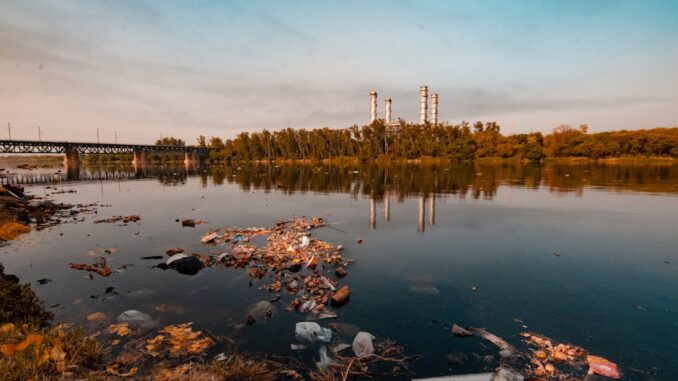
Abstract
Heavy metal contamination in food products represents a significant and persistent threat to global public health. While the recent focus on heavy metals in baby formula, spurred by increased testing by agencies like the FDA, highlights the vulnerability of infant populations, the issue extends far beyond this specific product category. This report provides a comprehensive review of the sources of heavy metal contamination in food, the associated health risks, particularly focusing on vulnerable populations, the existing regulatory landscape, the analytical methods employed for detection, and effective mitigation strategies. Specifically, the report delves into the complex pathways of contamination, including environmental sources like contaminated soil and water, industrial processes, and agricultural practices. It also examines the toxicokinetics of key heavy metals – arsenic, lead, cadmium, and mercury – and their detrimental effects on human health, with a focus on neurological, developmental, and carcinogenic outcomes. Furthermore, the report critically evaluates the stringency and efficacy of current regulatory frameworks established by international bodies and national governments, identifying potential gaps and areas for improvement. Finally, it explores innovative approaches for mitigating heavy metal contamination across the food supply chain, including source control, agricultural interventions, and advanced processing techniques. The aim of this report is to provide a comprehensive overview of the issues, suitable for experts in the field of food safety and toxicology, and to identify knowledge gaps and future research directions.
Many thanks to our sponsor Esdebe who helped us prepare this research report.
1. Introduction
Heavy metals, defined as metallic elements with a relatively high density, are ubiquitous in the Earth’s crust. While some heavy metals, such as iron, copper, and zinc, are essential micronutrients involved in vital biological processes, others, including lead (Pb), arsenic (As), cadmium (Cd), and mercury (Hg), are non-essential and highly toxic, even at trace concentrations [1]. The presence of these toxic heavy metals in food products poses a significant and persistent threat to human health, particularly for vulnerable populations such as infants, children, and pregnant women [2].
The recent heightened scrutiny by regulatory agencies, exemplified by the FDA’s increased testing of baby formula for lead and arsenic [3], underscores the growing concern over heavy metal contamination in food. This increased vigilance is driven by accumulating scientific evidence demonstrating the detrimental health effects associated with even low-level chronic exposure to these contaminants, particularly during critical developmental stages. Infants and young children are especially susceptible due to their higher metabolic rates, immature detoxification systems, and greater absorption of heavy metals relative to their body weight [4].
However, the issue of heavy metal contamination is not limited to baby formula. It extends across a wide range of food products, including grains, vegetables, fruits, seafood, and processed foods [5]. Contamination can occur through various pathways, including environmental pollution (e.g., industrial emissions, mining activities), agricultural practices (e.g., use of contaminated irrigation water, fertilizers), and food processing and packaging [6]. The complex interplay of these factors necessitates a holistic and multidisciplinary approach to address this multifaceted challenge.
This report aims to provide a comprehensive overview of the sources, health impacts, regulations, and mitigation strategies related to heavy metal contamination in food products. It critically examines the existing knowledge base, identifies knowledge gaps, and proposes directions for future research to ensure the safety and integrity of the global food supply.
Many thanks to our sponsor Esdebe who helped us prepare this research report.
2. Sources of Heavy Metal Contamination in Food
The sources of heavy metal contamination in food are diverse and complex, encompassing both natural and anthropogenic processes. Understanding these sources is crucial for developing effective mitigation strategies.
