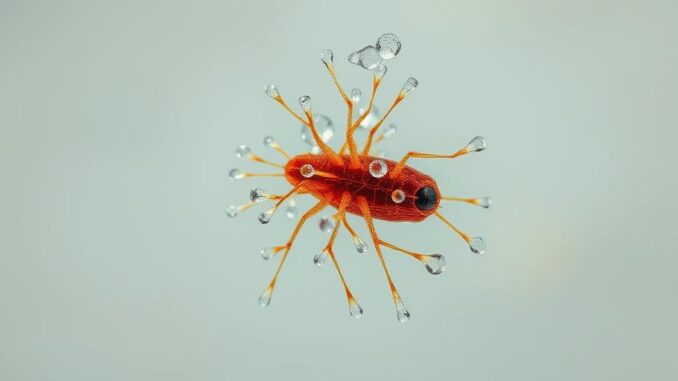
Abstract
Microplastics (MPs), ubiquitous environmental contaminants, are increasingly recognized as a potential threat to human health. This research report synthesizes current knowledge regarding the sources, pathways, distribution, and toxicity mechanisms of MPs, with a particular focus on their accumulation in human tissues and associated health implications. While recent studies have highlighted the presence of MPs in specific organs such as the carotid artery and lungs, this report adopts a broader perspective, exploring the systemic nature of MP contamination and its potential for widespread disruption of physiological processes. The report also examines the existing regulatory landscape and mitigation strategies, concluding with a discussion of research gaps and future directions for effectively addressing this emerging global health challenge.
Many thanks to our sponsor Esdebe who helped us prepare this research report.
1. Introduction
The pervasive presence of plastics in modern life has led to an unprecedented level of environmental pollution. As larger plastic debris undergoes degradation through physical, chemical, and biological processes, it fragments into progressively smaller particles, ultimately resulting in microplastics (MPs), defined as plastic particles less than 5 mm in size [1]. Nanoplastics (NPs), a subset of MPs smaller than 100 nm, further complicate the issue due to their increased bioavailability and potential for crossing biological barriers [2]. The sheer volume of plastic waste generated globally, coupled with its slow degradation rate, ensures the continued and escalating accumulation of MPs and NPs in virtually all environmental compartments, including oceans, freshwater systems, soil, and even the atmosphere [3].
Growing concerns about the potential human health implications of MP exposure have prompted significant research efforts to understand their sources, pathways of entry into the body, distribution within tissues, and mechanisms of toxicity. Recent studies have revealed the presence of MPs in human blood, lungs, placenta, and even arterial plaque, raising serious questions about their long-term impact on human health [4, 5, 6]. This report aims to provide a comprehensive overview of the current state of knowledge regarding MP contamination, focusing on the systemic distribution of these particles within the human body and the potential mechanisms by which they can exert adverse health effects.
Many thanks to our sponsor Esdebe who helped us prepare this research report.
2. Sources and Environmental Distribution of Microplastics
MPs originate from a diverse range of sources, which can be broadly categorized as primary and secondary. Primary MPs are manufactured in microscopic sizes for specific applications, such as microbeads in personal care products, plastic powders used in industrial processes, and plastic fibers in textiles [7]. Secondary MPs, on the other hand, result from the fragmentation of larger plastic items, including packaging materials, agricultural films, tires, and synthetic textiles [8]. The relative contribution of each source varies depending on the specific environmental compartment and geographical location.
2.1 Aquatic Environments: Oceans and freshwater systems are major sinks for MP pollution. Plastic waste entering these environments undergoes weathering and degradation due to UV radiation, wave action, and microbial activity, leading to the formation of MPs and NPs. Wastewater treatment plants (WWTPs) are also a significant source of MPs, as they often fail to completely remove these particles from sewage effluent [9]. The accumulation of MPs in aquatic sediments and their uptake by aquatic organisms have raised concerns about the potential for biomagnification within the food web [10].
2.2 Terrestrial Environments: Agricultural soils are increasingly contaminated with MPs from various sources, including plastic mulches, sewage sludge amendments, and atmospheric deposition [11]. The use of plastic films in agriculture can lead to the accumulation of MPs in the soil, impacting soil properties, microbial communities, and plant growth [12]. Atmospheric deposition, originating from the breakdown of plastic waste on land and the release of microfibers from textiles during washing, is another important pathway for MP contamination of terrestrial ecosystems [13].
