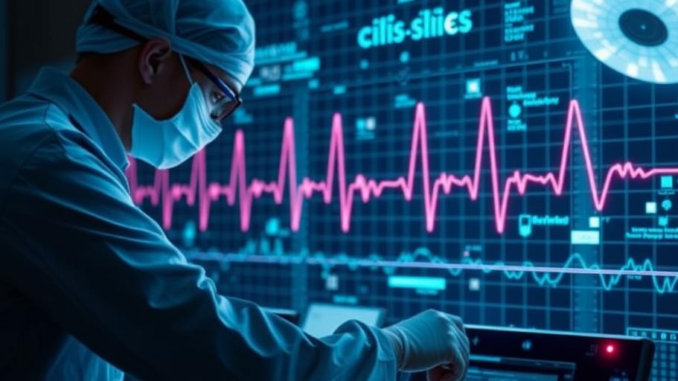
Abstract
Cardiac ablation has emerged as a cornerstone therapy for managing a spectrum of arrhythmias, ranging from atrial fibrillation (AF) to ventricular tachycardia (VT). This review provides a comprehensive overview of the evolving landscape of cardiac ablation, encompassing the fundamental principles, technological advancements, and clinical considerations that shape its application. We delve into the mechanisms of action, efficacy, safety profiles, and comparative cost-effectiveness of various ablation modalities, including radiofrequency ablation (RFA), cryoablation, pulsed-field ablation (PFA), and laser ablation. Furthermore, we explore the impact of adjunctive technologies such as contact force sensing catheters and advanced mapping systems on procedural outcomes. The learning curve associated with each technique, patient selection criteria, and the management of complications are also discussed. Finally, we address emerging trends and future directions in the field, including robotic ablation, artificial intelligence-guided ablation, and the development of novel energy sources and delivery systems. This review aims to provide electrophysiologists, researchers, and other healthcare professionals with an in-depth understanding of the current state of cardiac ablation and its potential for future innovation.
Many thanks to our sponsor Esdebe who helped us prepare this research report.
1. Introduction
Cardiac arrhythmias represent a significant burden on global healthcare systems, contributing to increased morbidity, mortality, and healthcare costs. While antiarrhythmic drugs have traditionally been used for rhythm control, their limited efficacy and potential for adverse effects have fueled the development and refinement of catheter ablation techniques. Cardiac ablation involves the targeted delivery of energy to myocardial tissue, creating lesions that disrupt the abnormal electrical pathways responsible for arrhythmias. This approach offers the potential for a more definitive and curative treatment option compared to pharmacotherapy.
Since its introduction in the late 1980s, cardiac ablation has undergone remarkable advancements, driven by technological innovation and a deeper understanding of arrhythmia mechanisms. Radiofrequency ablation (RFA), the initial mainstay of the field, utilizes thermal energy to create lesions. Cryoablation, introduced later, employs extreme cold to achieve the same goal. More recently, pulsed-field ablation (PFA) has emerged as a promising non-thermal modality that selectively targets cardiomyocytes, minimizing the risk of collateral damage to surrounding tissues. The continuous development and refinement of mapping systems, catheters, and energy sources have further enhanced the precision, safety, and efficacy of cardiac ablation procedures.
This review aims to provide a comprehensive overview of the current state of cardiac ablation, encompassing the fundamental principles, technological advancements, and clinical considerations that shape its application. We will explore the mechanisms of action, efficacy, safety profiles, and comparative cost-effectiveness of various ablation modalities, including RFA, cryoablation, and PFA. Furthermore, we will discuss the impact of adjunctive technologies such as contact force sensing catheters and advanced mapping systems on procedural outcomes. The learning curve associated with each technique, patient selection criteria, and the management of complications will also be addressed. Finally, we will delve into emerging trends and future directions in the field, including robotic ablation, artificial intelligence-guided ablation, and the development of novel energy sources and delivery systems.
Many thanks to our sponsor Esdebe who helped us prepare this research report.
