccines
Personalized vaccines are tailored to the individual’s genetic makeup and immune status. This approach is particularly relevant for cancer vaccines, where the antigens are specific to the individual’s tumor. Personalized vaccines can be designed to target neoantigens, unique mutations that arise in cancer cells. mRNA technology is well-suited for developing personalized cancer vaccines, as the mRNA sequence can be rapidly customized to encode for the individual’s tumor-specific antigens.
4.2 mRNA Vaccine Technology
The rapid development and deployment of mRNA vaccines during the COVID-19 pandemic has revolutionized the field of vaccinology. mRNA vaccines offer several advantages, including rapid manufacturing, high efficacy, and the ability to induce both humoral and cell-mediated immunity. Ongoing research is focused on improving the stability and delivery of mRNA vaccines, as well as exploring their potential for other infectious diseases and cancer. Self-amplifying mRNA vaccines, which contain RNA that encodes for its own replication machinery, are also being investigated as a way to enhance the immune response and reduce the dose required.
4.3 Adjuvants and Immunomodulators
Adjuvants are substances that enhance the immune response to vaccines. Traditional adjuvants, such as aluminum salts, have been used for decades, but newer adjuvants are being developed to improve vaccine efficacy and broaden the immune response. Immunomodulators are substances that can modulate the immune system to enhance vaccine responses. Examples of immunomodulators include Toll-like receptor (TLR) agonists, which activate innate immune cells and promote adaptive immunity. The development of novel adjuvants and immunomodulators is crucial for improving the efficacy of subunit vaccines and vaccines for immunocompromised individuals.
4.4 Vaccine Delivery Systems for Improved Immunogenicity
As discussed earlier, novel vaccine delivery systems are being developed to improve vaccine accessibility, efficacy, and patient compliance. Research is focused on optimizing the design and formulation of these delivery systems to enhance vaccine uptake by immune cells and promote a robust immune response. Nanoparticles, liposomes, and microencapsulation are being explored as ways to protect the vaccine from degradation and target it to specific immune cells. The development of effective vaccine delivery systems is crucial for improving the immunogenicity of vaccines, especially for difficult-to-immunize populations such as infants and the elderly.
4.5 Strategies for Overcoming Vaccine Hesitancy
Vaccine hesitancy, defined as the delay in acceptance or refusal of vaccination despite availability of vaccine services, is a growing global health threat. Misinformation, distrust, and lack of awareness contribute to vaccine hesitancy. Research is needed to understand the drivers of vaccine hesitancy and develop effective strategies to address it. These strategies include improving communication about vaccines, building trust with communities, and addressing misinformation. Healthcare professionals play a crucial role in educating patients about the benefits of vaccination and addressing their concerns. Furthermore, public health campaigns can be used to promote vaccination and combat misinformation.
4.6 Structural Biology and Rational Vaccine Design
Advances in structural biology are enabling a more rational approach to vaccine design. By determining the three-dimensional structure of viral proteins, researchers can identify key epitopes, the regions of the protein that elicit a protective immune response. This information can be used to design vaccines that specifically target these epitopes, leading to more effective and broadly protective vaccines. Structure-based vaccine design is particularly relevant for viruses that undergo rapid mutation, such as influenza and HIV.
Many thanks to our sponsor Esdebe who helped us prepare this research report.
5. Ethical Considerations
The development and deployment of vaccines raise several ethical considerations. These include:
- Informed Consent: Individuals must be fully informed about the risks and benefits of vaccination before making a decision. This includes providing clear and accurate information about the vaccine, potential side effects, and alternative options. The principle of autonomy dictates that individuals have the right to make their own decisions about their health, free from coercion.
- Equity and Access: Vaccines should be accessible to all individuals, regardless of their socioeconomic status, geographic location, or other factors. Equitable distribution of vaccines is essential for achieving herd immunity and protecting vulnerable populations. Global efforts are needed to ensure that vaccines are available in low- and middle-income countries.
- Vaccine Safety: Ensuring the safety of vaccines is paramount. Rigorous clinical trials are required to assess the safety and efficacy of new vaccines before they are licensed for use. Post-market surveillance is also important for monitoring the safety of vaccines and identifying any rare adverse events. Transparency in reporting vaccine safety data is crucial for building public trust.
- Mandatory Vaccination: Mandatory vaccination policies raise ethical concerns about individual autonomy and freedom of choice. While mandatory vaccination may be necessary in certain circumstances to protect public health, it should be implemented only after careful consideration of the ethical implications and with appropriate safeguards in place. Accommodations should be made for individuals with legitimate medical exemptions.