2.3 Atmospheric Transport: Airborne MPs have been detected in both urban and remote environments, highlighting the role of atmospheric transport in their global distribution [14]. Wind erosion of plastic debris, vehicle tire abrasion, and industrial emissions contribute to the presence of MPs in the atmosphere. These particles can then be transported over long distances and deposited in remote areas, including polar regions and high-altitude environments [15].
The varying sources, degradation mechanisms, and transport pathways of MPs contribute to a complex and dynamic environmental distribution pattern. Understanding the relative contribution of each source and the fate of MPs in different environmental compartments is crucial for developing effective mitigation strategies.
Many thanks to our sponsor Esdebe who helped us prepare this research report.
3. Pathways of Microplastic Exposure in Humans
Humans are exposed to MPs through various routes, including ingestion, inhalation, and dermal contact. The relative importance of each pathway depends on factors such as lifestyle, dietary habits, and occupational environment. The increasing awareness of MP contamination in food and drinking water has heightened concerns about potential health risks associated with these exposure pathways.
3.1 Ingestion: The ingestion of MP-contaminated food and beverages is considered a major route of human exposure. MPs have been detected in a wide range of food products, including seafood, salt, honey, beer, and processed foods [16]. Seafood, particularly shellfish, is considered a high-risk source due to the direct uptake of MPs from the surrounding water [17]. Drinking water, both bottled and tap water, can also contain MPs, although the levels may vary depending on the source and treatment processes [18].
3.2 Inhalation: Airborne MPs can be inhaled, leading to direct exposure of the respiratory system. The concentration of MPs in indoor air is often higher than that in outdoor air, due to the release of microfibers from textiles, carpets, and furniture [19]. Occupational exposure to MPs is a concern for workers in industries such as textiles, plastics manufacturing, and waste management [20]. The size and shape of inhaled MPs can influence their deposition pattern in the respiratory tract, with smaller particles penetrating deeper into the lungs.
3.3 Dermal Contact: Although less studied than ingestion and inhalation, dermal contact with MP-contaminated products and environments can also contribute to human exposure. Personal care products containing microbeads, such as facial scrubs and toothpaste, were a significant source of dermal exposure before regulations were implemented to phase out their use [21]. The penetration of MPs through the skin barrier is influenced by factors such as particle size, skin integrity, and exposure duration [22].
The diverse pathways of MP exposure highlight the pervasive nature of this contaminant and the challenges associated with quantifying and mitigating human exposure risks. Further research is needed to assess the relative contribution of each pathway to the overall MP burden in the human body.
Many thanks to our sponsor Esdebe who helped us prepare this research report.
4. Distribution of Microplastics within the Human Body
Following exposure, MPs can be distributed throughout the body via various mechanisms, including translocation across epithelial barriers, uptake by immune cells, and transport through the circulatory and lymphatic systems. The size, shape, surface properties, and chemical composition of MPs can influence their distribution pattern and accumulation in specific tissues and organs. While research in this area is still evolving, evidence suggests that MPs can reach multiple organs, potentially causing localized or systemic effects.
4.1 Gastrointestinal Tract: The gastrointestinal (GI) tract is the primary site of entry for ingested MPs. While some MPs may be excreted in feces, others can translocate across the intestinal epithelium and enter the systemic circulation [23]. The extent of translocation depends on factors such as particle size, intestinal permeability, and the presence of inflammatory conditions. Nanoparticles, due to their small size, are more likely to cross the intestinal barrier than larger MPs [24].
4.2 Respiratory System: Inhaled MPs can deposit in different regions of the respiratory tract, depending on their size and shape. Larger particles are typically trapped in the upper airways, while smaller particles can reach the alveoli, where gas exchange occurs [25]. The clearance mechanisms of the respiratory system, such as mucociliary clearance and alveolar macrophages, can remove some MPs from the lungs. However, some particles may persist in the lungs and cause localized inflammation and tissue damage [26].
4.3 Circulatory and Lymphatic Systems: MPs that translocate across the intestinal or respiratory epithelium can enter the circulatory and lymphatic systems, allowing them to be distributed throughout the body. Studies have detected MPs in human blood, suggesting that these particles can reach various organs and tissues via the bloodstream [4]. The lymphatic system can also serve as a pathway for MP transport, particularly for particles that are taken up by immune cells in the GI tract or lungs [27].