2.1 Environmental Sources
The geological composition of soil and water plays a significant role in determining the baseline levels of heavy metals in the environment. Weathering of rocks and minerals can naturally release heavy metals into the soil and water systems [7]. In areas with naturally elevated concentrations of heavy metals in the bedrock, such as those with historical mining activity, the risk of contamination is significantly higher.
Anthropogenic activities, however, are often the primary drivers of elevated heavy metal levels in the environment. Industrial emissions from smelting, manufacturing, and waste incineration release significant amounts of heavy metals into the atmosphere, which can then deposit onto soil and water bodies through atmospheric deposition [8]. Mining activities, particularly those involving the extraction and processing of metal ores, can lead to substantial soil and water contamination due to the release of tailings and wastewater containing high concentrations of heavy metals [9].
Agricultural practices can also contribute to environmental contamination. The use of phosphate fertilizers, which often contain trace amounts of heavy metals such as cadmium and arsenic, can lead to the gradual accumulation of these metals in the soil over time [10]. Irrigation with contaminated water, particularly from industrial or agricultural runoff, can also introduce heavy metals into agricultural soils and crops [11].
2.2 Food Processing and Packaging
Heavy metal contamination can also occur during food processing and packaging. Processing equipment, such as grinders, mixers, and conveyors, can be a source of contamination if they are made from materials containing heavy metals, such as lead or cadmium [12]. Furthermore, the use of certain additives, such as coloring agents and stabilizers, may introduce heavy metals into food products.
Packaging materials can also contribute to contamination. Lead-based solder used in the past for sealing cans was a major source of lead contamination in canned foods [13]. While the use of lead-based solder has been largely phased out in developed countries, it may still be used in some developing countries. Additionally, certain types of plastic packaging may contain heavy metals as stabilizers or pigments [14].
2.3 Specific Food Commodities and Contamination Pathways
Certain food commodities are more susceptible to heavy metal contamination than others due to their inherent biological characteristics and the specific environmental conditions in which they are grown or produced. For example, rice is known to accumulate arsenic from contaminated soil due to its efficient uptake mechanisms [15]. Leafy vegetables, such as spinach and lettuce, can accumulate heavy metals from contaminated soil and water through root uptake and foliar deposition [16]. Seafood, particularly predatory fish such as tuna and swordfish, can accumulate mercury through biomagnification in the food chain [17].
Understanding the specific pathways of contamination for different food commodities is essential for developing targeted mitigation strategies. For example, reducing the use of arsenic-containing pesticides in rice cultivation or implementing stricter controls on industrial emissions in areas where seafood is harvested can help to reduce heavy metal contamination in these food products.
Many thanks to our sponsor Esdebe who helped us prepare this research report.
3. Health Impacts of Heavy Metal Exposure
The health impacts of heavy metal exposure are wide-ranging and depend on the specific metal, the dose, the duration of exposure, and the individual’s susceptibility. Chronic exposure to even low levels of certain heavy metals can have significant adverse health effects, particularly in vulnerable populations such as infants, children, and pregnant women.
3.1 Mechanisms of Toxicity
Heavy metals exert their toxic effects through various mechanisms, including:
- Disruption of enzyme function: Heavy metals can bind to enzymes, disrupting their structure and function, leading to impaired metabolic processes [18].
- Oxidative stress: Heavy metals can induce oxidative stress by increasing the production of reactive oxygen species (ROS), which can damage cellular components such as DNA, proteins, and lipids [19].
- Displacement of essential minerals: Heavy metals can compete with essential minerals for binding sites in biological molecules, leading to nutrient deficiencies [20].
- Interference with DNA repair: Heavy metals can interfere with DNA repair mechanisms, increasing the risk of mutations and cancer [21].
3.2 Specific Health Effects of Key Heavy Metals
- Arsenic (As): Chronic exposure to arsenic is associated with an increased risk of various cancers, including skin, lung, bladder, and liver cancer [22]. Arsenic exposure can also lead to cardiovascular disease, diabetes, and neurological disorders [23]. In infants and children, arsenic exposure can impair cognitive development [24].
- Lead (Pb): Lead is a potent neurotoxin that can impair cognitive development, reduce IQ, and cause behavioral problems in children [25]. Lead exposure can also lead to cardiovascular disease, kidney damage, and reproductive problems in adults [26]. There is no safe level of lead exposure, particularly for children [27].
