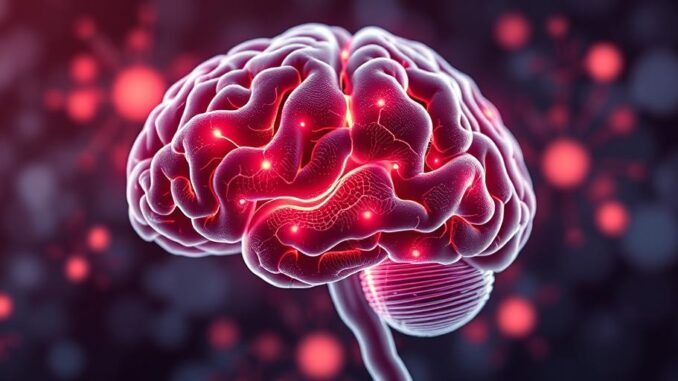
The Symphony of Signaling: A Comprehensive Exploration of Neuronal Networks in Metabolic Regulation
Many thanks to our sponsor Esdebe who helped us prepare this research report.
Abstract
This research report provides a comprehensive overview of the intricate role of neuronal networks in metabolic regulation, extending beyond the well-established function of AgRP neurons in blood glucose control. The report delves into the diverse populations of neurons within the hypothalamus and other brain regions that orchestrate energy homeostasis, glucose metabolism, and insulin sensitivity. We examine the specific molecular mechanisms through which these neuronal circuits exert their influence, encompassing neurotransmitter signaling, receptor dynamics, and intracellular signaling cascades. Furthermore, we explore the challenges and potential therapeutic avenues for targeting these neuronal networks in metabolic disorders such as obesity and type 2 diabetes, while also addressing the ethical considerations associated with manipulating brain activity for metabolic benefit. Finally, we discuss limitations of current research and future directions to advance the understanding of this complex system.
Many thanks to our sponsor Esdebe who helped us prepare this research report.
1. Introduction
Metabolic regulation is a complex and multifaceted process essential for maintaining energy balance and ensuring the proper functioning of physiological systems. While peripheral tissues and endocrine organs play crucial roles, the central nervous system (CNS), particularly the hypothalamus, emerges as a central command center in orchestrating metabolic homeostasis [1]. Within the hypothalamus, specialized neuronal populations integrate peripheral signals, such as hormones and nutrients, to regulate food intake, energy expenditure, and glucose metabolism. This report aims to provide a comprehensive overview of the key neuronal networks involved in metabolic control, focusing on their specific functions, underlying mechanisms, and therapeutic potential. Beyond the well-studied AgRP neurons, we will delve into the diverse range of neuronal populations that contribute to the intricate symphony of metabolic regulation. We aim to provide a deeper understanding of the complex interplay between neuronal signaling and metabolic outcomes, emphasizing the potential for novel therapeutic interventions while acknowledging the ethical complexities involved.
Many thanks to our sponsor Esdebe who helped us prepare this research report.
2. Hypothalamic Neuronal Circuits in Metabolic Control
The hypothalamus, a small but critical brain region, serves as a primary regulator of energy balance. Within the hypothalamus, several distinct nuclei contain specialized neuronal populations that sense metabolic cues and orchestrate appropriate responses. These nuclei include the arcuate nucleus (ARC), ventromedial hypothalamus (VMH), dorsomedial hypothalamus (DMH), and lateral hypothalamus (LH) [2].
2.1 The Arcuate Nucleus (ARC)
The ARC, strategically located near the median eminence, is a primary interface between the brain and the periphery. It contains two major neuronal populations with opposing effects on energy balance: anorexigenic proopiomelanocortin (POMC) neurons and orexigenic Agouti-related peptide (AgRP) neurons [3]. POMC neurons release α-melanocyte-stimulating hormone (α-MSH), which binds to melanocortin-4 receptors (MC4Rs) in downstream brain regions, such as the paraventricular nucleus (PVN), to suppress appetite and increase energy expenditure. Conversely, AgRP neurons co-express AgRP and neuropeptide Y (NPY), which antagonize MC4Rs and stimulate food intake, respectively. Both POMC and AgRP neurons are regulated by peripheral hormones, such as leptin and insulin, which act as signals of energy status. Leptin and insulin activate POMC neurons and inhibit AgRP neurons, thereby promoting energy expenditure and reducing food intake [4]. Perturbations in the signaling of either of these neurons can lead to metabolic dysfunction, such as obesity and insulin resistance.