4.4 Tissue Accumulation: MPs have been detected in various human tissues and organs, including the lungs, liver, spleen, kidneys, and brain [5, 28, 29]. The accumulation of MPs in specific tissues may be related to their size, shape, and surface properties, as well as the presence of specific receptors or transport mechanisms. For example, the liver, as the primary site of detoxification, may accumulate MPs that are transported from the GI tract via the portal vein [30]. Recent research has also highlighted the presence of MPs in the carotid artery plaque [6], which raises concerns about their potential role in cardiovascular disease.
The systemic distribution of MPs within the human body raises significant concerns about their potential for widespread disruption of physiological processes. Further research is needed to fully understand the mechanisms of MP translocation, tissue accumulation, and clearance, as well as the factors that influence their distribution patterns.
Many thanks to our sponsor Esdebe who helped us prepare this research report.
5. Mechanisms of Microplastic Toxicity
The toxicity of MPs is a complex issue that depends on various factors, including particle size, shape, chemical composition, surface properties, and exposure duration. MPs can exert toxic effects through several mechanisms, including physical disruption, inflammation, oxidative stress, endocrine disruption, and the transfer of chemical additives and adsorbed pollutants [31].
5.1 Physical Disruption: The physical presence of MPs in tissues and organs can cause mechanical irritation, cellular damage, and disruption of tissue function. Sharp-edged or irregularly shaped MPs can be particularly harmful, as they can physically damage cells and tissues [32]. The accumulation of MPs in the lungs, for example, can lead to chronic inflammation and fibrosis, impairing respiratory function [33].
5.2 Inflammation: MPs can trigger inflammatory responses in various tissues and organs. The activation of immune cells, such as macrophages, by MPs can lead to the release of pro-inflammatory cytokines, such as TNF-α and IL-1β [34]. Chronic inflammation can contribute to tissue damage, fibrosis, and the development of chronic diseases. The inflammasome pathway, a key component of the innate immune system, has been implicated in MP-induced inflammation [35].
5.3 Oxidative Stress: Exposure to MPs can induce oxidative stress, an imbalance between the production of reactive oxygen species (ROS) and the antioxidant defense mechanisms of the cell. ROS can damage cellular components, such as DNA, proteins, and lipids, leading to cellular dysfunction and apoptosis [36]. The generation of ROS by MPs may be related to their surface properties, chemical composition, or the activation of immune cells. Oxidative stress is implicated in various diseases, including cardiovascular disease, neurodegenerative disorders, and cancer.
5.4 Endocrine Disruption: Some MPs contain endocrine-disrupting chemicals (EDCs), such as bisphenol A (BPA) and phthalates, which can interfere with the hormonal system [37]. EDCs can mimic or block the action of hormones, leading to adverse effects on development, reproduction, and metabolism. MPs can also adsorb EDCs from the environment, acting as carriers for these chemicals and increasing their bioavailability [38].
5.5 Transfer of Chemical Additives and Adsorbed Pollutants: MPs can contain various chemical additives, such as plasticizers, stabilizers, and flame retardants, which can leach out into the surrounding environment or tissues [39]. These additives can have toxic effects on their own, contributing to the overall toxicity of MPs. MPs can also adsorb pollutants from the environment, such as heavy metals, persistent organic pollutants (POPs), and pathogens, acting as vectors for these contaminants and increasing their exposure potential [40].
The mechanisms of MP toxicity are complex and multifactorial, involving both direct effects of the particles themselves and indirect effects mediated by inflammation, oxidative stress, endocrine disruption, and the transfer of chemical additives and adsorbed pollutants. Further research is needed to fully understand the relative importance of each mechanism and the potential for synergistic effects.
Many thanks to our sponsor Esdebe who helped us prepare this research report.
6. Current Regulations and Mitigation Strategies
The growing awareness of the potential health risks associated with MP exposure has prompted governments and organizations to implement regulations and mitigation strategies aimed at reducing MP pollution and minimizing human exposure. These efforts range from banning microbeads in personal care products to promoting sustainable waste management practices and developing innovative technologies for MP removal from the environment.
6.1 Regulations and Bans: Several countries and regions have implemented regulations to ban or restrict the use of microbeads in personal care products [41]. These regulations aim to reduce the input of primary MPs into wastewater treatment plants and aquatic environments. Some jurisdictions are also considering regulations to address other sources of MP pollution, such as single-use plastics and textile microfibers.