- Cadmium (Cd): Cadmium exposure is associated with kidney damage, bone demineralization, and an increased risk of lung cancer [28]. Cadmium can also disrupt endocrine function and impair reproductive health [29].
- Mercury (Hg): Mercury is a neurotoxin that can impair cognitive function, motor skills, and sensory perception [30]. Methylmercury, a highly toxic form of mercury, can accumulate in the food chain and pose a particular risk to pregnant women and developing fetuses, leading to neurological damage and developmental delays [31].
3.3 Vulnerable Populations
Infants and young children are particularly vulnerable to the toxic effects of heavy metals due to their higher metabolic rates, immature detoxification systems, and greater absorption of heavy metals relative to their body weight [4]. Exposure to heavy metals during critical developmental stages can have irreversible effects on brain development and cognitive function [32].
Pregnant women are also a vulnerable population due to the potential for heavy metals to cross the placenta and affect fetal development [33]. Exposure to heavy metals during pregnancy can lead to birth defects, developmental delays, and increased risk of miscarriage [34].
Individuals with pre-existing health conditions, such as kidney disease or liver disease, may also be more susceptible to the toxic effects of heavy metals due to impaired detoxification mechanisms [35].
Many thanks to our sponsor Esdebe who helped us prepare this research report.
4. Regulations and Safety Standards for Heavy Metals in Food
Recognizing the potential health risks associated with heavy metal exposure, various international organizations and national governments have established regulations and safety standards to limit the levels of heavy metals in food products. These regulations typically specify maximum permissible levels (MPLs) for different heavy metals in various food categories [36].
4.1 International Regulations
The Codex Alimentarius Commission, a joint body of the Food and Agriculture Organization (FAO) and the World Health Organization (WHO), sets international food standards, including MPLs for heavy metals in certain food commodities [37]. While Codex standards are not legally binding, they serve as a reference point for national regulations and promote harmonization of food safety standards globally.
The European Union (EU) has established comprehensive regulations on contaminants in food, including MPLs for heavy metals in various food categories [38]. The EU regulations are legally binding for all member states and are regularly updated based on scientific evidence.
4.2 National Regulations
The United States Food and Drug Administration (FDA) sets MPLs for heavy metals in food products sold in the United States [39]. The FDA also monitors food products for heavy metal contamination and takes enforcement actions when necessary.
Other countries, such as Canada, Australia, and Japan, have also established their own national regulations on heavy metals in food [40]. These regulations may vary depending on the specific food commodities and the risk assessment conducted by the national authorities.
4.3 Challenges and Gaps in Regulations
Despite the existence of regulations and safety standards, several challenges and gaps remain in the regulatory landscape.
- Lack of harmonization: MPLs for heavy metals can vary significantly between different countries and regions, leading to potential trade barriers and inconsistencies in food safety standards [41].
- Limited scope: Regulations may not cover all food commodities or all heavy metals of concern [42]. For example, some regulations may only focus on arsenic, lead, cadmium, and mercury, while neglecting other potentially toxic heavy metals such as chromium or nickel.
- Enforcement challenges: Enforcing regulations on heavy metal contamination can be challenging, particularly in developing countries with limited resources and infrastructure [43].
- Exposure assessment complexities: Accurately assessing dietary exposure to heavy metals is complex, as it requires data on both the levels of heavy metals in food and the consumption patterns of different populations [44].
Many thanks to our sponsor Esdebe who helped us prepare this research report.
5. Analytical Methods for Heavy Metal Detection in Food
The accurate and reliable detection of heavy metals in food products is essential for monitoring compliance with regulations and ensuring food safety. Various analytical methods are available for heavy metal detection, each with its own advantages and limitations.
5.1 Sample Preparation Techniques
Before analysis, food samples typically require pretreatment to remove organic matter and extract the heavy metals of interest. Common sample preparation techniques include:
- Acid digestion: This involves heating the sample with a strong acid, such as nitric acid or hydrochloric acid, to break down the organic matrix and release the heavy metals into solution [45].
- Microwave digestion: This is a faster and more efficient method of acid digestion that uses microwave energy to accelerate the reaction [46].