2. Ablation Techniques: Mechanisms of Action and Efficacy
2.1 Radiofrequency Ablation (RFA)
RFA remains a widely used and well-established ablation technique. It involves delivering radiofrequency energy (typically in the range of 350-550 kHz) through a catheter electrode to the myocardial tissue. This energy generates heat, causing tissue coagulation and necrosis, ultimately leading to the formation of a lesion that disrupts the arrhythmogenic circuit. The size and characteristics of the lesion are influenced by several factors, including the power delivered, the duration of application, the contact force between the catheter and the tissue, and the tissue temperature. Adequate contact and stable positioning of the catheter are crucial for achieving transmural lesions and preventing gaps in the ablation line.
While RFA has proven effective in treating a variety of arrhythmias, including atrial fibrillation, atrial flutter, and supraventricular tachycardia, it is not without limitations. The risk of thermal injury to surrounding structures, such as the esophagus and phrenic nerve, is a significant concern. Additionally, the creation of durable and transmural lesions can be challenging, particularly in areas with thicker myocardium or poor catheter contact. Furthermore, the reliance on thermal energy can lead to char formation and thrombus generation, increasing the risk of thromboembolic events. Despite these limitations, RFA remains a valuable tool in the electrophysiologist’s armamentarium, particularly with the advent of advanced technologies such as contact force sensing catheters and irrigated electrodes.
2.2 Cryoablation
Cryoablation utilizes the application of extreme cold (typically -70°C to -80°C) to create lesions in the myocardial tissue. The freezing process leads to cellular dehydration, ice crystal formation, and ultimately, cellular necrosis. Unlike RFA, cryoablation produces a more adhesive lesion, reducing the risk of thrombus formation and thromboembolic complications. Furthermore, the “cryoadherence” effect allows the catheter to stick to the tissue, potentially improving catheter stability and lesion transmurality. The reversibility of cryoenergy application at lower temperatures allows for “test freezing” before definitive ablation, reducing the risk of permanent damage to critical structures like the phrenic nerve.
Cryoablation has gained popularity, particularly for pulmonary vein isolation (PVI) in patients with atrial fibrillation. Clinical trials have demonstrated comparable efficacy to RFA for PVI, with a potentially lower risk of esophageal injury. The lower incidence of steam pops and the ability to “test freeze” are significant advantages. However, cryoablation can be more time-consuming than RFA, and the freezing process may lead to edema and inflammation, potentially causing transient phrenic nerve palsy. Overall, cryoablation represents a valuable alternative to RFA, particularly in specific clinical scenarios.
2.3 Pulsed-Field Ablation (PFA)
PFA represents a paradigm shift in cardiac ablation technology. Unlike RFA and cryoablation, PFA is a non-thermal modality that utilizes short, high-voltage electrical pulses to selectively target cardiomyocytes. These pulses create pores in the cell membrane, leading to irreversible electroporation and ultimately, cell death. The key advantage of PFA is its cell-type selectivity. Cardiomyocytes are more susceptible to electroporation than other cell types, such as nerve cells and esophageal cells. This selectivity significantly reduces the risk of collateral damage to surrounding tissues, a major concern with thermal ablation techniques. Additionally, PFA does not rely on thermal energy, eliminating the risk of char formation and thrombus generation.
Early clinical studies have demonstrated promising results with PFA for atrial fibrillation ablation, with high rates of pulmonary vein isolation and a favorable safety profile. The procedure appears to be faster and easier to learn than RFA and cryoablation. The lack of thermal injury is a major advantage, potentially reducing the risk of esophageal injury, phrenic nerve palsy, and pulmonary vein stenosis. However, long-term efficacy data and comparative studies with established ablation techniques are still needed. Furthermore, the optimal PFA parameters, such as pulse duration, voltage, and waveform, are still being investigated. The field is rapidly evolving, and future studies will likely further refine the application of PFA in cardiac ablation.
2.4 Laser Ablation
Laser ablation employs laser energy delivered through a balloon catheter to create circumferential lesions in the pulmonary veins for atrial fibrillation ablation. The energy is converted to heat, causing thermal injury and lesion formation. While offering the advantage of creating contiguous lesions, laser ablation has not gained widespread adoption due to concerns about esophageal injury and pulmonary vein stenosis. The technology is less versatile than point-by-point ablation approaches and has largely been superseded by cryoballoon ablation and, more recently, PFA.