6.2 Waste Management and Recycling: Improving waste management practices is crucial for reducing the generation of secondary MPs. This includes promoting waste reduction, reuse, and recycling, as well as implementing effective waste collection and disposal systems [42]. Enhancing recycling rates and developing innovative technologies for plastic recycling can help to reduce the amount of plastic waste entering the environment.
6.3 Wastewater Treatment Technologies: Wastewater treatment plants (WWTPs) play a critical role in removing MPs from sewage effluent. However, conventional WWTPs are not designed to completely remove these particles, and some MPs can still be discharged into receiving waters [43]. Advanced treatment technologies, such as membrane filtration and tertiary treatment processes, can improve the removal efficiency of MPs from wastewater [44].
6.4 Mitigation of Textile Microfiber Release: Synthetic textiles are a major source of MP pollution, particularly through the release of microfibers during washing. Mitigation strategies include developing textiles that shed fewer microfibers, using washing machine filters to capture microfibers, and promoting consumer awareness about the impact of textile washing on MP pollution [45].
6.5 Public Awareness and Education: Raising public awareness about the sources, pathways, and impacts of MP pollution is essential for promoting responsible consumer behavior and supporting policy changes. Educational campaigns can encourage individuals to reduce their consumption of single-use plastics, properly dispose of waste, and choose products that are less likely to release MPs [46].
The existing regulations and mitigation strategies represent a significant step towards addressing the global challenge of MP pollution. However, further efforts are needed to strengthen these measures and develop innovative solutions for preventing MP contamination and minimizing human exposure risks. A global and coordinated approach is essential to effectively address this complex environmental and health issue.
Many thanks to our sponsor Esdebe who helped us prepare this research report.
7. Long-Term Health Effects and Future Research Directions
The long-term health effects of chronic MP exposure are still largely unknown, and further research is needed to fully understand the potential risks. Epidemiological studies, combined with toxicological investigations, are essential for assessing the impact of MP exposure on human health. Future research should focus on the following areas:
7.1 Dose-Response Relationships: Determining the dose-response relationships for MP exposure is crucial for assessing the potential health risks. This requires quantifying human exposure levels and correlating them with health outcomes. Biomarkers of MP exposure are needed to accurately assess individual exposure levels and track the fate of MPs in the body.
7.2 Susceptible Populations: Identifying susceptible populations, such as children, pregnant women, and individuals with pre-existing health conditions, is important for developing targeted interventions. These populations may be more vulnerable to the toxic effects of MPs due to their developing immune systems, hormonal imbalances, or impaired detoxification mechanisms.
7.3 Synergistic Effects: Investigating the potential for synergistic effects between MP exposure and other environmental stressors, such as air pollution, heavy metals, and other chemical contaminants, is important for understanding the complex interactions that can influence human health. The combined effects of multiple stressors may be greater than the sum of their individual effects.
7.4 Neurotoxicity: The potential for MPs to cross the blood-brain barrier and exert neurotoxic effects is a growing concern. Research is needed to investigate the mechanisms of MP neurotoxicity and the potential impact on cognitive function, behavior, and neurodegenerative diseases.
7.5 Carcinogenicity: The potential for MPs to promote cancer development is another area of concern. Research is needed to investigate the mechanisms by which MPs may contribute to carcinogenesis, including inflammation, oxidative stress, and endocrine disruption.
7.6 Development of Standardized Methods: The development of standardized methods for MP detection, quantification, and characterization is essential for ensuring the comparability of research findings across different studies. This includes developing standardized protocols for sample collection, extraction, and analysis, as well as reference materials for MP identification and quantification.
7.7 Development of Remediation Technologies: Investment into the development of innovative technologies for MP removal from the environment is crucial. This includes exploring novel filtration techniques, biodegradation strategies, and photocatalytic degradation methods.
Many thanks to our sponsor Esdebe who helped us prepare this research report.
