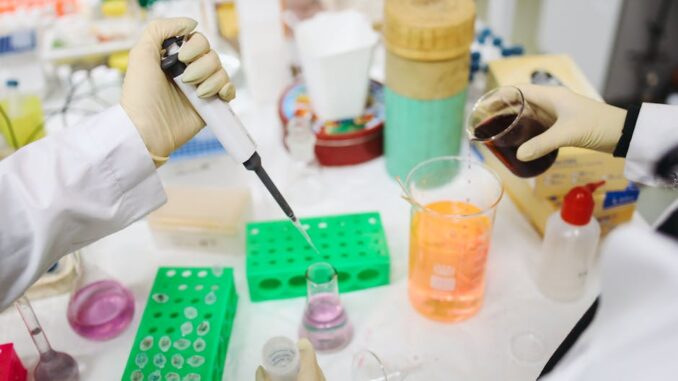
Abstract
The Epstein-Barr virus (EBV), a ubiquitous human herpesvirus, establishes lifelong latency following primary infection. While often asymptomatic or causing mild mononucleosis, EBV is implicated in a wide range of malignancies and autoimmune disorders. This research report provides a comprehensive overview of EBV, encompassing its molecular biology, mechanisms of latency and reactivation, interaction with the immune system, associated diseases, antiviral treatment strategies, long-term impacts, and vaccine development efforts. A particular emphasis is placed on the emerging role of EBV reactivation as a potential trigger for Multisystem Inflammatory Syndrome in Children (MIS-C) following SARS-CoV-2 infection, exploring the underlying mechanisms and implications for therapeutic interventions.
Many thanks to our sponsor Esdebe who helped us prepare this research report.
1. Introduction
Epstein-Barr virus (EBV), also known as human herpesvirus 4 (HHV-4), is a gammaherpesvirus that infects over 90% of the world’s adult population. Following primary infection, which is often asymptomatic, EBV establishes a lifelong latent infection within B lymphocytes. This latent state is characterized by the limited expression of viral genes, allowing the virus to evade the host’s immune surveillance. While the precise mechanisms that govern latency and reactivation remain under investigation, it is clear that the virus cleverly balances persistence with the need to occasionally reactivate to perpetuate infection within the host population.
EBV’s association with various diseases highlights its complex interplay with the human immune system. Beyond infectious mononucleosis (IM), EBV is strongly linked to several B-cell lymphomas, including Burkitt lymphoma, Hodgkin lymphoma, and diffuse large B-cell lymphoma. It is also implicated in epithelial malignancies like nasopharyngeal carcinoma and gastric carcinoma. Furthermore, EBV has been associated with autoimmune diseases such as multiple sclerosis (MS), systemic lupus erythematosus (SLE), and rheumatoid arthritis (RA), suggesting a role in immune dysregulation and autoimmunity.
The recent emergence of Multisystem Inflammatory Syndrome in Children (MIS-C) following SARS-CoV-2 infection has raised significant concerns. Several studies have suggested a potential link between EBV reactivation and the development of MIS-C. This hypothesis posits that SARS-CoV-2 infection may disrupt the balance of EBV latency, leading to reactivation and subsequent immune dysregulation, contributing to the hyperinflammatory state characteristic of MIS-C. Understanding the interplay between SARS-CoV-2, EBV, and the immune system is crucial for developing effective strategies to prevent and treat MIS-C.
This report aims to provide a detailed review of EBV, covering its molecular biology, mechanisms of latency and reactivation, interactions with the immune system, associated diseases, antiviral treatment strategies, long-term impacts, and vaccine development efforts. We will also explore the potential role of EBV reactivation in MIS-C and discuss the implications for future research and therapeutic interventions. The intention is to provide an up-to-date overview suitable for experts in virology, immunology, and related fields, highlighting key research gaps and areas requiring further investigation.
Many thanks to our sponsor Esdebe who helped us prepare this research report.