2.2 The Ventromedial Hypothalamus (VMH)
The VMH is another crucial hypothalamic nucleus involved in regulating energy expenditure and glucose metabolism. Neurons in the VMH are sensitive to glucose and fatty acids, and their activation promotes satiety and increases energy expenditure [5]. The VMH also plays a critical role in regulating hepatic glucose production and insulin sensitivity. Lesions of the VMH result in hyperphagia, obesity, and insulin resistance, highlighting its importance in metabolic control. Recent studies have identified specific neuronal subtypes within the VMH that mediate distinct metabolic functions, such as the regulation of glucose homeostasis and the modulation of physical activity [6].
2.3 The Dorsomedial Hypothalamus (DMH)
The DMH is implicated in the regulation of circadian rhythms, stress responses, and energy balance. DMH neurons receive inputs from various brain regions and project to other hypothalamic nuclei, including the PVN and LH [7]. Activation of DMH neurons increases sympathetic nervous system activity and promotes energy expenditure. The DMH also plays a role in regulating glucose metabolism, particularly during stress. Chronic stress can disrupt DMH function, leading to metabolic dysregulation and increased risk of metabolic disorders.
2.4 The Lateral Hypothalamus (LH)
The LH is primarily known for its role in promoting food intake and arousal. LH neurons produce orexin and melanin-concentrating hormone (MCH), which stimulate appetite and reduce energy expenditure [8]. Orexin neurons also play a crucial role in regulating wakefulness and motivation. The LH receives inputs from various brain regions, including the ARC, VMH, and DMH, and integrates these signals to regulate feeding behavior. While traditionally viewed as a “hunger center”, the LH’s function is more nuanced, influencing motivation and reward aspects of feeding beyond purely homeostatic drive.
Many thanks to our sponsor Esdebe who helped us prepare this research report.
3. Beyond the Hypothalamus: Extra-Hypothalamic Neuronal Networks
While the hypothalamus is a primary regulator of metabolic control, extra-hypothalamic brain regions also play significant roles in modulating energy balance and glucose metabolism. These regions include the brainstem, amygdala, and reward circuitry [9].
3.1 The Brainstem
The brainstem, particularly the nucleus of the solitary tract (NTS) and the area postrema (AP), receives direct sensory information from the gut and plays a crucial role in regulating food intake and satiety. These regions are sensitive to gut hormones, such as cholecystokinin (CCK) and peptide YY (PYY), which signal satiety and reduce food intake [10]. The brainstem also contains neurons that project to the hypothalamus and influence hypothalamic neuronal activity.
3.2 The Amygdala
The amygdala, involved in emotional processing and stress responses, also contributes to metabolic regulation. The amygdala receives inputs from the hypothalamus and brainstem and projects to other brain regions involved in energy balance. Stress and emotional states can influence food intake and energy expenditure through amygdala-mediated mechanisms. Chronic stress can lead to increased food intake and weight gain, partly due to amygdala dysfunction [11].
3.3 Reward Circuitry
The mesolimbic dopamine system, including the ventral tegmental area (VTA) and the nucleus accumbens (NAc), plays a crucial role in regulating reward-related behaviors, including food intake. Palatable foods activate the dopamine system, leading to increased motivation to consume them. Overactivation of the reward system by highly palatable foods can override homeostatic signals and contribute to overeating and obesity [12]. Dysregulation of dopamine signaling is implicated in compulsive eating behaviors.
Many thanks to our sponsor Esdebe who helped us prepare this research report.
4. Molecular Mechanisms Underlying Neuronal Control of Metabolism
Neuronal circuits regulate metabolic processes through a complex interplay of neurotransmitters, receptors, and intracellular signaling pathways. Understanding these molecular mechanisms is crucial for developing targeted therapies for metabolic disorders.
4.1 Neurotransmitter Signaling
Neurons communicate with each other through the release of neurotransmitters, which bind to specific receptors on target neurons. Several neurotransmitters are involved in regulating energy balance, including glutamate, GABA, dopamine, serotonin, and neuropeptides. Glutamate and GABA are the primary excitatory and inhibitory neurotransmitters in the brain, respectively. Dopamine and serotonin modulate reward-related behaviors and mood, influencing food intake and energy expenditure [13]. Neuropeptides, such as NPY, AgRP, orexin, and MCH, play specialized roles in regulating appetite, satiety, and energy expenditure.