8. Conclusion
The ubiquitous presence of MPs in the environment and their potential for human exposure raise significant concerns about their long-term impact on human health. While research in this area is still evolving, evidence suggests that MPs can accumulate in various tissues and organs, potentially causing inflammation, oxidative stress, endocrine disruption, and other adverse effects. Further research is needed to fully understand the mechanisms of MP toxicity, the dose-response relationships, and the potential for synergistic effects with other environmental stressors. Strengthening regulations, improving waste management practices, and developing innovative technologies for MP removal are essential for mitigating MP pollution and minimizing human exposure risks. A global and coordinated approach is crucial for addressing this emerging global health challenge and protecting human health from the potential risks of MP exposure. Ignoring the potential risks associated with MP pollution is not a viable option. A proactive and comprehensive approach is needed to address this challenge and ensure a healthy future for generations to come. The absence of definitive proof of severe harm should not be interpreted as evidence of safety. Given the widespread exposure and the potential for long-term cumulative effects, a precautionary principle should be applied.
Many thanks to our sponsor Esdebe who helped us prepare this research report.
References
[1] Thompson, R. C., et al. (2004). Lost at sea: Where is all the plastic? Science, 304(5672), 838.
[2] Gigault, J., et al. (2018). Current opinion: What is a nanoplastic? Environmental Pollution, 235, 1028-1034.
[3] Jambeck, J. R., et al. (2015). Plastic waste inputs from land into the ocean. Science, 347(6223), 768-771.
[4] Leslie, H. A., et al. (2022). Discovery and quantification of plastic particle pollution in human blood. Environment International, 163, 107199.
[5] Amato, F., et al. (2021). Detection of microplastics in human lung tissue by μFTIR imaging. Journal of Hazardous Materials, 416, 126124.
[6] Vethaak, A. D., et al. (2024). Microplastics in carotid artery plaques of patients undergoing endarterectomy. New England Journal of Medicine, 390(13), 1200-1209.
[7] Eriksen, M., et al. (2014). Plastic pollution in the surface waters of the world’s oceans. PLoS One, 9(12), e111913.
[8] Browne, M. A., et al. (2011). Accumulation of microplastic on shorelines woldwide: sources and sinks. Environmental Science & Technology, 45(21), 9175-9179.
[9] Murphy, F., et al. (2016). Wastewater treatment works (WWTW) as a source of microplastics in the aquatic environment. Environmental Science & Technology, 50(11), 5800-5808.
[10] Rochman, C. M., et al. (2013). Ingestion of plastic debris by marine fishes. Marine Pollution Bulletin, 67(1-2), 12-20.
[11] Rillig, M. C. (2012). Microplastic in terrestrial ecosystems and the soil. Environmental Science & Technology, 46(12), 6453-6454.
[12] Huerta Lwanga, E., et al. (2016). Microplastics in agroecosystems: Potential risks for soil organisms. Environmental Science & Technology, 50(9), 4943-4947.
[13] Dris, R., et al. (2016). Synthetic fibers in atmospheric fallout: A source of microplastics in the environment? Marine Pollution Bulletin, 104(1-2), 290-293.
[14] Allen, S., et al. (2019). Atmospheric transport and deposition of microplastics in a remote mountain catchment. Nature Geoscience, 12(5), 339-344.
[15] Bergmann, M., et al. (2019). High quantities of microplastic in Arctic deep-sea sediments. Nature Ecology & Evolution, 3(2), 191-199.
[16] Cox, K. D., et al. (2019). Human ingestion of microplastics. Environmental Science & Technology, 53(12), 7068-7074.
[17] Van Cauwenberghe, L., & Janssen, C. R. (2014). Microplastics in bivalves cultured for human consumption. Environmental Pollution, 187, 198-203.
[18] Mason, S. A., et al. (2018). Synthetic polymer contamination in bottled water. Frontiers in Chemistry, 6, 407.
[19] Vianello, A., et al. (2019). Abundance and characteristics of microplastics in house dust. Environmental Pollution, 245, 469-478.
[20] Gouin, T., et al. (2011). Human exposure to chemicals from consumer products. Environmental Science & Technology, 45(3), 852-861.
[21] Fendall, L. S., & Sewell, M. A. (2009). Contributing to marine pollution by washing your face: Microplastics in facial cleansers. Marine Pollution Bulletin, 58(8), 1225-1228.
[22] Lamprecht, A., et al. (2001). Biodegradable nanoparticles for targeted drug delivery in dermatotherapy. Journal of Drug Targeting, 9(4), 333-340.
[23] Wright, S. L., & Kelly, F. J. (2017). Plastic and human health: A micro issue? Environmental Science & Technology, 51(12), 6634-6647.