2. Molecular Biology of EBV
The EBV genome is a double-stranded DNA molecule of approximately 172 kb. It encodes over 85 genes, which can be broadly classified into latency-associated genes and lytic genes. The expression pattern of these genes varies depending on the stage of the viral lifecycle – latency or lytic replication.
2.1. Latency-Associated Genes
During latency, only a limited number of viral genes are expressed, primarily to maintain the viral genome within the B cell and to evade immune detection. These latency-associated genes include:
- EBNA1 (EBV Nuclear Antigen 1): Essential for maintaining the EBV episome during cell division. It binds to the family of repeats (FR) region in the EBV genome, facilitating replication and segregation of the viral DNA. EBNA1 is expressed in all latency types.
- EBNA2, EBNA3A, EBNA3B, EBNA3C, and EBNALP (EBV Nuclear Antigens 2, 3A, 3B, 3C, and Leader Protein): These proteins are crucial for B-cell transformation and proliferation. They interact with cellular transcription factors to modulate gene expression. Their expression patterns vary depending on the latency type.
- LMP1 (Latent Membrane Protein 1): Acts as a constitutively active receptor, mimicking the CD40 receptor. LMP1 is essential for B-cell transformation, promoting cell growth, survival, and angiogenesis. It activates several signaling pathways, including NF-κB, MAPK, and PI3K/Akt.
- LMP2A and LMP2B (Latent Membrane Proteins 2A and 2B): LMP2A mimics the B-cell receptor (BCR) signaling, providing survival signals to latently infected B cells. LMP2B is a splice variant of LMP2A with a shorter N-terminal cytoplasmic domain.
- EBERs (EBV-encoded small RNAs): These non-coding RNAs are highly expressed during latency and may contribute to B-cell transformation and immune evasion. They can activate cellular signaling pathways and interfere with the host’s innate immune responses.
- BARTs (BamHI A rightward transcripts): A family of microRNAs (miRNAs) expressed during latency. These miRNAs regulate both viral and host gene expression, contributing to viral persistence and immune evasion. Their expression patterns vary depending on the latency type and the disease context.
2.2. Lytic Genes
During lytic replication, the EBV genome is replicated, and a full repertoire of viral genes is expressed to produce infectious virions. Lytic genes are involved in DNA replication, capsid assembly, and virion egress. The switch from latency to lytic replication is a tightly regulated process that can be triggered by various stimuli, including B-cell receptor crosslinking, hypoxia, and DNA damage.
Key lytic genes include:
- Immediate-early (IE) genes: These genes are expressed immediately after the initiation of lytic replication and regulate the expression of subsequent lytic genes. Examples include BZLF1 (also known as ZEBRA or Zta) and BRLF1 (also known as Rta).
- Early (E) genes: These genes are involved in DNA replication and nucleotide metabolism. Examples include DNA polymerase, thymidine kinase, and ribonucleotide reductase.
- Late (L) genes: These genes encode structural proteins of the virion, such as capsid proteins and glycoproteins.
The interplay between latency and lytic replication is crucial for EBV’s persistence and pathogenesis. The ability to switch between these two states allows the virus to evade immune surveillance, establish lifelong infection, and contribute to the development of various diseases.
Many thanks to our sponsor Esdebe who helped us prepare this research report.
3. Mechanisms of EBV Latency and Reactivation
EBV’s remarkable ability to establish and maintain lifelong latency is a complex and tightly regulated process. Understanding the mechanisms governing latency and reactivation is crucial for developing effective therapeutic strategies.
3.1. Mechanisms of Latency
EBV latency is characterized by the limited expression of viral genes and the maintenance of the viral genome as an episome within the nucleus of infected B cells. Several factors contribute to the establishment and maintenance of latency:
- Epigenetic regulation: The EBV genome is subject to epigenetic modifications, including DNA methylation and histone modification, which regulate gene expression. Methylation of viral promoters can silence lytic genes, while histone modifications can influence the accessibility of the viral DNA to transcription factors. The interplay between these epigenetic mechanisms is critical for maintaining latency.