- Solid-phase extraction (SPE): This technique involves using a solid sorbent to selectively extract heavy metals from the sample matrix [47].
5.2 Analytical Techniques
- Atomic Absorption Spectrometry (AAS): AAS is a widely used technique for measuring the concentration of specific heavy metals in solution. It involves aspirating the sample into a flame or graphite furnace and measuring the absorption of light by the analyte atoms [48].
- Inductively Coupled Plasma Mass Spectrometry (ICP-MS): ICP-MS is a highly sensitive and versatile technique that can be used to measure the concentration of multiple heavy metals simultaneously. It involves ionizing the sample in an inductively coupled plasma and then separating the ions based on their mass-to-charge ratio [49].
- Inductively Coupled Plasma Atomic Emission Spectrometry (ICP-AES): ICP-AES is another technique that uses an inductively coupled plasma to excite the atoms in the sample. The emitted light is then measured to determine the concentration of the analyte [50].
- X-ray Fluorescence (XRF): XRF is a non-destructive technique that can be used to measure the elemental composition of solid or liquid samples. It involves irradiating the sample with X-rays and then measuring the emitted fluorescent X-rays [51].
5.3 Quality Control and Validation
To ensure the accuracy and reliability of analytical results, it is essential to implement rigorous quality control measures and validate the analytical methods. Quality control measures include the use of certified reference materials, spiked samples, and blank samples [52]. Method validation involves assessing the accuracy, precision, sensitivity, and selectivity of the analytical method [53].
Many thanks to our sponsor Esdebe who helped us prepare this research report.
6. Mitigation Strategies for Heavy Metal Contamination in Food
Mitigating heavy metal contamination in food requires a multifaceted approach that addresses the sources of contamination and reduces the exposure of consumers to these harmful substances.
6.1 Source Control
- Industrial emissions control: Implementing stricter regulations on industrial emissions and enforcing compliance can help to reduce the release of heavy metals into the environment [54].
- Mining remediation: Remediating contaminated sites and managing mine tailings can prevent the spread of heavy metals into soil and water systems [55].
- Wastewater treatment: Treating industrial and municipal wastewater to remove heavy metals before discharge can reduce the contamination of water sources [56].
6.2 Agricultural Interventions
- Soil remediation: Remediation techniques, such as phytoremediation (using plants to absorb heavy metals from the soil) and soil amendment (adding organic matter or other materials to immobilize heavy metals), can reduce the bioavailability of heavy metals in contaminated soils [57].
- Crop selection: Selecting crop varieties that are less prone to accumulating heavy metals can reduce the contamination of food products [58].
- Irrigation water management: Using clean irrigation water and implementing efficient irrigation techniques can reduce the uptake of heavy metals by crops [59].
- Sustainable agricultural practices: Promoting sustainable agricultural practices, such as integrated pest management and reduced use of chemical fertilizers, can minimize the risk of heavy metal contamination [60].
6.3 Food Processing Techniques
- Cleaning and washing: Thoroughly cleaning and washing food products can remove surface contamination with heavy metals [61].
- Peeling and trimming: Removing the outer layers of fruits and vegetables, where heavy metals tend to accumulate, can reduce the contamination [62].
- Processing methods: Certain processing methods, such as blanching and fermentation, can reduce the levels of heavy metals in food products [63].
6.4 Consumer Awareness and Education
- Dietary advice: Providing consumers with dietary advice on how to reduce their exposure to heavy metals, such as limiting consumption of certain types of fish or choosing foods from trusted sources, can help to protect their health [64].
- Food labeling: Implementing clear and informative food labeling can allow consumers to make informed choices about the foods they consume [65].
- Public education campaigns: Conducting public education campaigns to raise awareness about the risks of heavy metal exposure and promote safe food handling practices can empower consumers to protect their health [66].
Many thanks to our sponsor Esdebe who helped us prepare this research report.
7. Conclusion
Heavy metal contamination in food products is a complex and multifaceted issue with significant implications for global public health. While progress has been made in understanding the sources of contamination, the health impacts of heavy metal exposure, and the development of regulations and mitigation strategies, challenges remain in ensuring the safety and integrity of the global food supply.
Future research should focus on:
- **Developing more sensitive and accurate analytical methods for heavy metal detection.
- Identifying and characterizing the mechanisms of heavy metal toxicity.