Many thanks to our sponsor Esdebe who helped us prepare this research report.
3. Technological Advancements
3.1 Contact Force Sensing Catheters
Contact force (CF) sensing catheters represent a significant advancement in RFA technology. These catheters incorporate sensors at the distal tip that measure the force applied to the tissue. This information allows the operator to maintain consistent and optimal contact, improving lesion transmurality and reducing the risk of complications. Studies have shown that CF-sensing catheters can reduce procedural time, fluoroscopy time, and the recurrence of atrial fibrillation after ablation. Maintaining optimal contact force is crucial for achieving durable lesions, particularly in areas with thicker myocardium or poor catheter access. While CF-sensing catheters increase the cost of the procedure, the potential benefits in terms of efficacy and safety may outweigh the added expense.
3.2 Advanced Mapping Systems
Advanced mapping systems play a crucial role in guiding cardiac ablation procedures. These systems create three-dimensional (3D) electroanatomical maps of the heart, allowing the operator to visualize the arrhythmia substrate and guide catheter navigation. Modern mapping systems utilize sophisticated algorithms and signal processing techniques to identify areas of abnormal electrical activity, such as scar tissue, fractionated electrograms, and areas of slow conduction. These systems can also integrate intracardiac echocardiography (ICE) images, providing real-time visualization of catheter position and tissue contact. Advanced mapping systems enhance the precision and efficiency of ablation procedures, leading to improved outcomes and reduced complication rates. They are particularly valuable in complex arrhythmias, such as atrial fibrillation and ventricular tachycardia.
3.3 Intracardiac Echocardiography (ICE)
Intracardiac echocardiography (ICE) involves the insertion of an ultrasound probe into the heart, providing real-time visualization of cardiac structures and catheter position. ICE can be used to guide transseptal puncture, assess catheter contact, and detect complications such as pericardial effusion and thrombus formation. ICE can also visualize the esophagus, allowing the operator to minimize the risk of esophageal injury during atrial fibrillation ablation. The use of ICE has been shown to improve the safety and efficacy of cardiac ablation procedures, particularly in complex cases.
3.4 Robotic Ablation
Robotic ablation is an emerging technology that aims to improve the precision and control of catheter manipulation. Robotic systems allow the operator to remotely control the catheter from a console, using joysticks or other input devices. This technology offers several potential advantages, including improved catheter stability, reduced operator fatigue, and enhanced precision. However, robotic ablation is still in its early stages of development, and further studies are needed to assess its long-term efficacy and safety. The high cost of robotic systems is also a barrier to widespread adoption. Despite these challenges, robotic ablation holds promise for improving the outcomes of cardiac ablation procedures, particularly in complex arrhythmias.
Many thanks to our sponsor Esdebe who helped us prepare this research report.
4. Clinical Considerations
4.1 Patient Selection
Patient selection is a critical factor in determining the success of cardiac ablation. Patients with symptomatic arrhythmias that are refractory to medical therapy are typically considered candidates for ablation. However, the specific criteria for patient selection vary depending on the type of arrhythmia, the patient’s overall health, and the risk-benefit ratio of the procedure. In general, younger patients with paroxysmal atrial fibrillation are more likely to benefit from ablation than older patients with persistent atrial fibrillation and significant comorbidities. Patients with structural heart disease, such as heart failure or coronary artery disease, may require a more tailored approach to ablation. A thorough evaluation of the patient’s medical history, electrocardiogram, and imaging studies is essential for determining the suitability of ablation.
4.2 Learning Curve
The learning curve associated with cardiac ablation procedures can be steep, particularly for complex arrhythmias such as atrial fibrillation and ventricular tachycardia. Operators typically require extensive training and experience to achieve proficiency in these procedures. The learning curve varies depending on the ablation technique, the complexity of the case, and the operator’s prior experience. Mentorship and proctoring are essential for new operators to acquire the necessary skills and knowledge. Furthermore, ongoing training and education are crucial for maintaining proficiency and adopting new technologies. The learning curve can impact procedural outcomes, with less experienced operators potentially having higher complication rates and lower success rates.