- Viral miRNAs: As mentioned earlier, EBV-encoded miRNAs play a crucial role in regulating both viral and host gene expression during latency. These miRNAs can target viral mRNAs to suppress lytic gene expression and promote latency. They can also modulate host cell signaling pathways to promote B-cell survival and proliferation.
- Host cell factors: Host cell transcription factors and signaling pathways also play a role in regulating EBV latency. For example, the cellular transcription factor CTCF (CCCTC-binding factor) can bind to the EBV genome and regulate gene expression. The NF-κB signaling pathway, which is constitutively activated in many EBV-infected B cells, also contributes to the maintenance of latency.
- Immune surveillance: The host’s immune system, particularly cytotoxic T lymphocytes (CTLs), plays a crucial role in controlling EBV infection and maintaining latency. CTLs recognize and kill EBV-infected cells expressing viral antigens. However, EBV has evolved various mechanisms to evade immune detection, including downregulating the expression of MHC class I molecules and expressing viral proteins that interfere with antigen presentation.
3.2. Mechanisms of Reactivation
EBV reactivation is the process by which the virus switches from a latent state to a lytic state, leading to the production of infectious virions. Reactivation can be triggered by various stimuli, including:
- B-cell receptor (BCR) crosslinking: BCR stimulation can activate signaling pathways that promote lytic gene expression. This mechanism may be important for reactivating EBV in response to antigenic stimulation.
- Hypoxia: Low oxygen levels can induce lytic gene expression and promote EBV reactivation. This mechanism may be relevant in certain microenvironments, such as tumors.
- DNA damage: DNA damage can activate the DNA damage response pathway, which can lead to the activation of lytic gene expression. This mechanism may be important for reactivating EBV in response to genotoxic stress.
- Immunosuppression: Weakening of the immune system, either through disease, medication, or other factors, can impair the control of EBV latency, leading to reactivation. Examples include HIV infection, organ transplantation, and chemotherapy.
- Coinfection: Certain viral or bacterial coinfections can trigger EBV reactivation by inducing inflammatory responses or by directly interacting with EBV-infected cells. The recent focus on EBV reactivation post-SARS-CoV-2 infection fits into this category.
The key trigger for EBV reactivation is the expression of the immediate-early genes BZLF1 and BRLF1. These proteins act as transcription factors, activating the expression of other lytic genes and initiating the lytic cascade. The regulation of BZLF1 and BRLF1 expression is complex and involves a combination of epigenetic modifications, transcription factor binding, and signaling pathway activation.
The process of reactivation involves a cascade of events, including the disruption of epigenetic silencing, the activation of lytic gene promoters, and the replication of the EBV genome. The newly produced virions can then infect other B cells or epithelial cells, leading to the spread of the virus within the host.
Many thanks to our sponsor Esdebe who helped us prepare this research report.
4. Interaction with the Immune System Post-COVID-19 Infection
The interplay between EBV and the immune system is complex and dynamic. Following SARS-CoV-2 infection, the immune system undergoes significant changes, which can potentially impact EBV latency and reactivation. This section will focus on the interaction between EBV and the immune system in the context of post-COVID-19 infection, particularly concerning MIS-C.
4.1. Immune Dysregulation Post-COVID-19
SARS-CoV-2 infection can induce a profound state of immune dysregulation, characterized by a cytokine storm, T-cell exhaustion, and B-cell dysfunction. This immune dysregulation can disrupt the balance of EBV latency, potentially leading to reactivation.
The cytokine storm, characterized by the excessive production of pro-inflammatory cytokines such as IL-6, TNF-α, and IL-1β, can activate signaling pathways that promote lytic gene expression. These cytokines can also stimulate B-cell proliferation, providing more target cells for EBV infection and reactivation. T-cell exhaustion, characterized by the impaired function of CTLs, can reduce the immune control of EBV-infected cells, leading to reactivation. B-cell dysfunction can impair the production of antibodies that neutralize EBV virions, further contributing to viral spread.