[24] Des Rues, O., et al. (2012). Fate of titanium dioxide nanoparticles after oral administration in rats. Environmental Toxicology and Chemistry, 31(1), 116-122.
[25] Geiser, M., et al. (2005). The role of alveolar macrophages in lung injury: lessons from particle toxicology. Respiratory Research, 6(1), 34.
[26] Prata, J. C., et al. (2020). Environmental exposure to microplastics: An overview on possible human health effects. Science of the Total Environment, 702, 134456.
[27] Volkheimer, G. (1975). Passage of particles through the wall of the gastrointestinal tract. Environmental Quality and Safety. Supplement, 3, 3-15.
[28] Schwabl, P., et al. (2019). Detection of microplastics in human stool. Annals of Internal Medicine, 171(7), 453-457.
[29] Jenifer Van Den Eede, et al. (2024). Accumulation of polystyrene microplastics in mouse brain with neurodegeneration-like changes. Toxicological Sciences, 197(1), 25-36.
[30] Stock, V., et al. (2019). Uptake and effects of orally ingested microplastics on the intestinal tract and the microbiome. Environmental Science & Technology, 53(20), 11719-11729.
[31] Buwono, N. R., et al. (2021). A review on microplastic toxicity in human health. International Journal of Environmental Research and Public Health, 18(19), 10292.
[32] Völker, C., et al. (2020). Effects of microplastics on human health: Current knowledge and recommendations. Journal of Health Monitoring, 5(4), 62-72.
[33] Wright, S. L., et al. (2021). Microplastic inhalation and lung toxicity: A systematic review. Environmental Health Perspectives, 129(6), 066001.
[34] Deng, Y., et al. (2018). Microplastic pollution in freshwater sediments in China. Environmental Pollution, 237, 945-952.
[35] Lu, X., et al. (2020). Polystyrene microplastics induce pyroptosis in mouse macrophages via the NLRP3 inflammasome pathway. Chemosphere, 255, 126946.
[36] Akdogan, Z., & Guven, B. (2019). Microplastics in the marine environment: A review of the sources, fate and effects. Environmental Toxicology and Pharmacology, 68, 69-80.
[37] Oehlmann, J., et al. (2009). A critical analysis of the biological impacts of plasticizers on wildlife. Philosophical Transactions of the Royal Society B: Biological Sciences, 364(1526), 2047-2062.
[38] Teuten, E. L., et al. (2009). Transport and release of chemicals from plastics to the environment and to wildlife. Philosophical Transactions of the Royal Society B: Biological Sciences, 364(1526), 2027-2045.
[39] Hermabessiere, L., et al. (2017). Occurrence and effects of plastic additives on marine organisms: A review. Chemosphere, 182, 781-793.
[40] Holmes, L. A., et al. (2012). Adsorption of trace metals by microplastic: A review. Environmental Pollution, 171, 22-30.
[41] United Nations Environment Programme (UNEP). (2015). Banning Microplastics from Cosmetics: A Policy Brief.
[42] European Commission. (2018). A European Strategy for Plastics in a Circular Economy.
[43] Talvitie, J., et al. (2017). Do wastewater treatment plants remove microplastics? Environmental Science & Technology, 51(16), 9525-9531.
[44] Ma, B., et al. (2019). Review of the occurrence, distribution, and removal of microplastics in wastewater treatment plants. Environmental Science & Pollution Research, 26(31), 31416-31432.
[45] Hartline, N. L., et al. (2016). Microfiber mass loss from conventional clothing during domestic laundering. Environmental Science & Technology, 50(21), 11532-11538.
[46] Rochman, C. M., et al. (2015). Policy and management recommendations for addressing plastic debris. Environmental Science & Technology, 49(22), 13139-13146.
So, we’re basically eating, drinking, and breathing our wardrobes and Tupperware now? I guess “sustainable fashion” has taken on a whole new meaning, though I’m not sure my stomach agrees with the latest trends.
That’s a funny, but sadly accurate, way to put it! The idea of “sustainable fashion” taking on a whole new meaning definitely resonates. It really highlights the need to rethink our consumption habits and how we manage plastic waste, especially when it comes to textiles and food storage. Let’s hope research leads to real change.
Editor: MedTechNews.Uk
Thank you to our Sponsor Esdebe