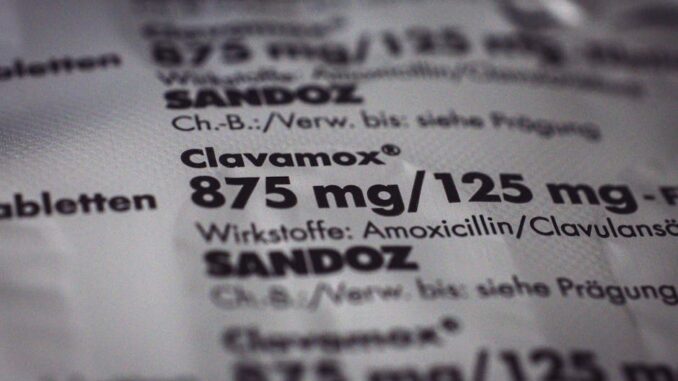
Abstract
Antimicrobial resistance (AMR) represents a significant and escalating threat to global public health and economic stability. This report provides a comprehensive overview of the AMR landscape, examining the intricate mechanisms driving resistance development across bacteria, viruses, fungi, and parasites. We delve into the specific pathogens of concern, highlighting their resistance profiles and clinical implications. Furthermore, we analyze the substantial economic burden associated with AMR, encompassing increased healthcare costs, reduced productivity, and broader societal impacts. The report critically evaluates the effectiveness of various intervention strategies, including antimicrobial stewardship programs, enhanced infection control practices, and the development of novel antimicrobial agents and alternative therapeutic approaches, such as bacteriophage therapy and immunomodulatory strategies. We also address the challenges in accurately assessing the true scope of AMR and propose avenues for future research and policy development to mitigate this critical global health threat. We conclude with a discussion of the need for a global, multi-sectoral ‘One Health’ approach to effectively combat AMR, emphasizing the interconnectedness of human, animal, and environmental health.
Many thanks to our sponsor Esdebe who helped us prepare this research report.
1. Introduction
Antimicrobial resistance (AMR) is no longer a looming threat; it is a present reality with profound consequences for human and animal health, global economies, and societal well-being. The widespread and often indiscriminate use of antimicrobial agents has driven the selection and proliferation of resistant microorganisms, rendering previously effective treatments obsolete. This complex issue transcends geographical boundaries and necessitates a multifaceted approach that encompasses understanding the underlying mechanisms of resistance, monitoring its spread, developing novel therapeutics, and implementing effective stewardship and infection control measures. This report aims to provide an in-depth analysis of the AMR landscape, focusing on the diverse mechanisms of resistance, the pathogens of greatest concern, the economic burden imposed by AMR, and the effectiveness of current and emerging intervention strategies. Furthermore, we will explore the challenges in quantifying and managing AMR and propose directions for future research and policy initiatives.
Many thanks to our sponsor Esdebe who helped us prepare this research report.
2. Mechanisms of Antimicrobial Resistance
The development of AMR is a complex evolutionary process driven by selective pressure exerted by antimicrobial agents. Microorganisms employ a variety of mechanisms to evade the effects of these drugs, which can be broadly classified into several categories:
2.1. Enzymatic Inactivation
A common mechanism of resistance involves the production of enzymes that inactivate or modify antimicrobial agents. Beta-lactamases, for instance, are enzymes that hydrolyze the beta-lactam ring of penicillin and cephalosporin antibiotics, rendering them ineffective. The emergence of extended-spectrum beta-lactamases (ESBLs) and carbapenemases has significantly broadened the spectrum of resistance, posing a major challenge in treating infections caused by Gram-negative bacteria. Similarly, aminoglycoside-modifying enzymes (AMEs) alter the structure of aminoglycoside antibiotics, preventing them from binding to their ribosomal target. The diversity and adaptability of these enzymes contribute significantly to the rapid spread of resistance.