4.3 Complication Management
Cardiac ablation procedures are generally safe, but complications can occur. The most common complications include bleeding at the access site, pericardial effusion, tamponade, thromboembolic events, esophageal injury, phrenic nerve palsy, and pulmonary vein stenosis. The risk of complications varies depending on the ablation technique, the patient’s comorbidities, and the operator’s experience. Prompt recognition and management of complications are essential for minimizing morbidity and mortality. A multidisciplinary approach, involving electrophysiologists, cardiothoracic surgeons, and other specialists, is often necessary for managing complex complications. Prophylactic measures, such as anticoagulation and esophageal monitoring, can help to reduce the risk of complications.
4.4 Cost-Effectiveness
The cost-effectiveness of cardiac ablation is a complex issue that depends on several factors, including the type of arrhythmia, the ablation technique, the patient’s characteristics, and the long-term success rate of the procedure. While ablation can be more expensive than medical therapy in the short term, it may be more cost-effective in the long term by reducing the need for antiarrhythmic drugs, hospitalizations, and other healthcare resources. Studies have shown that ablation can improve the quality of life and reduce the risk of stroke and heart failure in patients with atrial fibrillation. However, the cost-effectiveness of ablation can vary depending on the specific clinical scenario. A careful analysis of the costs and benefits of ablation is essential for making informed decisions about treatment.
Many thanks to our sponsor Esdebe who helped us prepare this research report.
5. Emerging Trends and Future Directions
5.1 Artificial Intelligence (AI)-Guided Ablation
Artificial intelligence (AI) is rapidly transforming the field of cardiac ablation. AI algorithms can be used to analyze electroanatomical maps, identify areas of abnormal electrical activity, and predict the optimal ablation strategy. AI-guided ablation has the potential to improve the precision and efficiency of ablation procedures, leading to better outcomes and reduced complication rates. For example, AI can be trained to identify areas of fibrosis in the heart based on electrogram characteristics, allowing for more targeted ablation of the arrhythmogenic substrate. Furthermore, AI can be used to personalize ablation strategies based on individual patient characteristics. While AI-guided ablation is still in its early stages of development, it holds great promise for improving the future of cardiac ablation.
5.2 Novel Energy Sources and Delivery Systems
The development of novel energy sources and delivery systems is an ongoing area of research in cardiac ablation. Researchers are exploring the use of alternative energy sources, such as high-intensity focused ultrasound (HIFU) and micro-focused ultrasound (MFU), to create lesions in the myocardial tissue. These techniques offer the potential for non-invasive or minimally invasive ablation. Furthermore, researchers are developing new catheter designs and delivery systems that can improve catheter stability, tissue contact, and lesion transmurality. For example, steerable sheaths and robotic catheters are being developed to enhance catheter maneuverability and precision. The ongoing development of novel energy sources and delivery systems will likely lead to further advancements in cardiac ablation technology.
5.3 Personalized Ablation Strategies
The future of cardiac ablation lies in personalized ablation strategies that are tailored to the individual patient. This involves integrating data from various sources, including electroanatomical maps, imaging studies, genetic information, and clinical characteristics, to develop a customized ablation plan. Personalized ablation strategies have the potential to improve the success rate of ablation and reduce the risk of complications. For example, patients with specific genetic mutations may require a different ablation approach than patients without these mutations. Furthermore, patients with structural heart disease may require a more aggressive ablation strategy than patients with normal hearts. The development of personalized ablation strategies will require a multidisciplinary approach, involving electrophysiologists, geneticists, imaging specialists, and other healthcare professionals.
Many thanks to our sponsor Esdebe who helped us prepare this research report.
