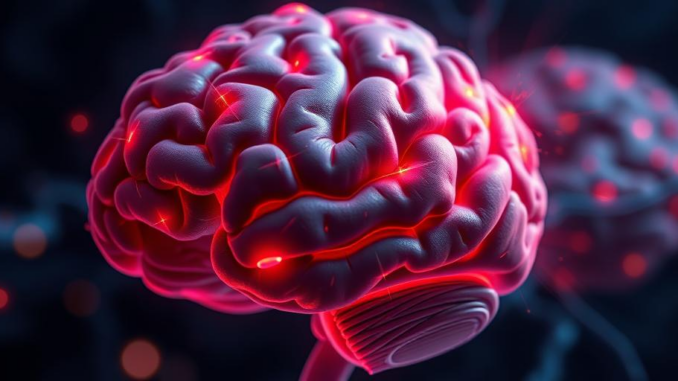
Epilepsy: A Comprehensive Review of Pathophysiology, Diagnosis, Treatment Modalities, and Future Directions
Abstract
Epilepsy, a chronic neurological disorder characterized by recurrent, unprovoked seizures, affects approximately 1% of the global population. Despite advancements in antiepileptic drugs (AEDs) and surgical interventions, a significant proportion of individuals with epilepsy continue to experience uncontrolled seizures, representing a major challenge in clinical management. This report provides a comprehensive overview of epilepsy, encompassing its underlying pathophysiology, diverse seizure types, diagnostic modalities, and a spectrum of treatment options, including pharmacological, surgical, and neuromodulatory approaches. We delve into the complex genetic and environmental factors contributing to epileptogenesis, explore the evolving landscape of neuroimaging and electrophysiological biomarkers for improved diagnosis and prognosis, and critically assess the efficacy and limitations of current therapeutic strategies. Furthermore, we highlight emerging research avenues, such as gene therapy, personalized medicine, and novel neuromodulation techniques, that hold promise for revolutionizing epilepsy management in the future. This review aims to provide a valuable resource for clinicians, researchers, and individuals affected by epilepsy, fostering a deeper understanding of the disease and guiding the development of more effective and targeted therapies.
1. Introduction
Epilepsy is not a single disease entity but rather a heterogeneous group of disorders characterized by the propensity to generate spontaneous, recurrent seizures. The International League Against Epilepsy (ILAE) defines epilepsy as occurring after at least two unprovoked seizures more than 24 hours apart or one unprovoked seizure with a high probability of recurrence over the next 10 years, similar to the general recurrence risk after two unprovoked seizures [1]. The underlying causes of epilepsy are diverse, ranging from genetic mutations and structural brain abnormalities to acquired brain injuries and infections. The clinical manifestations of epilepsy are equally varied, encompassing a wide spectrum of seizure types, from brief, subtle alterations in awareness to convulsive tonic-clonic seizures. The impact of epilepsy extends beyond the physical consequences of seizures, significantly affecting cognitive function, emotional well-being, and quality of life.
Approximately one-third of individuals with epilepsy develop drug-resistant epilepsy (DRE), defined as the failure to achieve sustained seizure freedom with adequate trials of two appropriately chosen and tolerated AEDs [2]. This population faces a significantly increased risk of morbidity, mortality, and psychosocial challenges. Traditional treatment strategies for DRE include surgical resection of epileptogenic tissue and vagus nerve stimulation (VNS). However, these approaches are not always effective or suitable for all patients. Emerging neuromodulatory techniques, such as deep brain stimulation (DBS) and responsive neurostimulation (RNS), offer promising alternative options for seizure control in individuals with DRE. Understanding the intricate pathophysiology of epilepsy, refining diagnostic methods, and developing novel therapeutic strategies are crucial for improving the lives of individuals living with this challenging neurological disorder.
2. Pathophysiology of Epilepsy
The pathophysiology of epilepsy is complex and multifactorial, involving intricate interactions between genetic predispositions, acquired brain injuries, and alterations in neuronal excitability and inhibition. At the cellular level, epileptogenesis, the process by which normal brain tissue develops the propensity to generate seizures, is characterized by a cascade of molecular and structural changes that disrupt the delicate balance between excitation and inhibition. These changes include alterations in ion channel function, neurotransmitter receptor expression, synaptic plasticity, and neuronal network connectivity.