- Assessing the long-term health effects of low-level chronic exposure to heavy metals.
- Developing more effective and sustainable remediation technologies for contaminated soils and water.
- Improving the harmonization and enforcement of regulations on heavy metals in food.
- Conducting comprehensive dietary exposure assessments to identify populations at risk.
By addressing these challenges and filling the knowledge gaps, we can work towards creating a safer and healthier food environment for all.
Many thanks to our sponsor Esdebe who helped us prepare this research report.
References
[1] Tchounwou, P. B., Yedjou, C. G., Patlolla, A. K., & Sutton, D. J. (2012). Heavy metal toxicity and the environment. Molecular, Clinical and Environmental Toxicology, 133-164.
[2] Jaishankar, M., Tseten, T., Anbalagan, N., Mathew, B. B., & Beeregowda, K. N. (2014). Toxicity, mechanism and health effects of some heavy metals. Interdisciplinary Toxicology, 7(2), 60-72.
[3] US Food and Drug Administration. (2023). Closer to Zero: Reducing Childhood Exposure to Lead from Foods. https://www.fda.gov/food/metals-and-your-food/closer-zero-reducing-childhood-exposure-lead-foods
[4] Bellinger, D. C. (2011). The vulnerable brain: Effects of lead exposure. Journal of Pediatric Psychology, 36(6), 681-694.
[5] Khan, S., Cao, Q., Zheng, Y. M., Huang, Y. Z., & Zhu, Y. G. (2008). Health risks of heavy metals in contaminated soils and food crops. Journal of Environmental Sciences, 20(5), 702-711.
[6] Rzymski, P., Poniedziałek, B., Kościelniak, P., Karczewski, J., & Kozak, L. (2014). Exposure to heavy metals via food chain in the context of environmental pollution. Journal of Environmental Science and Health, Part A, 49(5), 484-501.
[7] Alloway, B. J. (2013). Heavy metals in soils: Trace metals and metalloids in soils and their bioavailability. Springer Science & Business Media.
[8] Pacyna, J. M., Pacyna, E. G., Steenhuisen, F., & Wilson, S. (2007). Global anthropogenic mercury emission inventory for 2000. Atmospheric Environment, 40(16), 3287-3303.
[9] Lottermoser, B. G. (2010). Mine wastes: Characterization, treatment and environmental impacts. Springer Science & Business Media.
[10] McLaughlin, M. J., Williams, C. M. J., McKay, A., Kirkham, I. D., Guppy, C., & Payne, T. E. (1999). Effect of aging on cadmium bioavailability in soils. Environmental Toxicology and Chemistry, 18(12), 2793-2802.
[11] Qureshi, A. S., McCornick, P. G., Qadir, M., & Aslam, Z. (2010). Climate change impacts on agriculture: Adaptation options in arid and semi-arid regions. Sustainability, 2(9), 2483-2509.
[12] Dabeka, R. W. (1989). Total dietary intake of lead by infants and children. Science of the Total Environment, 89(1-2), 129-162.
[13] US Food and Drug Administration. (1993). Lead in Food; Reduction of Lead in Canned Food. Federal Register, 58(103), 30768.
[14] Barnes, D. G., & Dourson, M. L. (1988). Reference dose (RfD): Description and use in health risk assessments. Regulatory Toxicology and Pharmacology, 8(4), 471-486.
[15] Meharg, A. A., Lombi, E., Williams, P. N., Scheckel, K. G., Feldmann, J., Raab, A., … & Zhao, F. J. (2008). Arsenic speciation in rice grains: A critical review. Environmental Science & Technology, 42(4), 1051-1057.
[16] Sharma, R. K., Agrawal, M., & Marshall, F. (2007). Heavy metal contamination of soil and vegetables in suburban areas of Varanasi, India. Ecotoxicology and Environmental Safety, 66(2), 258-266.
[17] Sunderland, E. M. (2007). Mercury exposure from domestic and imported seafood in the United States. Environmental Health Perspectives, 115(2), 235-242.
[18] Valko, M., Morris, H., & Cronin, M. T. D. (2005). Metals, toxicity and oxidative stress. Current Medicinal Chemistry, 12(10), 1161-1208.