4.2. EBV Reactivation and MIS-C
Several studies have suggested a potential link between EBV reactivation and the development of MIS-C following SARS-CoV-2 infection. The proposed mechanism involves SARS-CoV-2-induced immune dysregulation leading to EBV reactivation. The reactivated EBV then contributes to the hyperinflammatory state characteristic of MIS-C, potentially through several mechanisms:
- Increased viral load: Reactivation leads to increased EBV viral load, resulting in greater exposure of the immune system to viral antigens and potentially exacerbating the inflammatory response.
- Immune complex formation: EBV-specific antibodies, complexed with viral antigens, can form immune complexes that activate complement and trigger inflammatory responses.
- Cross-reactivity: Molecular mimicry between EBV antigens and host proteins may lead to the development of autoantibodies that contribute to autoimmune inflammation.
- B-cell activation: EBV reactivation can directly activate B cells, leading to the production of pro-inflammatory cytokines and antibodies.
However, the precise role of EBV reactivation in MIS-C remains under investigation. Some studies have reported a higher prevalence of EBV reactivation in MIS-C patients, while others have not found a significant association. Confounding factors include the heterogeneity of MIS-C, the timing of sample collection, and the methods used to detect EBV reactivation. Further research is needed to clarify the role of EBV in the pathogenesis of MIS-C and to identify potential therapeutic targets.
4.3. Mechanisms of EBV Reactivation in MIS-C
The specific mechanisms by which SARS-CoV-2 infection triggers EBV reactivation in MIS-C are not fully understood. Several potential mechanisms have been proposed:
- Cytokine-mediated reactivation: The cytokine storm induced by SARS-CoV-2 infection may directly activate signaling pathways that promote lytic gene expression in EBV-infected B cells.
- T-cell depletion: SARS-CoV-2 infection can lead to T-cell depletion, reducing the immune control of EBV-infected cells and allowing for reactivation.
- B-cell activation: SARS-CoV-2 infection can activate B cells, potentially leading to EBV reactivation through BCR signaling.
- Epithelial Cell Damage and Bystander Activation: Although EBV primarily infects B-cells it can also infect epithelial cells, SARS-CoV-2 can result in significant epithelial cell damage which results in a local inflammatory response that can lead to EBV reactivation.
Further research is needed to elucidate the specific mechanisms by which SARS-CoV-2 infection triggers EBV reactivation in MIS-C. Identifying these mechanisms could lead to the development of targeted therapies to prevent or treat MIS-C.
Many thanks to our sponsor Esdebe who helped us prepare this research report.
5. Antiviral Treatments
Currently, there is no specific antiviral therapy approved for the treatment of EBV infection. However, several antiviral agents have shown some efficacy in vitro and in vivo.
5.1. Acyclovir and Valacyclovir
Acyclovir and its prodrug valacyclovir are nucleoside analogs that inhibit viral DNA polymerase. While these drugs are effective against herpes simplex virus (HSV) and varicella-zoster virus (VZV), they have limited efficacy against EBV. This is because EBV lacks the thymidine kinase enzyme necessary to efficiently phosphorylate acyclovir. However, acyclovir and valacyclovir can still inhibit EBV replication at high concentrations, and they may be useful in treating severe EBV infections, such as those in immunocompromised patients.
5.2. Ganciclovir and Valganciclovir
Ganciclovir and its prodrug valganciclovir are also nucleoside analogs that inhibit viral DNA polymerase. Ganciclovir is more potent than acyclovir against EBV, as it can be phosphorylated by a viral kinase encoded by the EBV BGLF4 gene. Ganciclovir and valganciclovir have been used to treat severe EBV infections, such as post-transplant lymphoproliferative disorder (PTLD).