A key mechanism underlying epileptogenesis is the enhancement of excitatory neurotransmission mediated by glutamate. Increased glutamate release, reduced glutamate reuptake, and upregulation of glutamate receptors, such as NMDA receptors, contribute to excessive neuronal excitation and seizure generation [3]. Conversely, a reduction in inhibitory neurotransmission mediated by GABA also plays a critical role in epileptogenesis. Decreased GABA synthesis, impaired GABA receptor function, and reduced GABAergic interneuron activity can lead to disinhibition and increased neuronal excitability [4].
Genetic factors play a significant role in many forms of epilepsy. Mutations in genes encoding ion channels (e.g., sodium, potassium, and calcium channels), neurotransmitter receptors, and synaptic proteins have been identified in various epilepsy syndromes. These mutations can directly affect neuronal excitability and contribute to seizure susceptibility. For example, mutations in SCN1A, a gene encoding a sodium channel subunit, are a common cause of Dravet syndrome, a severe form of childhood epilepsy [5].
Acquired brain injuries, such as traumatic brain injury (TBI), stroke, and infection, are also major risk factors for epilepsy. These injuries can trigger epileptogenesis through various mechanisms, including neuronal cell death, gliosis, inflammation, and alterations in brain connectivity. The latent period between the initial brain injury and the onset of epilepsy can vary widely, ranging from months to years [6].
Inflammation is increasingly recognized as a significant contributor to epileptogenesis. Pro-inflammatory cytokines, such as interleukin-1β (IL-1β) and tumor necrosis factor-α (TNF-α), can enhance neuronal excitability and promote seizure generation. Furthermore, inflammation can disrupt the blood-brain barrier, leading to increased brain permeability and neuronal damage [7].
Recent research has focused on the role of glial cells, particularly astrocytes, in epileptogenesis. Astrocytes play a critical role in regulating extracellular ion concentrations, neurotransmitter levels, and synaptic function. Alterations in astrocyte function, such as impaired glutamate uptake and increased release of pro-inflammatory cytokines, can contribute to neuronal hyperexcitability and seizure generation [8]. The interplay between neurons, glial cells, and the immune system is now considered a crucial aspect of the pathophysiology of epilepsy.
3. Classification and Diagnosis of Epilepsy
The accurate classification of epilepsy syndromes is essential for guiding diagnosis, treatment, and prognosis. The ILAE provides a comprehensive classification system that categorizes epilepsy based on seizure type, epilepsy type, and epilepsy syndrome [9]. Seizure types are classified as focal (originating in one hemisphere) or generalized (originating in both hemispheres simultaneously). Focal seizures can be further classified based on the level of awareness (aware or impaired awareness) and the presence of motor or non-motor symptoms. Generalized seizures include absence seizures, myoclonic seizures, tonic-clonic seizures, tonic seizures, atonic seizures, and clonic seizures. Epilepsy types are classified as focal, generalized, combined generalized and focal, or unknown. Epilepsy syndromes are characterized by a specific constellation of clinical features, including age of onset, seizure type, EEG findings, and associated neurological or cognitive deficits.
The diagnosis of epilepsy typically involves a combination of clinical history, neurological examination, electroencephalography (EEG), and neuroimaging. A detailed clinical history, including a description of the seizures, precipitating factors, and family history of epilepsy, is crucial for establishing the diagnosis. The neurological examination can help identify underlying neurological deficits or structural brain abnormalities.
EEG is a fundamental diagnostic tool for epilepsy, providing a non-invasive measure of brain electrical activity. EEG can detect epileptiform discharges, such as spikes, sharp waves, and spike-wave complexes, which are indicative of increased neuronal excitability. EEG can also help classify seizure types and identify the location of seizure onset. In some cases, prolonged EEG monitoring, including ambulatory EEG or video-EEG monitoring, may be necessary to capture seizures and characterize their electrographic features [10].
Neuroimaging techniques, such as magnetic resonance imaging (MRI), are essential for identifying structural brain abnormalities that may be causing epilepsy. MRI can detect lesions such as hippocampal sclerosis, tumors, cortical dysplasia, and vascular malformations. High-resolution MRI and advanced imaging techniques, such as diffusion tensor imaging (DTI) and functional MRI (fMRI), can provide more detailed information about brain structure and function [11].
