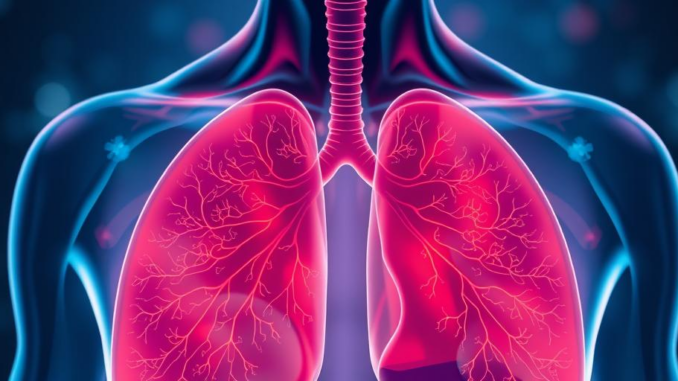
Abstract
Lung regeneration, the process of repairing or regrowing damaged lung tissue, holds immense promise for treating a wide range of respiratory diseases, including chronic obstructive pulmonary disease (COPD), pulmonary fibrosis, and acute respiratory distress syndrome (ARDS). While the lung possesses some inherent regenerative capacity, this is often insufficient to overcome severe injury or chronic disease progression. This review provides a comprehensive overview of the current state of lung regeneration research, encompassing the biological mechanisms underlying lung homeostasis and repair, the role of various cell types including stem and progenitor cells, the application of growth factors and biomaterials, and the potential of emerging technologies such as gene editing and decellularized lung scaffolds. We critically evaluate the successes and limitations of pre-clinical studies and early clinical trials, and identify key challenges and future directions for translating regenerative strategies into effective therapies for human lung diseases. Furthermore, we discuss ethical considerations surrounding lung regeneration research and clinical applications.
Many thanks to our sponsor Esdebe who helped us prepare this research report.
1. Introduction
The respiratory system, responsible for vital gas exchange, is constantly exposed to environmental insults, including pollutants, pathogens, and irritants. As a result, the lung is susceptible to a variety of acute and chronic diseases that can significantly impair its function and lead to substantial morbidity and mortality. While current therapies can manage symptoms and slow disease progression, they often fail to provide a cure or reverse the underlying lung damage. Lung transplantation, although a life-saving option for end-stage respiratory failure, is limited by donor availability, rejection risk, and long-term complications.
Lung regeneration represents a paradigm shift in the treatment of lung diseases by aiming to restore damaged lung tissue to its functional state. This approach holds the potential to address the root cause of respiratory diseases, improve lung function, and ultimately enhance patient survival and quality of life. However, lung regeneration is a complex process that involves the coordinated interaction of multiple cell types, growth factors, and signaling pathways. A deep understanding of these mechanisms is crucial for developing effective regenerative therapies.
This review aims to provide a comprehensive overview of the latest advancements in lung regeneration research, focusing on the key biological processes involved, the various therapeutic strategies being explored, and the challenges and opportunities that lie ahead. We also discuss the ethical considerations that must be addressed to ensure the responsible and equitable development of lung regenerative medicine.
Many thanks to our sponsor Esdebe who helped us prepare this research report.
2. Biological Mechanisms of Lung Regeneration
2.1 Lung Development and Homeostasis
Understanding the developmental processes that establish the lung’s intricate structure and function is critical for informing regenerative strategies. Lung development begins early in embryogenesis with the formation of the lung bud from the foregut endoderm. This bud undergoes a series of branching morphogenetic events, guided by complex signaling pathways involving growth factors such as fibroblast growth factors (FGFs), bone morphogenetic proteins (BMPs), and Wnt ligands, to generate the elaborate airway tree and alveolar structures [1]. Specific progenitor cell populations, including basal cells in the airways and alveolar type 1 (AT1) and alveolar type 2 (AT2) cells in the alveoli, differentiate into specialized cell types responsible for gas exchange and surfactant production [2].
In the adult lung, homeostasis is maintained by a delicate balance between cell proliferation, differentiation, and apoptosis. Alveolar type 2 (AT2) cells serve as progenitor cells capable of self-renewal and differentiation into AT1 cells, thereby maintaining the alveolar epithelium [3]. Basal cells in the airways also contribute to epithelial repair following injury. However, the regenerative capacity of the adult lung is limited, and severe or chronic injury can overwhelm these intrinsic repair mechanisms, leading to fibrosis and impaired lung function.
2.2 Stem and Progenitor Cells in Lung Regeneration
Stem and progenitor cells play a central role in lung regeneration by providing a source of new cells to replace damaged tissue. Several types of stem and progenitor cells have been identified in the lung, each with distinct characteristics and regenerative potential.