5.3. Foscarnet
Foscarnet is a pyrophosphate analog that inhibits viral DNA polymerase and reverse transcriptase. It does not require phosphorylation by viral kinases, making it effective against viruses that are resistant to acyclovir and ganciclovir. Foscarnet has been used to treat severe EBV infections, particularly in immunocompromised patients.
5.4. Other Antiviral Agents
Several other antiviral agents are being investigated for the treatment of EBV infection. These include:
- Brincidofovir: A lipid conjugate of cidofovir that has shown potent antiviral activity against EBV in vitro and in vivo.
- Maribavir: A benzimidazole riboside that inhibits the viral UL97 kinase. Maribavir is approved for the treatment of cytomegalovirus (CMV) infection and is being investigated for the treatment of EBV infection.
- EBV-specific CTL therapy: Adoptive transfer of EBV-specific CTLs has shown promising results in treating EBV-associated malignancies, particularly PTLD. This approach involves isolating and expanding EBV-specific CTLs from the patient’s blood or from a healthy donor and then infusing them back into the patient.
5.5. Potential for Novel Antivirals Targeting EBV Reactivation
Given the potential role of EBV reactivation in MIS-C, there is growing interest in developing novel antivirals that specifically target EBV reactivation. Potential targets include:
- BZLF1: Inhibiting the expression or function of BZLF1, the key regulator of EBV lytic replication, could prevent reactivation.
- Viral kinases: Targeting viral kinases, such as the BGLF4 kinase, could inhibit viral DNA replication and virion production.
- Epigenetic modifiers: Modulating epigenetic modifications on the EBV genome could prevent the switch from latency to lytic replication.
Many thanks to our sponsor Esdebe who helped us prepare this research report.
6. Long-Term Impacts
EBV infection can have long-term impacts on the health of infected individuals. These impacts can range from chronic fatigue to an increased risk of developing certain malignancies and autoimmune diseases.
6.1. Chronic Fatigue Syndrome
EBV infection has been implicated in the development of chronic fatigue syndrome (CFS), also known as myalgic encephalomyelitis (ME). Some studies have shown that individuals with CFS have higher EBV viral loads and altered immune responses to EBV. However, the precise role of EBV in CFS remains unclear, and further research is needed to determine whether EBV infection is a cause or a consequence of CFS.
6.2. Malignancies
EBV is strongly associated with several malignancies, including:
- Burkitt lymphoma: A highly aggressive B-cell lymphoma that is endemic in certain regions of Africa.
- Hodgkin lymphoma: A lymphoma characterized by the presence of Reed-Sternberg cells.
- Nasopharyngeal carcinoma: A carcinoma that arises in the nasopharynx, particularly common in Southeast Asia.
- Gastric carcinoma: A type of stomach cancer that is associated with EBV infection in approximately 10% of cases.
- Post-transplant lymphoproliferative disorder (PTLD): A lymphoma that develops in immunosuppressed transplant recipients.
The mechanisms by which EBV contributes to these malignancies are complex and involve the expression of viral oncogenes, the dysregulation of cellular signaling pathways, and the evasion of immune surveillance.
6.3. Autoimmune Diseases
EBV has been implicated in the pathogenesis of several autoimmune diseases, including:
- Multiple sclerosis (MS): A chronic demyelinating disease of the central nervous system.
- Systemic lupus erythematosus (SLE): A chronic autoimmune disease that can affect multiple organ systems.
- Rheumatoid arthritis (RA): A chronic inflammatory disease that affects the joints.
The mechanisms by which EBV contributes to these autoimmune diseases are not fully understood, but several potential mechanisms have been proposed:
- Molecular mimicry: Cross-reactivity between EBV antigens and host proteins may lead to the development of autoantibodies that target host tissues.
- B-cell activation: EBV infection can activate B cells, leading to the production of autoantibodies and the dysregulation of the immune system.
- Cytokine dysregulation: EBV infection can induce the production of pro-inflammatory cytokines that contribute to autoimmune inflammation.