In addition to EEG and MRI, other diagnostic modalities, such as positron emission tomography (PET) and single-photon emission computed tomography (SPECT), can be used to assess brain metabolism and blood flow. These techniques can help identify areas of hypometabolism or hyperperfusion that may be associated with epileptogenic tissue [12]. Magnetoencephalography (MEG) is a non-invasive neuroimaging technique that measures magnetic fields generated by neuronal activity. MEG can provide complementary information to EEG and can be particularly useful for localizing seizure onset zones [13].
4. Treatment Modalities for Epilepsy
The primary goal of epilepsy treatment is to achieve seizure freedom with minimal side effects. The cornerstone of epilepsy treatment is pharmacotherapy with AEDs. Numerous AEDs are available, each with its own mechanism of action, efficacy profile, and side effect profile. The choice of AED is typically based on the seizure type, epilepsy syndrome, patient age, and other medical conditions. Commonly used AEDs include phenytoin, carbamazepine, valproic acid, lamotrigine, levetiracetam, and topiramate. Newer generation AEDs, such as brivaracetam, eslicarbazepine acetate, and perampanel, offer alternative options for seizure control [14].
Despite the availability of numerous AEDs, approximately one-third of individuals with epilepsy develop DRE. In these cases, surgical intervention may be considered. Surgical options for epilepsy include resective surgery, in which the epileptogenic tissue is removed, and palliative surgery, which aims to reduce seizure frequency and severity. Resective surgery is typically considered for individuals with focal epilepsy who have a well-defined epileptogenic zone that can be safely resected [15]. Temporal lobe resection is the most common type of resective surgery for epilepsy. Other resective procedures include frontal lobe resection, parietal lobe resection, and extratemporal resections.
Corpus callosotomy, a palliative surgical procedure that involves severing the corpus callosum, can be effective in reducing the frequency of drop attacks in individuals with generalized epilepsy. VNS is another palliative treatment option that involves implanting a device that stimulates the vagus nerve. VNS has been shown to reduce seizure frequency in a subset of individuals with DRE [16].
Neuromodulation techniques, such as DBS and RNS, offer promising alternative treatment options for individuals with DRE. DBS involves implanting electrodes deep within the brain to deliver electrical stimulation to specific brain targets. The most common target for DBS in epilepsy is the anterior nucleus of the thalamus (ANT). DBS of the ANT has been shown to reduce seizure frequency in individuals with DRE [17]. RNS involves implanting a device that detects abnormal brain activity and delivers electrical stimulation to disrupt seizure activity. The NeuroPace RNS System is the only FDA-approved RNS device for epilepsy. RNS has been shown to reduce seizure frequency and improve quality of life in individuals with DRE [18].
In addition to pharmacological and surgical interventions, lifestyle modifications, such as avoiding sleep deprivation, managing stress, and maintaining a healthy diet, can help reduce seizure frequency. The ketogenic diet, a high-fat, low-carbohydrate diet, has been shown to be effective in reducing seizures in some individuals with DRE, particularly children [19].
5. Emerging Research and Future Directions
Research into the pathophysiology, diagnosis, and treatment of epilepsy is rapidly evolving. Emerging research avenues include gene therapy, personalized medicine, and novel neuromodulation techniques. Gene therapy holds promise for correcting the underlying genetic defects that cause some forms of epilepsy. Several gene therapy approaches are being investigated, including viral vector-mediated gene transfer and CRISPR-Cas9 gene editing [20].
Personalized medicine approaches aim to tailor treatment to the individual patient based on their genetic profile, seizure characteristics, and response to therapy. Pharmacogenomics, the study of how genes affect a person’s response to drugs, can help identify individuals who are more likely to respond to specific AEDs or experience adverse effects. Biomarkers, such as EEG features, neuroimaging findings, and blood-based markers, can help predict seizure recurrence and treatment response [21].
Novel neuromodulation techniques, such as transcranial magnetic stimulation (TMS) and transcranial direct current stimulation (tDCS), offer non-invasive approaches to modulate brain activity. TMS involves using magnetic pulses to stimulate or inhibit specific brain regions. tDCS involves applying a weak electrical current to the scalp to modulate neuronal excitability. These techniques are being investigated as potential treatments for epilepsy and as tools for mapping epileptogenic networks [22].