2.2. Target Modification
Another strategy employed by microorganisms is to alter the target site of the antimicrobial agent, reducing its binding affinity and efficacy. Mutations in the genes encoding ribosomal proteins can confer resistance to macrolides, tetracyclines, and aminoglycosides. In bacteria, mutations in DNA gyrase or topoisomerase IV can lead to resistance to quinolones. In viruses, mutations in the reverse transcriptase or protease enzymes of HIV can confer resistance to antiretroviral drugs. The accumulation of such mutations over time can lead to high-level resistance, making infections extremely difficult to treat.
2.3. Efflux Pumps
Efflux pumps are transmembrane proteins that actively transport antimicrobial agents out of the cell, reducing their intracellular concentration and preventing them from reaching their target. Many bacteria possess multiple efflux pumps with broad substrate specificity, conferring resistance to a wide range of antibiotics. The overexpression of efflux pumps is often regulated by complex genetic mechanisms, contributing to the adaptive nature of resistance. Efflux pumps are also implicated in resistance to antifungal and antiviral drugs.
2.4. Reduced Permeability
Changes in the cell wall or membrane structure can reduce the permeability of microorganisms to antimicrobial agents, limiting their access to intracellular targets. This mechanism is particularly important in Gram-negative bacteria, which possess an outer membrane that acts as a barrier to many antibiotics. Mutations in porin proteins, which facilitate the entry of antibiotics into the cell, can reduce permeability and confer resistance. Similarly, changes in the lipid composition of fungal cell membranes can reduce the effectiveness of antifungal drugs.
2.5. Metabolic Bypass
Some microorganisms can develop alternative metabolic pathways that bypass the target of the antimicrobial agent, rendering it ineffective. For example, some bacteria can acquire an alternative dihydrofolate reductase (DHFR) enzyme that is resistant to trimethoprim, a drug that inhibits folate synthesis. This mechanism allows the bacteria to continue growing even in the presence of the antibiotic.
2.6 Horizontal Gene Transfer
Horizontal gene transfer (HGT) plays a crucial role in the rapid spread of AMR. Bacteria can acquire resistance genes from other bacteria through various mechanisms, including conjugation (transfer of plasmids), transduction (transfer of DNA by bacteriophages), and transformation (uptake of free DNA from the environment). Mobile genetic elements, such as plasmids, transposons, and integrons, often carry multiple resistance genes, facilitating the dissemination of multidrug resistance. The ease with which resistance genes can be transferred between bacteria underscores the importance of controlling the spread of resistant strains and preventing the emergence of new resistance mechanisms.
Many thanks to our sponsor Esdebe who helped us prepare this research report.
3. Specific Pathogens Involved in AMR
AMR is a problem across a wide range of pathogens, including bacteria, viruses, fungi, and parasites. However, certain pathogens are of particular concern due to their high prevalence, virulence, and resistance profiles.
3.1. Antibiotic-Resistant Bacteria
The World Health Organization (WHO) has identified several antibiotic-resistant bacteria as critical priorities for research and development of new antibiotics. These include:
- Carbapenem-resistant Acinetobacter baumannii: A highly virulent opportunistic pathogen that causes pneumonia, bloodstream infections, and wound infections, particularly in healthcare settings. Carbapenem resistance severely limits treatment options.
- Carbapenem-resistant Pseudomonas aeruginosa: Another opportunistic pathogen that causes a variety of infections, including pneumonia, bloodstream infections, and urinary tract infections. Carbapenem resistance is increasingly common, making treatment difficult.
- Carbapenem-resistant and ESBL-producing Enterobacteriaceae: A large family of bacteria that includes Escherichia coli and Klebsiella pneumoniae. These bacteria are common causes of urinary tract infections, bloodstream infections, and pneumonia. The emergence of carbapenem resistance and ESBL production in these bacteria poses a significant threat.
- Methicillin-resistant Staphylococcus aureus (MRSA): A common cause of skin and soft tissue infections, pneumonia, and bloodstream infections. MRSA is resistant to many commonly used antibiotics, including methicillin and other beta-lactams.