2.2.1 Resident Lung Stem/Progenitor Cells:
- Basal Cells: Located in the large airways, basal cells are multipotent progenitor cells capable of differentiating into ciliated cells, goblet cells, and club cells [4]. They play a critical role in airway epithelial repair following injury and are considered the primary progenitor cells for airway regeneration.
- Alveolar Type 2 (AT2) Cells: As mentioned previously, AT2 cells serve as facultative progenitor cells in the alveoli, capable of self-renewal and differentiation into AT1 cells to maintain the alveolar epithelium [3]. In response to injury, AT2 cells proliferate and migrate to damaged areas, contributing to alveolar repair.
- Club Cells: Primarily found in the distal airways, club cells secrete surfactant components and detoxifying enzymes. They can differentiate into ciliated cells and are believed to contribute to airway epithelial repair in certain contexts [5].
2.2.2 Bone Marrow-Derived Stem Cells (BMDSCs):
BMDSCs, including mesenchymal stem cells (MSCs) and hematopoietic stem cells (HSCs), have been shown to contribute to lung repair and regeneration in pre-clinical studies. While their mechanism of action is still under investigation, it is believed that BMDSCs exert their regenerative effects primarily through paracrine signaling, releasing growth factors and cytokines that stimulate endogenous lung repair mechanisms [6].
2.2.3 Induced Pluripotent Stem Cells (iPSCs):
iPSCs, generated by reprogramming adult somatic cells into a pluripotent state, hold tremendous potential for lung regeneration. They can be differentiated into various lung cell types, including alveolar epithelial cells and airway epithelial cells, providing a potentially unlimited source of cells for transplantation or in vitro lung tissue engineering [7].
2.3 Growth Factors and Signaling Pathways
Growth factors and signaling pathways play a crucial role in regulating lung development, homeostasis, and regeneration. Several growth factors have been implicated in lung repair, including:
- Fibroblast Growth Factors (FGFs): FGFs are involved in lung branching morphogenesis, alveolar development, and epithelial cell proliferation and differentiation. FGF7 (keratinocyte growth factor, KGF) and FGF10 have been shown to promote lung epithelial repair in animal models of lung injury [8].
- Hepatocyte Growth Factor (HGF): HGF promotes epithelial cell proliferation, migration, and survival. It has been shown to protect against lung injury and promote lung regeneration in preclinical studies [9].
- Vascular Endothelial Growth Factor (VEGF): VEGF is a potent angiogenic factor that promotes blood vessel formation and endothelial cell survival. It plays a crucial role in maintaining alveolar-capillary barrier integrity and has been shown to improve lung function in animal models of lung injury [10].
- Transforming Growth Factor-β (TGF-β): TGF-β is a multifunctional cytokine involved in cell proliferation, differentiation, and extracellular matrix production. While TGF-β is essential for lung development, its dysregulation can contribute to pulmonary fibrosis [11].
Signaling pathways, such as the Wnt, Notch, and Hedgehog pathways, also play critical roles in regulating lung development and regeneration [12]. Understanding the complex interplay of these growth factors and signaling pathways is essential for developing targeted therapies to promote lung repair.
Many thanks to our sponsor Esdebe who helped us prepare this research report.
3. Therapeutic Strategies for Lung Regeneration
3.1 Cell-Based Therapies
Cell-based therapies involve the transplantation of stem or progenitor cells into the damaged lung to promote tissue repair. Several cell types have been investigated for their potential to regenerate lung tissue, including mesenchymal stem cells (MSCs), bone marrow-derived stem cells (BMDSCs), and induced pluripotent stem cell (iPSC)-derived lung cells.
3.1.1 Mesenchymal Stem Cells (MSCs):
MSCs are multipotent stromal cells that can be isolated from various tissues, including bone marrow, adipose tissue, and umbilical cord blood. MSCs have demonstrated promising therapeutic effects in preclinical models of lung injury, including ARDS and pulmonary fibrosis. MSCs are believed to exert their regenerative effects primarily through paracrine signaling, releasing growth factors and cytokines that modulate the inflammatory response, promote angiogenesis, and stimulate endogenous lung repair [6].
Clinical trials evaluating the safety and efficacy of MSCs for treating ARDS and other lung diseases have yielded mixed results. While some studies have reported improvements in lung function and clinical outcomes, others have shown no significant benefit. Further research is needed to optimize MSC delivery methods, cell dosage, and patient selection criteria to improve the efficacy of MSC-based therapies.
3.1.2 Induced Pluripotent Stem Cells (iPSCs):
iPSCs offer a potentially unlimited source of lung cells for transplantation. iPSCs can be differentiated into various lung cell types, including alveolar epithelial cells, airway epithelial cells, and endothelial cells. iPSC-derived lung cells have shown promise in preclinical studies for treating lung injury and disease [7].