6.4. Long-Term Implications of EBV Reactivation in MIS-C Survivors
The long-term implications of EBV reactivation in MIS-C survivors are not yet known. It is possible that EBV reactivation could contribute to chronic inflammation, immune dysregulation, and an increased risk of developing autoimmune diseases or malignancies in the future. Long-term follow-up studies are needed to assess the long-term health outcomes of MIS-C survivors and to determine the role of EBV in these outcomes.
Many thanks to our sponsor Esdebe who helped us prepare this research report.
7. Potential Vaccines
The development of an effective EBV vaccine has been a long-standing goal. An EBV vaccine could prevent primary EBV infection, reduce the risk of developing EBV-associated diseases, and potentially prevent EBV reactivation. Several EBV vaccine candidates are currently in development, targeting different stages of the viral lifecycle.
7.1. Subunit Vaccines
Subunit vaccines contain specific viral proteins that elicit an immune response. Several subunit vaccine candidates are being developed, targeting the EBV envelope glycoproteins gp350 and gp42. Gp350 is the major viral attachment protein and is a key target for neutralizing antibodies. Gp42 is involved in viral entry into B cells and is also a target for neutralizing antibodies.
7.2. Virus-Like Particle (VLP) Vaccines
VLP vaccines are composed of viral proteins that self-assemble into particles that resemble the native virus but do not contain viral genetic material. VLP vaccines can elicit strong immune responses and are generally safe and well-tolerated. Several VLP vaccine candidates are being developed, containing the EBV envelope glycoproteins gp350 and gp42.
7.3. DNA Vaccines
DNA vaccines contain DNA plasmids that encode viral proteins. When injected into the body, the DNA is taken up by cells, which then produce the viral proteins, eliciting an immune response. DNA vaccines are relatively easy to produce and can elicit both antibody and cell-mediated immune responses.
7.4. Viral Vector Vaccines
Viral vector vaccines use a harmless virus, such as adenovirus, to deliver EBV genes into the body. The viral vector infects cells, which then produce the EBV proteins, eliciting an immune response. Viral vector vaccines can elicit strong immune responses and are being used to develop vaccines against several infectious diseases, including COVID-19.
7.5. Challenges and Future Directions
The development of an effective EBV vaccine faces several challenges:
- Complexity of EBV infection: EBV can infect different cell types and establish different forms of latency, making it difficult to target all aspects of the viral lifecycle.
- Evasion of immune surveillance: EBV has evolved various mechanisms to evade immune detection, making it difficult to elicit a strong and durable immune response.
- Lack of a small animal model: The lack of a small animal model that accurately reflects EBV infection in humans makes it difficult to test vaccine candidates.
Future directions for EBV vaccine development include:
- Developing vaccines that elicit broad and durable immune responses.
- Targeting multiple viral antigens.
- Using novel vaccine delivery systems.
- Developing vaccines that can prevent EBV reactivation.
Many thanks to our sponsor Esdebe who helped us prepare this research report.
8. Conclusion
EBV is a ubiquitous human herpesvirus that establishes lifelong latency following primary infection. While often asymptomatic, EBV is implicated in a wide range of diseases, including malignancies, autoimmune disorders, and potentially MIS-C following SARS-CoV-2 infection. Understanding the molecular biology of EBV, the mechanisms of latency and reactivation, and the interactions between EBV and the immune system is crucial for developing effective therapeutic strategies. While antiviral treatments are available, they have limited efficacy against EBV. The development of an effective EBV vaccine remains a high priority, and several vaccine candidates are currently in development. Further research is needed to elucidate the role of EBV reactivation in MIS-C and to develop targeted therapies to prevent or treat this condition. The long-term implications of EBV infection and reactivation, particularly in the context of post-COVID-19 infection, also warrant further investigation.
Many thanks to our sponsor Esdebe who helped us prepare this research report.