- Vancomycin-resistant Enterococcus (VRE): A common cause of bloodstream infections, urinary tract infections, and wound infections. VRE is resistant to vancomycin, a glycopeptide antibiotic that is often used as a last-line treatment for Gram-positive bacterial infections.
- Neisseria gonorrhoeae: The bacterium that causes gonorrhea, a sexually transmitted infection. Resistance to cephalosporins, the last-line antibiotics for gonorrhea, is increasing globally.
- Streptococcus pneumoniae: A common cause of pneumonia, meningitis, and otitis media. Resistance to penicillin and other antibiotics is increasing in some areas.
- Mycobacterium tuberculosis: The bacterium that causes tuberculosis (TB). Multidrug-resistant TB (MDR-TB) and extensively drug-resistant TB (XDR-TB) are major public health threats.
3.2. Antiviral-Resistant Viruses
Antiviral resistance is a growing concern for several viruses, including:
- Human immunodeficiency virus (HIV): Resistance to antiretroviral drugs is a major challenge in managing HIV infection. Regular monitoring of viral resistance is essential to guide treatment decisions.
- Influenza virus: Resistance to antiviral drugs such as oseltamivir and zanamivir can reduce the effectiveness of influenza treatment. Surveillance of influenza virus strains is important to track the emergence and spread of resistance.
- Hepatitis B virus (HBV): Resistance to antiviral drugs such as lamivudine and adefovir can occur in patients with chronic HBV infection. Regular monitoring of viral resistance is necessary to guide treatment decisions.
- Herpes simplex virus (HSV): Resistance to antiviral drugs such as acyclovir can occur in patients with HSV infections, particularly in immunocompromised individuals.
3.3. Antifungal-Resistant Fungi
Antifungal resistance is an emerging threat, particularly in immunocompromised patients. The following fungi are of particular concern:
- Candida species: Candida albicans is the most common cause of candidiasis, but other Candida species, such as Candida glabrata and Candida auris, are becoming increasingly resistant to antifungal drugs.
- Aspergillus species: Aspergillus fumigatus is the most common cause of invasive aspergillosis, a serious infection that can occur in immunocompromised patients. Resistance to azole antifungal drugs is increasing in Aspergillus fumigatus.
- Cryptococcus neoformans: A common cause of meningitis in immunocompromised patients, particularly those with HIV infection. Resistance to antifungal drugs such as fluconazole can occur in Cryptococcus neoformans.
3.4. Antiparasitic-Resistant Parasites
Antiparasitic resistance is a growing problem for several parasitic diseases, including:
- Plasmodium falciparum: The parasite that causes malaria. Resistance to antimalarial drugs such as chloroquine, artemisinin, and mefloquine is increasing in many parts of the world.
- Leishmania species: The parasites that cause leishmaniasis. Resistance to antimonial drugs, the first-line treatment for leishmaniasis, is increasing in some areas.
- Helminths (worms): Resistance to anthelmintic drugs is a growing problem for several helminth infections, including hookworm, roundworm, and whipworm.
Many thanks to our sponsor Esdebe who helped us prepare this research report.
4. Economic Burden of Antimicrobial Resistance
The economic burden of AMR is substantial and multifaceted, encompassing increased healthcare costs, reduced productivity, and broader societal impacts. It is crucial to recognize that the costs associated with AMR extend beyond the direct costs of treating resistant infections.
4.1. Increased Healthcare Costs
Resistant infections are more difficult and costly to treat than susceptible infections. Patients with resistant infections often require longer hospital stays, more intensive care, and more expensive antibiotics. The use of last-line antibiotics, which are often more toxic and have more side effects, also contributes to increased healthcare costs. Furthermore, the need for isolation and infection control measures to prevent the spread of resistant organisms adds to the overall cost of healthcare.
4.2. Reduced Productivity
AMR can lead to reduced productivity due to increased morbidity and mortality. Patients with resistant infections may be unable to work or attend school, leading to lost wages and reduced economic output. The premature death of individuals due to resistant infections also represents a significant loss of human capital.