Closed-loop neuromodulation systems, which deliver stimulation based on real-time monitoring of brain activity, are being developed to improve the efficacy and specificity of neuromodulation therapy. These systems can detect preictal activity and deliver stimulation to prevent seizures from occurring. Advances in artificial intelligence (AI) and machine learning are being applied to epilepsy research to improve seizure prediction, diagnostic accuracy, and treatment optimization [23].
6. Social and Economic Impact of Epilepsy
Epilepsy has a significant social and economic impact on individuals, families, and society. Individuals with epilepsy often face challenges related to employment, education, driving, and social stigma. Seizures can lead to injuries, hospitalizations, and lost productivity. The direct and indirect costs of epilepsy are substantial, including medical expenses, lost wages, and disability payments.
Support groups and advocacy organizations play a crucial role in providing information, resources, and emotional support to individuals with epilepsy and their families. These organizations advocate for policies that improve the lives of individuals with epilepsy, such as access to healthcare, employment opportunities, and social inclusion [24]. Public awareness campaigns can help reduce stigma and promote understanding of epilepsy.
7. Conclusion
Epilepsy is a complex and heterogeneous neurological disorder that poses significant challenges to diagnosis, treatment, and management. Despite advancements in AEDs and surgical interventions, a substantial proportion of individuals with epilepsy continue to experience uncontrolled seizures. A deeper understanding of the underlying pathophysiology of epilepsy, refined diagnostic methods, and the development of novel therapeutic strategies are crucial for improving the lives of individuals living with this condition. Emerging research avenues, such as gene therapy, personalized medicine, and novel neuromodulation techniques, offer promising approaches for revolutionizing epilepsy management in the future. Continued research, advocacy, and support are essential to address the social and economic impact of epilepsy and improve the quality of life for individuals affected by this disorder.
References
[1] Fisher, R. S., Acevedo, C., Arzimanoglou, A., Bogacz, A., Cross, J. H., Elger, C. E., … & Zuberi, S. M. (2014). ILAE official report: a practical clinical definition of epilepsy. Epilepsia, 55(4), 475-482.
[2] Kwan, P., Arzimanoglou, A., Berg, A. T., Brodie, M. J., Allen Hauser, W., Mathern, G., … & Engel Jr, J. (2010). Definition of drug resistant epilepsy: Report of the ILAE Task Force on Therapeutic Strategies. Epilepsia, 51(6), 1069-1077.
[3] Meldrum, B. S. (2000). Glutamate as a neurotransmitter in the brain: review of physiology and pathology. The Journal of Nutrition, 130(4S), 1007S-1015S.
[4] Braat, S., van de Berg, W. D. J., & Geurts, J. J. (2015). The role of GABAergic dysfunction in epilepsy. Epilepsy Currents, 15(1), 34-41.
[5] Dravet, C. (2011). Dravet syndrome. Developmental Medicine & Child Neurology, 53(S2), 1-11.
[6] Pitkänen, A., & Engel Jr, J. (2003). Post-traumatic epilepsy. Epilepsia, 44, 13-21.
[7] Vezzani, A., French, J., Bartfai, T., & Aronica, E. (2011). The role of inflammation in epilepsy. Nature Reviews Neurology, 7(1), 31-40.
[8] Seifert, G., Carmignoto, G., & Steinhäuser, C. (2010). Astrocyte dysfunction in epilepsy. Brain Research Reviews, 63(1-2), 212-221.
[9] Scheffer, I. E., Berkovic, S., Capovilla, G., Connolly, M. B., French, J., Guilhoto, L., … & Zuberi, S. M. (2017). ILAE classification of the epilepsies: Position paper of the ILAE Commission for Classification and Terminology. Epilepsia, 58(4), 512-521.
[10] Tatum, W. O. (2021). Handbook of EEG Interpretation. Lippincott Williams & Wilkins.
[11] Bernasconi, A., & Bernasconi, N. (2011). Structural neuroimaging in epilepsy. Epilepsy Currents, 11(4), 132-137.
[12] Van Paesschen, W. (2004). PET and SPECT in epilepsy. Seminars in Neurology, 24(3), 283-291.
[13] Hillebrand, A., Barnes, G. R., Bosboom, J. L., Fuchs, T., Romani, G. L., & Vrba, J. (2005). Magnetoencephalography. Neuroimage, 25(1), 167-186.