- Animal Research: Animal research is often necessary for the development and testing of vaccines. The use of animals in research raises ethical concerns about animal welfare. Researchers have a responsibility to minimize animal suffering and to use alternative methods whenever possible.
Many thanks to our sponsor Esdebe who helped us prepare this research report.
6. Conclusion
Vaccines have revolutionized public health, preventing countless cases of infectious diseases and saving millions of lives. The field of vaccinology is constantly evolving, with ongoing research focused on developing more effective, safer, and more accessible vaccines. Novel vaccine technologies, such as mRNA vaccines and personalized vaccines, hold great promise for the future. However, vaccine hesitancy remains a significant challenge, requiring concerted efforts to improve communication, build trust, and address misinformation. By embracing innovation, addressing ethical concerns, and working collaboratively, we can harness the power of vaccines to protect global health and prevent future pandemics.
Many thanks to our sponsor Esdebe who helped us prepare this research report.
References
- Plotkin, S. A., Orenstein, W. A., Offit, P. A., & Edwards, K. M. (2017). Plotkin’s vaccines. Elsevier.
- Poland, G. A., Jacobson, R. M., & Ovsyannikova, I. G. (2021). New vaccine development. Mayo Clinic Proceedings, 96(2), 475-486.
- Rappuoli, R., Dormitzer, P. R., Del Giudice, G., & Di Mattia, G. (2011). Vaccines for the twenty-first century society. Nature Reviews Immunology, 11(12), 865-875.
- Khan, S., Ali, A., Khan, M. T., Nawaz, A., Zahid, M., Rehman, F. U., … & Bilal, M. (2021). COVID-19 vaccines: potential therapeutic options and immunological advancements. Biomedicine & Pharmacotherapy, 133, 111021.
- Callaway, E. (2021). How COVID vaccines conquered the world in record time. Nature, 599(7886), 542-548.
- Hotez, P. J. (2021). Preventing the next pandemic: Vaccine diplomacy in a time of anti-science. PLoS Biology, 19(2), e3001126.
- World Health Organization. (2014). Vaccine hesitancy: A review of causes. Strategic Advisory Group of Experts on Immunization (SAGE).
- Fine, P., Eames, K., & Heymann, D. L. (2011). “Herd immunity”: a rough guide. Clinical Infectious Diseases, 52(7), 911-916.
- Lurie, N., Manolio, T., Patterson, A. P., Collins, F., & Frieden, T. (2020). Developing COVID-19 vaccines at pandemic speed. New England Journal of Medicine, 382(21), 1969-1973.
- Sette, A., & Crotty, S. (2003). T-cell immunity in severe acute respiratory syndrome coronavirus infections. Expert Review of Vaccines, 2(4), 513-520.
- Zuckerman, J. (2009). Combining vaccines to enhance immunogenicity. Human Vaccines, 5(9), 557-567.
- Pulendran, B., & Ahmed, R. (2011). Systems vaccinology. Immunity, 34(4), 509-521.
- Crowe Jr, J. E. (2014). Nanomaterials for vaccine delivery. Science Translational Medicine, 6(239), 239ps7.
- Park, K., Pack, D. W., Allen, R. C., & Pun, S. H. (2005). Biodegradable polymers for oral delivery of peptides: challenges and opportunities. European Journal of Pharmaceutics and Biopharmaceutics, 60(3), 355-362.
- Schroeder, J. T., Bienias, J. L., & Crowe Jr, J. E. (2010). New technologies for vaccine delivery. Current Opinion in Immunology, 22(3), 341-347.
- Tregoning, J. S., Brown, H., Flight, K. E., Higham, S. L., Goodfellow, I., & Butcher, E. C. (2015). Intranasal vaccination for respiratory infectious diseases. Immunity, 43(3), 370-383.
- De Gregorio, E., Tritto, E., Baliou, S., Serruto, D., Rappuoli, R., & D’Oro, U. (2013). Vaccine adjuvants: mode of action and pharmaceutical perspectives. Journal of Pharmaceutical Sciences, 102(6), 1705-1717.
- Lambert, P. H., Laurent, P. E., Del Giudice, G., & Poolman, J. (2005). Adjuvants for human vaccines. Novartis Foundation Symposium, 270, 13-27; discussion 27-37, 266-270.
- Centers for Disease Control and Prevention. (n.d.). Understanding How Vaccines Work. Retrieved from https://www.cdc.gov/vaccines/hcp/conversations/understanding-vaccines.html
Be the first to comment