4.3. Broader Societal Impacts
AMR can have broader societal impacts, including reduced access to healthcare, increased poverty, and decreased economic development. In low- and middle-income countries, where healthcare resources are limited, AMR can exacerbate existing health inequalities and undermine efforts to improve public health. The threat of AMR can also discourage investment in healthcare and tourism, further hindering economic development.
4.4 Challenges in Economic Burden Estimation
Accurately quantifying the economic burden of AMR presents several challenges. Data on the prevalence and incidence of resistant infections are often limited, particularly in low- and middle-income countries. Furthermore, it can be difficult to isolate the direct costs attributable to AMR from other factors that influence healthcare costs. The indirect costs of AMR, such as reduced productivity and broader societal impacts, are even more challenging to quantify. Despite these challenges, numerous studies have attempted to estimate the economic burden of AMR, with findings consistently highlighting the significant financial impact of this global health threat.
Many thanks to our sponsor Esdebe who helped us prepare this research report.
5. Effectiveness of Different Intervention Strategies
A comprehensive approach to combating AMR requires the implementation of multiple intervention strategies, including antimicrobial stewardship programs, enhanced infection control practices, and the development of novel antimicrobial agents and alternative therapies.
5.1. Antimicrobial Stewardship Programs
Antimicrobial stewardship programs (ASPs) aim to optimize the use of antimicrobial agents, ensuring that they are used appropriately, effectively, and safely. ASPs typically involve a multidisciplinary team of healthcare professionals, including physicians, pharmacists, nurses, and microbiologists. Key components of ASPs include:
- Developing and implementing guidelines for antimicrobial use: These guidelines should be based on evidence-based practices and tailored to the local context.
- Monitoring antimicrobial use: This involves tracking the use of antimicrobial agents and identifying areas where improvements can be made.
- Providing education and training to healthcare professionals: This helps to ensure that healthcare professionals are knowledgeable about antimicrobial resistance and appropriate antimicrobial use.
- Implementing interventions to improve antimicrobial use: These interventions may include restricting the use of certain antibiotics, requiring prior authorization for certain antibiotics, and providing feedback to prescribers on their prescribing practices.
Meta-analyses have consistently demonstrated that ASPs can reduce antimicrobial use, improve patient outcomes, and decrease the development of AMR. Effective ASPs require strong institutional support, dedicated resources, and ongoing monitoring and evaluation.
5.2. Infection Control Practices
Infection control practices are essential for preventing the spread of resistant microorganisms in healthcare settings. Key infection control measures include:
- Hand hygiene: Frequent and thorough hand hygiene is one of the most effective ways to prevent the spread of infections.
- Isolation precautions: Patients with known or suspected resistant infections should be placed in isolation to prevent transmission to other patients and healthcare workers.
- Environmental cleaning and disinfection: Regular cleaning and disinfection of environmental surfaces can help to reduce the spread of resistant organisms.
- Surveillance: Monitoring the incidence of resistant infections can help to identify outbreaks and track the effectiveness of infection control measures.
- Screening: Screening high-risk patients for colonization with resistant organisms can help to identify and isolate carriers.
Adherence to infection control practices is crucial for preventing the spread of AMR. However, implementing and maintaining effective infection control programs can be challenging, particularly in resource-limited settings.
5.3. Development of Novel Antimicrobial Agents
The development of novel antimicrobial agents is essential for combating AMR. However, the pipeline of new antibiotics is limited, and the rate of discovery has slowed in recent years. Several factors contribute to this challenge, including the high cost of drug development, the long development timelines, and the regulatory hurdles. Furthermore, the economic incentives for developing new antibiotics are often insufficient, as the return on investment may be low due to the relatively short duration of treatment and the potential for resistance to develop rapidly.
Despite these challenges, several new antimicrobial agents have been developed in recent years, including:
- Cephalosporin-beta-lactamase inhibitor combinations: These drugs combine a cephalosporin antibiotic with a beta-lactamase inhibitor, which protects the cephalosporin from degradation by beta-lactamases.
