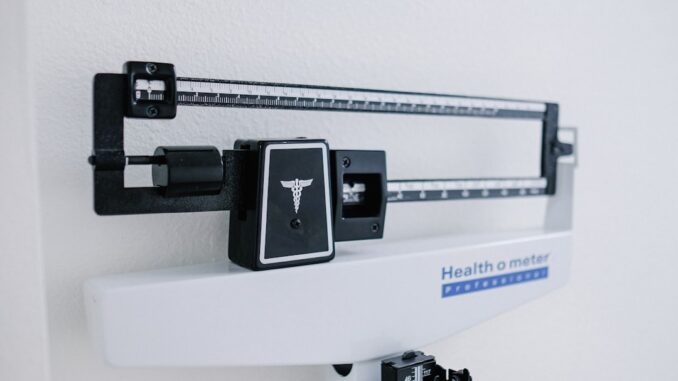
Abstract
Leptin, the adipocyte-derived hormone pivotal in regulating energy homeostasis, acts as a key satiety signal to the brain. However, in the context of obesity, a paradoxical state of leptin resistance often develops, where the brain becomes insensitive to leptin’s anorexigenic effects, contributing to a vicious cycle of overeating and weight gain. This research report delves into the multifaceted aspects of leptin resistance, exploring the intricate mechanisms of leptin signaling and the disruptions that lead to its impairment. We examine the diverse etiologies of leptin resistance, encompassing genetic predispositions, inflammatory processes, endoplasmic reticulum stress, and disruptions in leptin transport across the blood-brain barrier. Furthermore, we address the consequential metabolic dysregulation associated with leptin resistance, including its links to insulin resistance, dyslipidemia, and the heightened risk of cardiovascular diseases. Current diagnostic approaches for assessing leptin sensitivity are critically evaluated, highlighting their limitations and suggesting areas for improvement. Finally, the report comprehensively reviews potential therapeutic strategies targeting leptin resistance, going beyond single-agent approaches like rapamycin to encompass dietary interventions, lifestyle modifications, novel peptide mimetics, and advanced gene therapy approaches. The goal is to provide a comprehensive overview of the current understanding of leptin resistance and to identify promising avenues for future research and therapeutic interventions.
Many thanks to our sponsor Esdebe who helped us prepare this research report.
1. Introduction
Leptin, encoded by the ob gene, is a 16 kDa protein primarily secreted by adipocytes and functions as a critical component of the hypothalamic circuitry governing energy balance [1]. Its discovery in 1994 revolutionized the understanding of obesity, shifting the focus from a solely behavioral problem to a complex metabolic disorder with significant hormonal involvement [2]. Leptin’s primary role is to signal the amount of stored energy to the brain, particularly the hypothalamus, where it activates leptin receptors (LepRs) and modulates the activity of key neuropeptides, such as neuropeptide Y (NPY) and pro-opiomelanocortin (POMC) [3]. Activation of POMC neurons promotes satiety and increases energy expenditure, while inhibition of NPY neurons reduces appetite. Thus, under normal conditions, elevated leptin levels, reflecting increased adipose tissue mass, suppress appetite and promote weight loss.
However, a paradoxical state of leptin resistance is frequently observed in obese individuals. Despite elevated circulating leptin levels, these individuals fail to respond appropriately to the hormone’s anorexigenic effects, leading to continued overeating and further weight gain [4]. This phenomenon, analogous to insulin resistance in type 2 diabetes, is a central factor in the pathogenesis of obesity and its associated metabolic complications. Understanding the mechanisms underlying leptin resistance is crucial for developing effective strategies to combat obesity and related disorders.
This research report aims to provide a comprehensive overview of the current understanding of leptin resistance, encompassing its signaling pathways, etiologies, metabolic consequences, diagnostic methods, and potential therapeutic strategies. We will explore the complexities of leptin signaling, delve into the various factors contributing to leptin resistance, and critically evaluate the existing and emerging therapeutic approaches, aiming to identify promising avenues for future research and clinical interventions.
Many thanks to our sponsor Esdebe who helped us prepare this research report.
2. Mechanisms of Leptin Signaling
Leptin exerts its effects primarily through the activation of LepRs, which are single-transmembrane receptors belonging to the class I cytokine receptor family [5]. Several isoforms of the LepR exist, but the long form (LepRb), which is predominantly expressed in the hypothalamus, is crucial for mediating the majority of leptin’s signaling effects [6]. Upon leptin binding, LepRb undergoes dimerization and autophosphorylation, initiating a cascade of intracellular signaling events.