[14] Perucca, E., & Tomson, T. (2011). The pharmacological treatment of epilepsy in adults. BMJ, 342, d2085.
[15] Engel Jr, J. (1996). Surgery for seizures. New England Journal of Medicine, 334(10), 647-652.
[16] Ben-Menachem, E., Revesz, D., Simon, B. J., Silberstein, S., & the Vagus Nerve Stimulation Study Group. (1994). Vagus nerve stimulation for the treatment of medically intractable seizures. Neurology, 44(12), 2028-2035.
[17] Fisher, R. S., Salanova, V., Witt, T., Worth, R., Henry, T. R., Gross, R. E., … & Vagus Nerve Stimulation Study Group. (2010). Electrical stimulation of the anterior nucleus of thalamus for treatment of refractory epilepsy. Epilepsia, 51(5), 899-908.
[18] Morrell, M. J. (2011). Responsive cortical stimulation for the treatment of medically intractable partial epilepsy. The Lancet Neurology, 10(11), 947-957.
[19] Neal, E. G., Chaffe, B., Schwartz, R. H., Lawson, M. S., Edwards, N., Fitzsimmons, G., … & Cross, J. H. (2008). The ketogenic diet for the treatment of childhood epilepsy: a randomised controlled trial. The Lancet Neurology, 7(6), 500-506.
[20] Ricard, D., & Brooks-Kayal, A. (2023). Gene therapy for epilepsy: current status and future directions. Current Opinion in Neurology, 36(2), 154-160.
[21] Tate, S. K., & Sisodiya, S. M. (2013). Pharmacogenomics in epilepsy. Pharmacogenomics, 14(16), 2173-2189.
[22] Lahrberg, J. M., & Lüdke, K. (2022). Non-invasive brain stimulation in epilepsy: A systematic review and meta-analysis. Seizure, 97, 141-152.
[23] Thodoroff, E. C., & Goodfellow, M. (2021). Artificial intelligence in epilepsy management. Epilepsy & Behavior, 115, 107662.
[24] Epilepsy Foundation. (n.d.). Retrieved from https://www.epilepsy.com
Given the intricate interplay between genetic and environmental factors in epileptogenesis, how might personalized environmental modification, alongside genetic therapies, contribute to more effective preventative strategies?
That’s a great point! Considering personalized environmental modifications alongside genetic therapies could be transformative. Imagine tailoring diet, light exposure, and even soundscapes to mitigate individual seizure triggers. Further research into gene-environment interactions is key to unlocking truly preventative epilepsy strategies.
Editor: MedTechNews.Uk
Thank you to our Sponsor Esdebe
This is a great overview. With the rise of personalized medicine, could advances in metabolomics provide further insights into individual responses to AEDs, potentially optimizing drug selection and dosage for better seizure control?
Thank you! That’s an insightful question. Metabolomics certainly holds tremendous potential. Combining metabolomic data with genetic and clinical information could lead to predictive models for AED response, allowing for truly personalized treatment strategies. Exploring these avenues is critical for improving outcomes in epilepsy.
Editor: MedTechNews.Uk
Thank you to our Sponsor Esdebe
Given the complexity of epileptogenesis involving various cellular interactions, could further investigation into the specific roles of diverse astrocyte subtypes provide more targeted therapeutic strategies?
That’s a fantastic point! The heterogeneity of astrocytes is often overlooked. Diving deeper into the specific functions of different astrocyte subtypes, especially in relation to neuronal circuits, could reveal novel targets. Imagine astrocyte-specific therapies alongside existing treatments! It’s a very promising area for future research.
Editor: MedTechNews.Uk
Thank you to our Sponsor Esdebe
Given the heterogeneity of epilepsy and the potential for personalized medicine, what role might advanced computational modeling play in predicting individual patient responses to various treatment modalities?
That’s an excellent question! Advanced computational modeling has the potential to revolutionize personalized medicine for epilepsy. By integrating diverse datasets (genomics, imaging, clinical history), we can potentially predict treatment responses and optimize therapeutic strategies for individual patients. This could lead to more effective seizure control and fewer side effects. It will be an exciting area to watch!
Editor: MedTechNews.Uk
Thank you to our Sponsor Esdebe