[19] Ercal, N., Gurer, O. A., & Demirel, B. M. (2001). Free radicals and antioxidants in health and disease: mercury toxicity. Free Radical Biology and Medicine, 30(5), 463-474.
[20] Flora, S. J. S., Gupta, V. K., & Tiwari, A. (2012). Toxicity of lead: a review with recent updates. Interdisciplinary Toxicology, 5(2), 47-58.
[21] Beyersmann, D., & Hartwig, A. (2008). Carcinogenic metal compounds: recent insights into molecular mechanisms. Environmental Health Perspectives, 116(1), 3-9.
[22] IARC Working Group on the Evaluation of Carcinogenic Risks to Humans. (2012). Arsenic and arsenic compounds. IARC Monographs on the Evaluation of Carcinogenic Risks to Humans, 100C, 41-93.
[23] Naujokas, M. F., Anderson, B., Ahsan, H., Aposhian, H. V., Graziano, J. H., Thompson, C., & Suk, W. A. (2013). The broad scope of health effects from chronic arsenic exposure: Update on a worldwide public health problem. Environmental Health Perspectives, 121(3), 295-302.
[24] Wasserman, G. A., Liu, X., Parvez, F., Factor-Litvak, P., Ahsan, H., Levy, D., … & Graziano, J. H. (2004). Water arsenic exposure and children’s intellectual function in Araihazar, Bangladesh. Environmental Health Perspectives, 112(13), 1329-1333.
[25] Needleman, H. L. (2004). Lead poisoning. Annual Review of Medicine, 55, 209-222.
[26] ATSDR. (2007). Toxicological Profile for Lead. Agency for Toxic Substances and Disease Registry, Atlanta, GA.
[27] WHO. (2021). Lead poisoning. World Health Organization. https://www.who.int/news-room/fact-sheets/detail/lead-poisoning-and-health
[28] Nordberg, G. F., Jin, T., Wu, X., & Frech, W. (2007). Low-level exposure to cadmium and health: an updated perspective. Toxicology Letters, 175(1-3), 1-9.
[29] Lafuente, A. (2013). Cadmium exposure: General mechanisms of toxicity and influence on reproductive system. Veterinary Sciences, 1(3), 244-264.
[30] Clarkson, T. W., Magos, L., & Myers, G. J. (2003). The toxicology of mercury–current exposures and clinical manifestations. New England Journal of Medicine, 349(18), 1731-1737.
[31] Grandjean, P., Weihe, P., White, R. F., Needham, L. L., Burse, V. W., Lesca, M., & Murata, K. (1997). Cognitive deficit in 7-year-old children with prenatal exposure to methylmercury. Neurotoxicology and Teratology, 19(6), 417-428.
[32] Landrigan, P. J., Rauh, V. A., Galvez, M. P., Brenner, B. L., Stigliani, J. L., & Repetto, R. (2005). Neurodevelopmental disorders and environmental exposures: a critical review. Environmental Health Perspectives, 113(suppl 1), 1-19.
[33] Goyer, R. A. (1990). Transplacental transport of lead. Environmental Health Perspectives, 89, 101-105.
[34] Kippler, M., Tofail, F., Hamadani, J. D., Gardner, R. M., & Vahter, M. (2012). Early-life cadmium exposure and child development in 5 birth cohorts from low-and middle-income countries. Environmental Health Perspectives, 120(11), 1595-1600.
[35] Jarup, L. (2003). Hazards of heavy metal contamination. British Medical Bulletin, 68(1), 167-182.
[36] WHO. (1989). Evaluation of certain food additives and contaminants: Thirty-third report of the Joint FAO/WHO Expert Committee on Food Additives. World Health Organization.
[37] Codex Alimentarius Commission. (2023). Contaminants. http://www.fao.org/fao-who-codexalimentarius/standards/list-standards/en/
[38] European Commission. (2023). Contaminants in Food. https://food.ec.europa.eu/safety/chemical-safety/contaminants_en
[39] US Food and Drug Administration. (2023). Metals and Your Food. https://www.fda.gov/food/metals-and-your-food
[40] Health Canada. (2023). Chemical Contaminants in Foods. https://www.canada.ca/en/health-canada/services/food-nutrition/food-safety/chemical-contaminants.html
[41] Van Assche, K., & Swinnen, J. F. (2009). Quality standards and export performance in developing countries. Review of World Economics, 145(2), 249-272.