However, several challenges remain before iPSC-based therapies can be translated into clinical practice, including the risk of teratoma formation, the need for efficient and reproducible differentiation protocols, and the potential for immune rejection. Immunocompatibility is a major concern for iPSC based therapies, and techniques to overcome this are under development, for instance using CRISPR to remove MHC molecules from iPSCs [13].
3.2 Growth Factor Therapy
Growth factors can be administered directly to the lung to stimulate endogenous repair mechanisms. Several growth factors, including FGF7 (KGF), HGF, and VEGF, have shown promise in preclinical models of lung injury [8, 9, 10].
Clinical trials evaluating the efficacy of growth factor therapy for treating lung diseases have been limited. Aerosolized KGF has been investigated for the treatment of ARDS, but the results have been inconclusive. Further research is needed to identify the optimal growth factors, delivery methods, and patient populations for growth factor therapy.
3.3 Gene Therapy
Gene therapy involves the delivery of therapeutic genes to the lung to correct genetic defects or enhance lung repair. Several gene therapy approaches have been investigated for the treatment of lung diseases, including:
- Gene augmentation therapy: This approach involves delivering a functional copy of a gene that is mutated or deficient in patients with genetic lung diseases, such as cystic fibrosis.
- Gene editing: Gene editing technologies, such as CRISPR-Cas9, can be used to correct genetic mutations in lung cells or to enhance lung repair mechanisms [14]. CRISPR has been used to improve the immunocompatibility of iPSCs by removing MHC molecules [13].
Gene therapy has shown promise in preclinical studies for treating various lung diseases. However, several challenges remain, including the development of safe and efficient gene delivery vectors, the potential for off-target effects, and the long-term durability of gene expression.
3.4 Biomaterials and Tissue Engineering
Biomaterials and tissue engineering approaches aim to create functional lung tissue in vitro or in vivo to replace damaged lung tissue. Several strategies are being explored, including:
- Decellularized lung scaffolds: Decellularized lung scaffolds are generated by removing all cells from a donor lung, leaving behind the extracellular matrix (ECM). The ECM provides a natural scaffold that can be recellularized with lung cells to create a functional lung graft [15].
- 3D bioprinting: 3D bioprinting involves the layer-by-layer deposition of cells, biomaterials, and growth factors to create complex lung structures. This technology holds the potential to generate customized lung grafts for transplantation [16].
Biomaterials and tissue engineering approaches are still in the early stages of development, but they hold great promise for lung regeneration. Challenges include the development of biocompatible and biodegradable biomaterials, the efficient recellularization of lung scaffolds, and the creation of functional alveolar structures.
Many thanks to our sponsor Esdebe who helped us prepare this research report.
4. Challenges and Limitations
Despite significant progress in lung regeneration research, several challenges and limitations remain that need to be addressed to translate regenerative strategies into effective therapies for human lung diseases.
- Limited Understanding of Lung Regeneration Mechanisms: Our understanding of the biological mechanisms underlying lung regeneration is still incomplete. Further research is needed to identify the key signaling pathways, cell types, and environmental factors that promote lung repair.
- Difficulty in Delivering Cells and Growth Factors to the Lung: Efficient and targeted delivery of cells and growth factors to the damaged lung tissue remains a major challenge. Current delivery methods, such as intravenous injection and aerosol inhalation, often result in poor cell engraftment and limited distribution of growth factors.
- Risk of Fibrosis and Scarring: Excessive inflammation and extracellular matrix deposition can lead to fibrosis and scarring, which can impair lung function. Strategies to prevent or reverse fibrosis are crucial for successful lung regeneration.
- Immune Rejection: Transplanted cells and tissues can be rejected by the host immune system, leading to graft failure. Immunosuppression is often required to prevent rejection, but it can increase the risk of infection and other complications.
- Ethical Considerations: Lung regeneration research raises several ethical considerations, including the use of human embryonic stem cells, the potential for off-target effects of gene editing, and the equitable access to regenerative therapies.
Many thanks to our sponsor Esdebe who helped us prepare this research report.
5. Future Directions
Lung regeneration research is a rapidly evolving field with tremendous potential to transform the treatment of respiratory diseases. Future research efforts should focus on:
- Elucidating the mechanisms of lung regeneration: Deeper understanding of the complex interplay of cells, growth factors, and signaling pathways involved in lung repair is crucial. Single-cell sequencing and spatial transcriptomics can provide valuable insights into the cellular heterogeneity and molecular mechanisms of lung regeneration.
- Developing novel cell delivery strategies: More efficient and targeted cell delivery methods are needed to improve cell engraftment and therapeutic efficacy. Nanoparticle-based delivery systems and cell-laden hydrogels are promising approaches.
- Improving biomaterials and tissue engineering techniques: The development of biocompatible and biodegradable biomaterials that mimic the native lung ECM is essential for creating functional lung grafts. Advances in 3D bioprinting and microfabrication technologies can enable the creation of more complex and realistic lung structures.