- Lipoglycopeptides: These drugs are similar to vancomycin but have improved activity against some resistant bacteria.
- Oxazolidinones: These drugs inhibit protein synthesis and are active against some resistant Gram-positive bacteria.
- Polymyxins: These drugs are last-line antibiotics that are active against some multidrug-resistant Gram-negative bacteria. However, they are also associated with significant toxicity.
Continued investment in research and development of novel antimicrobial agents is crucial for combating AMR. Innovative approaches, such as targeting novel bacterial pathways and developing immunomodulatory therapies, are needed to overcome the challenges of resistance.
5.4. Alternative Therapies
Alternative therapies, such as bacteriophage therapy and immunomodulatory strategies, offer promising approaches for combating AMR. Bacteriophages are viruses that infect and kill bacteria. Bacteriophage therapy involves using bacteriophages to treat bacterial infections. This approach has several advantages, including the ability to target specific bacteria, the potential for rapid development of new bacteriophages, and the lack of cross-resistance with antibiotics. However, bacteriophage therapy also faces challenges, including the potential for bacterial resistance to develop, the need for careful selection of bacteriophages, and the regulatory hurdles.
Immunomodulatory strategies aim to enhance the host’s immune response to infection. These strategies may involve using cytokines, antibodies, or other immune modulators to boost the immune system’s ability to clear infections. Immunomodulatory therapies may be particularly useful for treating infections caused by multidrug-resistant bacteria.
Many thanks to our sponsor Esdebe who helped us prepare this research report.
6. Challenges in Assessing the True Scope of AMR
Accurately assessing the true scope of AMR is critical for informing public health interventions and resource allocation. However, several challenges complicate this assessment.
6.1. Limited Surveillance Data
Many countries, particularly low- and middle-income countries, lack comprehensive surveillance systems for AMR. This lack of data makes it difficult to track the emergence and spread of resistant organisms and to assess the impact of interventions.
6.2. Inconsistent Diagnostic Practices
Inconsistent diagnostic practices can lead to underreporting or misreporting of resistant infections. Variations in laboratory testing methods, the use of different breakpoints for defining resistance, and the lack of standardized reporting mechanisms can all contribute to this problem.
6.3. Asymptomatic Colonization
Asymptomatic colonization with resistant organisms can be a significant source of transmission. However, routine screening for asymptomatic colonization is not always performed, leading to an underestimation of the true prevalence of AMR.
6.4. Data Integration and Analysis
Integrating and analyzing data from multiple sources, such as human, animal, and environmental health, is essential for understanding the complex dynamics of AMR. However, this requires collaboration between different sectors and the development of standardized data sharing mechanisms.
Many thanks to our sponsor Esdebe who helped us prepare this research report.
7. Future Research and Policy Directions
Addressing the global threat of AMR requires a sustained and coordinated effort across multiple sectors. Key areas for future research and policy development include:
7.1. Strengthening Surveillance Systems
Investing in the development and implementation of robust surveillance systems for AMR is essential. These systems should include data from human, animal, and environmental health and should be based on standardized methods and reporting mechanisms.
7.2. Promoting Antimicrobial Stewardship
Expanding and strengthening antimicrobial stewardship programs in all healthcare settings is crucial. These programs should be based on evidence-based practices and tailored to the local context.
7.3. Enhancing Infection Control
Implementing and maintaining effective infection control programs in all healthcare settings is essential for preventing the spread of resistant organisms. This requires adequate resources, training, and monitoring.
7.4. Supporting Research and Development
Investing in research and development of novel antimicrobial agents and alternative therapies is crucial. This requires public and private sector collaboration and the development of innovative financing mechanisms.
7.5. Promoting Public Awareness
Raising public awareness about AMR is essential for changing behaviors and promoting responsible antimicrobial use. This requires effective communication strategies and engagement with the public.
7.6. Fostering International Collaboration
AMR is a global problem that requires international collaboration. This includes sharing data, coordinating research efforts, and developing harmonized policies.
Many thanks to our sponsor Esdebe who helped us prepare this research report.