4.2 Receptor Dynamics
The effects of neurotransmitters depend on the expression, localization, and signaling properties of their respective receptors. For example, MC4Rs, the primary targets of α-MSH, are G protein-coupled receptors (GPCRs) that activate intracellular signaling cascades, leading to decreased food intake and increased energy expenditure. Dysfunctional MC4R signaling is associated with obesity. Similarly, orexin receptors, OX1R and OX2R, mediate the effects of orexin on arousal and food intake. Targeting these receptors with pharmacological agents has shown promise in treating sleep disorders and obesity [14].
4.3 Intracellular Signaling Cascades
Neurotransmitter receptor activation triggers intracellular signaling cascades that ultimately regulate gene expression and neuronal activity. Key signaling pathways involved in metabolic control include the cAMP/PKA pathway, the MAPK pathway, and the PI3K/Akt pathway. These pathways regulate the expression of genes involved in energy metabolism, glucose metabolism, and neuronal function. Dysregulation of these signaling pathways can contribute to metabolic disorders [15].
Many thanks to our sponsor Esdebe who helped us prepare this research report.
5. Therapeutic Targeting of Neuronal Circuits in Metabolic Disorders
The growing understanding of neuronal networks in metabolic regulation has opened up new avenues for therapeutic intervention in metabolic disorders such as obesity and type 2 diabetes. However, targeting these neuronal circuits therapeutically presents significant challenges, including the blood-brain barrier, the complexity of neuronal networks, and the potential for off-target effects.
5.1 Pharmacological Approaches
Pharmacological agents that target specific neurotransmitter receptors or signaling pathways involved in metabolic control have shown promise in treating obesity and type 2 diabetes. For example, lorcaserin, a selective serotonin 2C receptor (5-HT2C) agonist, promotes satiety and reduces food intake. Naltrexone/bupropion, a combination of an opioid antagonist and a dopamine/norepinephrine reuptake inhibitor, targets reward pathways to reduce food cravings and promote weight loss [16]. However, these drugs often have limited efficacy and can cause side effects.
5.2 Gene Therapy and Optogenetics
Gene therapy and optogenetics offer more targeted approaches to manipulate neuronal activity in specific brain regions. Gene therapy involves delivering genes that encode for specific proteins into target neurons, altering their function. Optogenetics involves using light-sensitive proteins to control neuronal activity with light. These techniques have shown promise in preclinical studies for treating obesity and type 2 diabetes [17]. However, gene therapy and optogenetics are still in their early stages of development and face challenges related to safety, efficacy, and delivery.
5.3 Ethical Considerations
The manipulation of brain activity for metabolic benefit raises significant ethical considerations. The potential for unintended consequences, off-target effects, and the risk of altering personality and behavior must be carefully considered. It is crucial to ensure that any therapeutic interventions are safe, effective, and ethically sound. Furthermore, the potential for social inequalities in access to these therapies must be addressed. The responsible development and implementation of neuronal-targeted therapies require careful ethical oversight and public engagement.
Many thanks to our sponsor Esdebe who helped us prepare this research report.
6. Limitations and Future Directions
Despite significant advances in understanding the role of neuronal networks in metabolic regulation, several limitations remain. The complexity of neuronal circuits, the heterogeneity of neuronal populations, and the lack of precise tools to manipulate neuronal activity in vivo pose significant challenges. Future research should focus on:
- Developing more sophisticated tools to map and manipulate neuronal circuits with greater precision.
- Identifying novel neuronal subtypes and their specific functions in metabolic control.
- Elucidating the molecular mechanisms underlying neuronal plasticity and adaptation in response to metabolic challenges.
- Developing more targeted and effective therapies for metabolic disorders based on neuronal mechanisms.
- Conducting long-term studies to assess the safety and efficacy of neuronal-targeted therapies.
Furthermore, there is a need for increased focus on the impact of environmental factors, such as diet and stress, on neuronal function and metabolic regulation. Longitudinal studies are needed to understand how these factors contribute to the development of metabolic disorders over time. Finally, interdisciplinary collaborations between neuroscientists, endocrinologists, and clinicians are essential for translating basic research findings into clinically relevant therapies.
Many thanks to our sponsor Esdebe who helped us prepare this research report.