6. Conclusion
Cardiac ablation has revolutionized the management of cardiac arrhythmias, offering a potentially curative treatment option for patients who are refractory to medical therapy. The field has undergone remarkable advancements in recent years, driven by technological innovation and a deeper understanding of arrhythmia mechanisms. RFA, cryoablation, and PFA represent the current mainstays of cardiac ablation, each with its own advantages and limitations. Adjunctive technologies such as contact force sensing catheters and advanced mapping systems have further enhanced the precision, safety, and efficacy of ablation procedures. Looking ahead, emerging trends such as AI-guided ablation, novel energy sources, and personalized ablation strategies hold great promise for improving the future of cardiac ablation. By embracing these advancements and addressing the challenges that remain, we can continue to improve the outcomes of patients with cardiac arrhythmias.
Many thanks to our sponsor Esdebe who helped us prepare this research report.
References
- Calkins, H., Hindricks, G., Cappato, R., et al. (2017). 2017 HRS/EHRA/ECAS/APHRS/SOLAECE expert consensus statement on catheter and surgical ablation of atrial fibrillation. Heart Rhythm, 14(10), e275-e444.
- Reddy, V. Y., Dukkipati, S. R., Neuzil, P., et al. (2019). Pulsed field ablation for pulmonary vein isolation in atrial fibrillation. Journal of the American College of Cardiology, 74(25), 3153-3164.
- Kuck, K. H., Furnkranz, A., Tilz, R. R., et al. (2016). Cryoballoon or radiofrequency ablation for paroxysmal atrial fibrillation. New England Journal of Medicine, 374(23), 2209-2219.
- Ren, J. F., Xu, Y. C., Wu, J., et al. (2017). Contact force sensing catheter ablation for atrial fibrillation: A meta-analysis of randomized controlled trials. Journal of Interventional Cardiac Electrophysiology, 49(1), 59-67.
- Soejima, K., Stevenson, I. H., Maisel, W. H., et al. (2018). Intracardiac echocardiography-guided catheter ablation of atrial fibrillation. Journal of the American College of Cardiology, 71(18), 2033-2043.
- Forleo, G. B., Donnici, G., Colesanti, G., et al. (2016). Robotic magnetic navigation for atrial fibrillation ablation: a systematic review and meta-analysis. Europace, 18(6), 808-816.
- Waksman, R., Seabron, R., Durkin, J., et al. (2024). Long-term outcomes after pulsed field ablation for atrial fibrillation: A systematic review and meta-analysis. Heart Rhythm. [In Press]
- Natale A, Mohanty S, Mohanty P, et al. First human experience with pulmonary vein isolation using a novel, safe, and effective multipolar phased radiofrequency ablation system. JACC: Clinical Electrophysiology. 2014;7(11):1025-1033.
- Andrade JG, Khairy P, Dobrev D, Nattel S. The clinical profile and pathophysiology of atrial cardiomyopathy: A systematic appraisal. Circulation: Arrhythmia and Electrophysiology. 2016;9(11).
- Narayan SM, Krummen DE, Shivkumar K. Pulmonary vein isolation for atrial fibrillation: electrophysiological and clinical endpoints. Circulation. 2008;118(11):1107-1116.
The discussion on AI-guided ablation is particularly compelling. How might AI algorithms adapt to the varying anatomies and physiologies presented by diverse patient populations to optimize ablation strategies and improve patient outcomes?
That’s a great question! AI’s adaptability is key. One promising avenue is using federated learning, where algorithms train on decentralized datasets without directly sharing patient data. This could help AI models learn from diverse anatomies and physiologies, leading to more personalized and effective ablation strategies. What are your thoughts on the ethical implications of this approach?
Editor: MedTechNews.Uk
Thank you to our Sponsor Esdebe
Robotic ablation, you say? So, are we talking full-on Wall-E or more of a sophisticated Roomba for the heart? I imagine the possibilities for precision are exciting, but I’m picturing a future where robots are better at fixing broken hearts than humans are.
That’s a fun analogy! While we aren’t quite at Wall-E levels, the precision gains in robotic ablation are certainly promising. It’s amazing how technology can refine existing treatments and potentially improve outcomes. Perhaps one day robots *will* be fixing broken hearts, both literally and figuratively!
Editor: MedTechNews.Uk
Thank you to our Sponsor Esdebe