8. Conclusion
Antimicrobial resistance is a complex and multifaceted challenge that requires a comprehensive and coordinated response. Understanding the mechanisms of resistance, monitoring the spread of resistant organisms, developing novel therapeutics, and implementing effective stewardship and infection control measures are all essential for mitigating this critical global health threat. A ‘One Health’ approach, recognizing the interconnectedness of human, animal, and environmental health, is paramount to effectively combat AMR. Future research and policy efforts should focus on strengthening surveillance systems, promoting antimicrobial stewardship, enhancing infection control, supporting research and development, promoting public awareness, and fostering international collaboration. Only through a sustained and coordinated effort can we hope to preserve the effectiveness of antimicrobial agents and protect future generations from the devastating consequences of AMR.
Many thanks to our sponsor Esdebe who helped us prepare this research report.
References
- World Health Organization. (2014). Antimicrobial resistance: global report on surveillance 2014. WHO.
- O’Neill, J. (2016). Tackling drug-resistant infections globally: final report and recommendations. Review on Antimicrobial Resistance.
- Centers for Disease Control and Prevention. (2019). Antibiotic resistance threats in the United States, 2019. CDC.
- Spellberg, B., Guidos, R., Gilbert, D., Bradley, J., Boucher, H. W., Link, A., … & Bartlett, J. G. (2008). The epidemic of antibiotic-resistant infections: a call to action for the medical community. Archives of internal medicine, 168(12), 1323-1328.
- Ventola, C. L. (2015). The antibiotic resistance crisis: part 1: causes and threats. P&T, 40(4), 277.
- Laxminarayan, R., Duse, A., Wattal, C., Zaidi, A. K., Wertheim, H. F., Sumpradit, N., … & Cars, O. (2013). Antibiotic resistance—the need for global solutions. The Lancet infectious diseases, 13(12), 1057-1098.
- Read, A. F., & Woods, R. J. (2014). Antibiotic resistance management. Evolution, medicine, and public health, 2014(1), 147-159.
- Magiorakos, A. P., Srinivasan, A., Carey, R. B., Carmeli, Y., Falagas, M. E., Giske, C. G., … & Weber, J. T. (2012). Multidrug-resistant, extensively drug-resistant and pandrug-resistant bacteria: an international expert proposal for interim standard definitions for acquired resistance. Clinical microbiology and infection, 18(3), 268-281.
- Munita, J. M., & Arias, C. A. (2016). Mechanisms of antibiotic resistance. Microbial biotechnology, 9(5), 557-561.
- Blair, J. M., Webber, M. A., Baylay, A. J., Ogbolu, D. O., & Piddock, L. J. (2015). Molecular mechanisms of antibiotic resistance. Nature Reviews Microbiology, 13(1), 42.
- Davies, J., & Davies, D. (2010). Origins and evolution of antibiotic resistance. Microbiology and molecular biology reviews, 74(3), 417-433.
- Fair, R. J., & Tor, Y. (2014). Antibiotics and bacterial resistance in the 21st century. Perspectives in medicinal chemistry, 6, PMC14187.
- Livermore, D. M. (2000). Antibiotic resistance in staphylococci. International journal of antimicrobial agents, 16, S3-S10.
- Rice, L. B. (2008). Federal funding for the study of antimicrobial resistance in nosocomial pathogens: no ESKAPE. Journal of infectious diseases, 197(8), 1079-1081.
- Tacconelli, E., Carrara, E., Savini, V., Harbarth, S., Mendelson, M., Monnet, D. L., … & Hryniewicz, W. (2018). Discovery, research, and development of new antibiotics: the WHO priority list of antibiotic-resistant bacteria and fungi. The Lancet infectious diseases, 18(3), 318-327.
- Nathan, C., & Cars, O. (2014). Antibiotic resistance—problems, causes, and solutions. Bmj, 348, f2121.
- Klein, E. Y., & Laxminarayan, R. (2010). Estimating the contribution of human and livestock antimicrobial use to antimicrobial resistance. Clinical infectious diseases, 51(12), 1459-1464.