[42] EFSA Panel on Contaminants in the Food Chain (CONTAM). (2012). Scientific Opinion on the risks to public health related to the presence of nickel in food and drinking water. EFSA Journal, 10(12), 3002.
[43] Henson, S., & Loader, R. (2001). Barriers to agricultural exports from developing countries: the role of sanitary and phytosanitary requirements. World Development, 29(1), 85-102.
[44] WHO. (2000). Hazard assessment of metals. Assessment of dietary intake. World Health Organization.
[45] USEPA. (1996). Method 3050B: Acid digestion of sediments, sludges, and soils. United States Environmental Protection Agency.
[46] Mester, Z., & Sturgeon, R. E. (2003). Microwave digestion for ICP-MS. RSC Publishing.
[47] Thurman, E. M., & Mills, M. S. (1998). Solid-phase extraction: Principles and practice. John Wiley & Sons.
[48] Welz, B., & Sperling, M. (1999). Atomic absorption spectrometry. Wiley-VCH.
[49] Jarvis, K. E., Gray, A. L., & Houk, R. S. (1992). Handbook of inductively coupled plasma mass spectrometry. Blackie.
[50] Montaser, A. (Ed.). (1998). Inductively coupled plasma atomic emission spectrometry. Wiley-VCH.
[51] Jenkins, R. (1999). X-ray fluorescence spectrometry. John Wiley & Sons.
[52] EURACHEM. (1998). The fitness for purpose of analytical methods: A laboratory guide to method validation and related topics. LGC.
[53] Magnusson, B., & Örnemark, U. (Eds.). (2014). Eurachem Guide: The Fitness for Purpose of Analytical Methods – A Laboratory Guide to Method Validation and Related Topics (2nd ed.). https://www.eurachem.org/images/stories/Guides/pdf/MV_guide_2nd_ed.pdf
[54] UNEP. (2013). Global Mercury Assessment 2013. United Nations Environment Programme.
[55] Younger, P. L. (2002). Mining impacts on the fresh water environment: Technical and managerial guidelines for responsible mining. Crc Press.
[56] Metcalf & Eddy. (2014). Wastewater engineering: Treatment and resource recovery. McGraw-Hill Education.
[57] Ali, H., Khan, E., & Sajad, M. A. (2013). Phytoremediation of heavy metals—Concepts and applications. Chemosphere, 91(7), 869-881.
[58] Clemens, J. C., Palacios-Rojas, N., & McLaughlin, M. J. (2013). Genotypic differences in plant cadmium accumulation: A review of mechanisms and implications for human health. Environmental Science & Technology, 47(5), 1771-1787.
[59] Ayers, R. S., & Westcot, D. W. (1985). Water quality for agriculture. Food and Agriculture Organization of the United Nations.
[60] Pretty, J. (2008). Agricultural sustainability: concepts, principles and evidence. Philosophical Transactions of the Royal Society B: Biological Sciences, 363(1491), 447-465.
[61] Imathiu, S. M. (2009). Food contamination pathways and control measures. Pakistan Journal of Nutrition, 8(11), 1741-1763.
[62] Sharma, R. K., Agrawal, M., & Marshall, F. (2009). Heavy metal contamination in vegetables grown in suburban areas of Varanasi, India: Implications for human health. Environmental Toxicology and Chemistry, 28(3), 491-498.
[63] Farooq, M. A., Rehman, A., Ashraf, M., & Hussain, I. (2016). Processing effects on heavy metal content in food items: A review. Journal of Food Science and Technology, 53(1), 1-12.
[64] EFSA. (2012). Scientific Opinion on the benefits of fish/seafood consumption compared to the risks of methylmercury exposure. EFSA Journal, 10(7), 2798.
[65] Golan, E., Kuchler, F., & Mitchell, L. (2001). Economics of food labeling. Journal of Consumer Policy, 24(2), 117-184.
[66] WHO. (2002). Food safety: A practical guide for managers of health programmes. World Health Organization.
So, if soil and water are key contamination pathways, are hydroponics and aquaponics the silver bullets we’ve been missing, or just another set of challenges wrapped in a “sustainable” bow?