- Conducting rigorous clinical trials: Well-designed clinical trials are needed to evaluate the safety and efficacy of lung regenerative therapies in human patients. These trials should include appropriate controls, standardized outcome measures, and long-term follow-up.
- Addressing ethical considerations: Open and transparent discussions are needed to address the ethical considerations surrounding lung regeneration research and clinical applications. Guidelines and regulations should be developed to ensure the responsible and equitable development of regenerative medicine.
Many thanks to our sponsor Esdebe who helped us prepare this research report.
6. Conclusion
Lung regeneration holds great promise for treating a wide range of respiratory diseases. Significant progress has been made in understanding the biological mechanisms underlying lung repair and in developing novel therapeutic strategies. However, several challenges remain before lung regenerative therapies can be translated into clinical practice. Future research efforts should focus on elucidating the mechanisms of lung regeneration, developing novel cell delivery strategies, improving biomaterials and tissue engineering techniques, conducting rigorous clinical trials, and addressing ethical considerations. With continued research and development, lung regeneration has the potential to revolutionize the treatment of respiratory diseases and improve the lives of millions of patients.
Many thanks to our sponsor Esdebe who helped us prepare this research report.
References
[1] Warburton, D., et al. “Mechanisms of early lung development.” Physiological reviews 83.4 (2003): 1575-1626.
[2] Hogan, B. L. M., et al. “Repair and regeneration in the respiratory system.” Nature reviews Molecular cell biology 15.5 (2014): 277-289.
[3] Rock, J. R., et al. “Multiple progenitor populations contribute to mouse lung alveolar regeneration.” Cell stem cell 9.5 (2011): 436-443.
[4] Tata, P. R., et al. “Myoepithelial lineage of basal cells reveals the origins of the lung epithelium.” Nature 502.7471 (2013): 445-448.
[5] Rawlins, E. L., et al. “The role of Scgb1a1+ club cells in airway homeostasis and regeneration.” American journal of respiratory cell and molecular biology 31.1 (2004): 42-49.
[6] Krasnodembskaya, A., et al. “Mesenchymal stem cells transform macrophages into a phenotype that enhances fibroblast engraftment and attenuation of scar formation.” American journal of physiology-lung cellular and molecular physiology 304.10 (2013): L1045-L1059.
[7] Gotoh, S., et al. “Generation of alveolar epithelial cells from human induced pluripotent stem cells via an endodermal route.” PloS one 9.2 (2014): e89924.
[8] Barazzone-Michelet, D., et al. “Keratinocyte growth factor and hepatocyte growth factor induce alveolar epithelial cell proliferation in vitro.” American journal of physiology-lung cellular and molecular physiology 266.6 (1994): L381-L388.
[9] Mason, R. J., et al. “Hepatocyte growth factor protects against lung injury in mice.” American journal of respiratory cell and molecular biology 24.6 (2001): 703-711.
[10] Hashimoto, A., et al. “Vascular endothelial growth factor (VEGF) prevents bleomycin-induced pulmonary fibrosis.” The Journal of clinical investigation 97.7 (1996): 1551-1559.
[11] Willis, B. C., et al. “TGF-β1 induces human lung fibroblast proliferation through EGFR-dependent and independent mechanisms.” American journal of respiratory cell and molecular biology 34.6 (2006): 720-727.
[12] Cardoso, W. V. “Molecular regulation of lung development.” Annual review of physiology 62.1 (2000): 457-482.
[13] Xu, H., Wang, B., Ono, M., Katsuda, T., Takeda, Y., Yabe, H., … & Uede, T. (2019). Disruption of HLA genes enhances the anti-tumor activity of human iPSC-derived NK cells. Cell stem cell, 24(2), 198-200.
[14] Porteous, M. K., et al. “Gene therapy progress and prospects: genome editing.” Gene therapy 24.1 (2017): 1-9.
[15] Ott, H. C., et al. “Regeneration and orthotopic transplantation of a bioartificial lung.” Nature medicine 16.8 (2010): 927-933.
[16] Grigoryan, B., et al. “Bioprinting of complex 3D functional tissues using laser-induced forward transfer.” Science 364.6439 (2019): 458-464.
So, if we are decellularizing lungs, does that mean there is a market for old lungs? Asking for a friend who hoards unusual medical specimens.
That’s a fascinating thought! While we’re not quite at the point of a ‘market’ for decellularized lungs, the potential for repurposing organs that are unsuitable for traditional transplants is definitely an area of active research. Perhaps your friend is a visionary ahead of the curve! It also highlights an interesting element of sustainable science.
Editor: MedTechNews.Uk
Thank you to our Sponsor Esdebe
So, we’re decellularizing lungs now? Does this mean my sourdough starter culture finally has some competition in the obscure lab hobby space? I’m suddenly feeling so basic.