- Teillant, A., Laxminarayan, R., & Bonomo, R. A. (2015). Economics of antibiotic resistance: a systematic review. Expert review of anti-infective therapy, 13(7), 895-907.
- Roberts, R. R., Scott II, R. D., Hota, B., Kampe, L. M., Suppes, A. B., Cordell, R., … & Jernigan, J. A. (2009). Costs attributable to healthcare-acquired infections: a prospective multicenter cohort study. American journal of infection control, 37(4), 261-267.
- Bartsch, S. M., Nelson, R. E., McKinnell, J. A., Powell, M. M., Tian, J., Orenstein, E. W., … & Lee, B. Y. (2017). The potential economic burden of antibiotic resistance in the United States. Clinical infectious diseases, 65(12), 2029-2036.
- Davey, P., Brown, E., Fenelon, L., Finch, R., Gould, I., Littlejohns, P., … & Wilcox, M. (2005). Interventions to improve antibiotic prescribing practices for hospital inpatients. Cochrane Database of Systematic Reviews, *(4).
- Barlam, T. F., Cosgrove, S. E., Abbo, L. M., MacMillan, K. L., Sinkowitz-Cochran, R. L., Chambers, J. S., … & Wright, M. O. (2016). Implementing an antibiotic stewardship program: guidelines by the Infectious Diseases Society of America and the Society for Healthcare Epidemiology of America. Clinical infectious diseases, 62(10), e51-e77.
- Goff, D. A., Kullar, R., Goldstein, E. J., Gilchrist, M., Zilberberg, M. D., & Nathan, C. (2017). Antimicrobial stewardship interventions: a systematic review. Infection control & hospital epidemiology, 38(4), 372-385.
- Landers, T. F., Linkin, D. R., & Sawyer, R. G. (2012). Content and outcomes of antimicrobial stewardship programs: a survey of US hospitals. American journal of health-system pharmacy, 69(10), 843-848.
- Sartelli, M., Viale, P., Kočova, E., Tumietto, F., Ansaloni, L., Moore, E. E., … & Catena, F. (2011). WSES guidelines for management of skin and soft tissue infections. World journal of emergency surgery, 6(1), 13.
- Loveday, H. P., Wilson, J. A., Pratt, R. J., Golsorkhi, M., Tingle, A., Bak, A., … & Wilcox, M. (2014). epic3: national evidence-based guidelines for preventing healthcare-associated infections in NHS hospitals in England. Journal of Hospital Infection, 86, S1-S70.
- Clardy, J., Fischbach, M. A., Currie, C. R., & Walsh, C. T. (2006). The natural products pipeline. Chemistry & biology, 13(7), 689-695.
- Silver, L. L. (2011). Challenges of antibacterial discovery. Clinical microbiology reviews, 24(1), 71-109.
- Fischbach, M. A., & Walsh, C. T. (2009). Antibiotics for emerging pathogens. Science, 325(5944), 1089-1093.
- Rohde, S. H., Cleto, K., Krylov, V. N., & Plagens, A. (2018). Structure and activity of bacteriophages for therapy. Antibiotics, 7(4), 113.
- Chan, B. K., Abedon, S. T., & Loc-Carrillo, C. (2013). Phage therapy. Bacteriophage, 3(2), e26366.
- Morens, D. M., Folkers, G. K., & Fauci, A. S. (2008). Emerging infections: a perpetual challenge. The Lancet infectious diseases, 8(11), 710-719.
- Bloom, B. R. (2006). Tuberculosis: a disease of the past?. The Journal of clinical investigation, 116(8), 1999-2007.
- Boucher, H. W., Talbot, G. H., Bradley, J. S., Edwards, J. E., Gilbert, D., Rice, L. B., … & Bartlett, J. G. (2009). Bad bugs, no drugs: no ESKAPE!. An update from the Infectious Diseases Society of America. Clinical Infectious Diseases, 48(1), 1-12.
Be the first to comment