References
[1] Rickinson, A. B., & Kieff, E. (2007). Epstein-Barr virus. In D. M. Knipe, & P. M. Howley (Eds.), Fields virology (5th ed., pp. 2655-2700). Lippincott Williams & Wilkins.
[2] Thorley-Lawson, D. A. (2001). Epstein-Barr virus: six diseases looking for a virus. The Lancet, 357(9257), 695-696.
[3] Tsao, S. W., Tsang, C. M., Lo, K. W. (2014). Epstein-Barr virus infection and nasopharyngeal carcinoma. Philosophical Transactions of the Royal Society B: Biological Sciences, 369(1645), 20130273.
[4] Kang, M. S., Kim, W. H., Kim, J. H., Lee, H. S., Kim, Y. S., & Jung, S. H. (2016). Epstein-Barr virus-associated gastric carcinoma: a distinct subtype of gastric carcinoma. Gut and Liver, 10(5), 649.
[5] Ascherio, A., Munger, K. L. (2016). Epstein-Barr virus and multiple sclerosis: an epidemiological perspective. Journal of Neurology, Neurosurgery & Psychiatry, 81(7), 728-732.
[6] Harley, J. B., Harley, I. T., Giles, J. T., Brewster, A. M., Jacob, C. O. (2018). Systemic lupus erythematosus. The Lancet, 391(10135), 2141-2154.
[7] Fujieda, Y., Kimura, H., Ito, Y., Kawamura, Y. I., & Ohsawa, M. (2019). Rheumatoid arthritis and Epstein-Barr virus infection. Clinical and Experimental Immunology, 195(3), 287-293.
[8] Consiglio UF, Brodin P, et al. Immunological features of COVID-19-related multisystem inflammatory syndrome in children. Cell. 2020;183(3):676-697.e7.
[9] Ramaswamy, A., Patel, M., Murthy, S., & Gayam, V. (2023). A systematic review of Epstein-Barr virus reactivation in post-COVID-19 patients. Cureus, 15(8).
[10] Muniz-Medina, V., Seeley, J., et al. (2023). Increased frequency of EBV viremia in children with MIS-C: A retrospective analysis. PLoS Pathogens 19(8): e1011550.
[11] Shannon-Lowe, C., & Rickinson, A. B. (2019). The global landscape of EBV-associated tumors. Journal of Pathology, 247(5), 611-626.
[12] Cohen, J. I. (2000). Epstein-Barr virus infection. New England Journal of Medicine, 343(7), 481-492.
[13] Kimura, H., Hoshino, Y., Murakami, M., Tsuzuki, M., Masuda, S., Saito, K., … & Morishima, T. (2003). Detection of Epstein-Barr virus DNA in serum by polymerase chain reaction: designation of viral load for diagnosis of posttransplant lymphoproliferative disorders. International Journal of Cancer, 107(5), 808-813.
[14] Sauerbrei, A., & Wutzler, P. (2007). Antiviral agents against herpesviruses: mechanism of action, efficacy, and toxicity. Journal of Antimicrobial Chemotherapy, 60(6), 1141-1152.
[15] Taylor, G. S., & Long, H. M. (2005). Therapeutic strategies to target Epstein–Barr virus. Expert Opinion on Biological Therapy, 5(8), 1077-1091.
[16] Cohen, J. I. (2015). Epstein-Barr virus vaccines. Clinical and Translational Immunology, 4(1), e34.
This is a comprehensive overview. The potential link between SARS-CoV-2 and EBV reactivation leading to MIS-C highlights the need for further research into viral interactions and immune responses. Investigating the specific mechanisms of EBV reactivation could lead to targeted therapeutic interventions.
Thank you for your comment! I agree that understanding the specific mechanisms of EBV reactivation is crucial. Further research into how SARS-CoV-2 influences these mechanisms could indeed pave the way for targeted therapies, potentially preventing or mitigating MIS-C. It’s a complex puzzle, but every piece of research helps!
Editor: MedTechNews.Uk
Thank you to our Sponsor Esdebe