7. Conclusion
The intricate interplay between neuronal networks and metabolic regulation is crucial for maintaining energy balance and overall health. This report has highlighted the diverse neuronal populations within the hypothalamus and other brain regions that orchestrate metabolic homeostasis, glucose metabolism, and insulin sensitivity. Understanding the specific molecular mechanisms through which these neuronal circuits exert their influence is essential for developing targeted therapies for metabolic disorders. While significant challenges remain, the potential for manipulating neuronal activity to improve metabolic health is promising. However, ethical considerations must be carefully addressed to ensure that these therapies are safe, effective, and ethically sound. Future research should focus on developing more sophisticated tools to map and manipulate neuronal circuits, identifying novel neuronal subtypes, and elucidating the molecular mechanisms underlying neuronal plasticity. By addressing these challenges, we can unlock the full potential of neuronal-targeted therapies for metabolic disorders and improve the lives of millions of people worldwide. This endeavor requires a multi-faceted approach that incorporates advanced research methodologies, rigorous ethical evaluation, and collaborative partnerships across disciplines.
Many thanks to our sponsor Esdebe who helped us prepare this research report.
References
[1] Schwartz, M. W., Woods, S. C., Porte, D., Seeley, R. J., & Baskin, D. G. (2000). Central nervous system control of food intake. Nature, 404(6778), 661-671.
[2] Grill, H. J., & Hayes, M. R. (2012). Hypothalamic and brainstem integration of energy balance. Physiological Reviews, 92(2), 627-666.
[3] Elmquist, J. K., Elias, C. F., & Saper, C. B. (1999). From lesions to leptin: hypothalamic control of food intake and body weight. Neuron, 22(2), 221-232.
[4] Schwartz, M. W., Seeley, R. J., Campfield, L. A., Burn, P., & Woods, S. C. (1996). Identification of targets of leptin action in rat brain. Journal of Clinical Investigation, 98(5), 1101.
[5] Meek, T. H., & Morton, G. J. (2016). The role of the ventromedial hypothalamus in glucose homeostasis. American Journal of Physiology-Regulatory, Integrative and Comparative Physiology, 310(11), R1157-R1167.
[6] Su, Y., et al. (2017). Identification of a neuronal population in the ventromedial hypothalamus that regulates energy expenditure. Cell, 170(4), 727-738.e17.
[7] Choi, D. L., Davis, J. F., Benoit, S. C., & Clegg, D. J. (2013). The role of the dorsomedial hypothalamus in the regulation of energy balance. Endocrinology, 154(1), 3-9.
[8] Sakurai, T. (2007). The neural circuit of orexin (hypocretin): its role in the regulation of sleep and wakefulness. Archives Italiennes de Biologie, 145(2-3), 93-111.
[9] Berthoud, H. R. (2011). Metabolic and hedonic drives in the neural control of appetite. Current Biology, 21(19), R757-R766.
[10] Dockray, G. J. (2013). Gut hormone regulation of appetite. Annals of the New York Academy of Sciences, 1281(1), 1-17.
[11] Ulrich-Lai, Y. M., & Herman, J. P. (2009). Neural regulation of endocrine and autonomic responses to stress. Nature Reviews Neuroscience, 10(6), 397-409.
[12] Volkow, N. D., Wang, G. J., Tomasi, D., & Baler, R. D. (2013). Obesity and addiction: neurobiological parallels. Obesity Reviews, 14(1), 2-18.
[13] Blouet, C., & Schwartz, G. J. (2010). The central control of energy balance: an overview. Diabetes, Obesity and Metabolism, 12 Suppl 2, 1-14.
[14] Smart, B. J., & Jerman, J. C. (2012). Orexin receptor antagonists as potential therapeutics for insomnia. Annual Reports in Medicinal Chemistry, 47, 239-250.
[15] Saltiel, A. R., & Kahn, C. R. (2001). Insulin signalling and the regulation of glucose and lipid metabolism. Nature, 414(6865), 799-806.
[16] Greenway, F. L. (2015). Physiological adaptations to weight loss and the need for sustained lifestyle change. Obesity Reviews, 16(1), 1-9.
[17] Roth, C. L., Joseph, D. P., & Gill, R. S. (2014). Emerging therapies in obesity: a focus on gut hormones. Journal of Obesity, 2014.
This is a fascinating overview. The discussion on ethical considerations, particularly regarding potential unintended consequences of manipulating brain activity for metabolic benefit, is crucial and warrants further exploration. How do we balance individual autonomy with societal benefits in this rapidly advancing field?