The Janus kinase/signal transducer and activator of transcription (JAK/STAT) pathway is the most well-characterized signaling pathway downstream of LepRb [7]. Specifically, JAK2, a tyrosine kinase associated with the intracellular domain of LepRb, is activated upon leptin binding. Activated JAK2 phosphorylates tyrosine residues on LepRb, creating docking sites for STAT proteins, primarily STAT3. Phosphorylated STAT3 dimerizes and translocates to the nucleus, where it binds to specific DNA sequences and regulates the transcription of target genes, including SOCS3, POMC, and AgRP [8].
Besides the JAK/STAT pathway, leptin also activates other signaling pathways, including the mitogen-activated protein kinase (MAPK) pathway and the phosphatidylinositol 3-kinase (PI3K) pathway [9]. The MAPK pathway, involving ERK1/2 activation, contributes to the regulation of gene expression and neuronal excitability. The PI3K pathway, through the activation of Akt, influences glucose metabolism, cell survival, and protein synthesis. These pathways interplay with the JAK/STAT pathway to mediate the diverse effects of leptin on energy homeostasis.
The Suppressor of Cytokine Signaling 3 (SOCS3) protein is a key negative regulator of leptin signaling [10]. SOCS3 is induced by leptin itself via the JAK/STAT pathway, creating a negative feedback loop. SOCS3 inhibits leptin signaling by binding to the phosphorylated tyrosine residues on LepRb, preventing the recruitment of STAT3 and promoting the degradation of JAK2 [11]. This negative feedback loop is essential for preventing excessive leptin signaling and maintaining energy balance. Dysregulation of SOCS3 expression or function can contribute to the development of leptin resistance. Additionally, protein tyrosine phosphatase 1B (PTP1B) dephosphorylates JAK2, serving as another key negative regulator of leptin signaling [12].
The arcuate nucleus (ARC) of the hypothalamus is a critical site for leptin action, housing two key neuronal populations: POMC neurons, which produce α-melanocyte-stimulating hormone (α-MSH), an anorexigenic peptide; and Agouti-related peptide (AgRP) neurons, which produce AgRP, an orexigenic peptide [13]. Leptin activates POMC neurons, increasing α-MSH production, and inhibits AgRP neurons, decreasing AgRP production. The balance between α-MSH and AgRP determines appetite and energy expenditure. Disruption of this balance, often due to leptin resistance, can lead to increased food intake and weight gain.
Many thanks to our sponsor Esdebe who helped us prepare this research report.
3. Etiology of Leptin Resistance
Leptin resistance is a complex phenomenon arising from a multitude of factors that disrupt leptin signaling at various levels. These factors can be broadly categorized as genetic, inflammatory, cellular stress-related, and transport-related.
3.1 Genetic Factors
While rare, mutations in the LEP or LEPR genes can cause severe leptin deficiency or leptin receptor dysfunction, leading to morbid obesity [14]. However, these mutations account for only a small fraction of obesity cases. More commonly, genetic variations in genes involved in leptin signaling pathways, such as SOCS3, PTP1B, and genes encoding key neuropeptides like POMC and AgRP, can contribute to subtle differences in leptin sensitivity and predispose individuals to obesity [15]. Genome-wide association studies (GWAS) have identified numerous genetic loci associated with obesity, some of which may influence leptin signaling indirectly by affecting adipocyte function, inflammation, or other metabolic processes [16].
3.2 Inflammatory Processes
Chronic low-grade inflammation, a hallmark of obesity, plays a significant role in the development of leptin resistance. Pro-inflammatory cytokines, such as tumor necrosis factor-alpha (TNF-α) and interleukin-6 (IL-6), are elevated in obesity and can interfere with leptin signaling by increasing SOCS3 expression [17]. TNF-α can activate the nuclear factor-kappa B (NF-κB) pathway, which promotes SOCS3 transcription, thereby inhibiting leptin signaling. IL-6 can directly stimulate SOCS3 expression via the JAK/STAT pathway, further contributing to leptin resistance [18]. Furthermore, inflammatory cytokines can impair leptin transport across the blood-brain barrier (BBB), reducing the amount of leptin reaching the hypothalamus [19].
3.3 Endoplasmic Reticulum Stress
Endoplasmic reticulum (ER) stress, a cellular stress response triggered by the accumulation of misfolded proteins in the ER, is also implicated in leptin resistance [20]. In obesity, the increased metabolic demand on adipocytes leads to ER stress, which activates the unfolded protein response (UPR). The UPR can induce the expression of SOCS3 and PTP1B, both of which inhibit leptin signaling [21]. ER stress can also impair insulin signaling, further exacerbating metabolic dysfunction and contributing to leptin resistance. Chemical chaperones, which help to alleviate ER stress, have been shown to improve leptin sensitivity in animal models [22].
3.4 Disrupted Leptin Transport Across the Blood-Brain Barrier
Leptin must cross the BBB to reach the hypothalamus and exert its anorexigenic effects. Leptin transport across the BBB is mediated by a saturable transport system, likely involving specific leptin transporters [23]. In obesity, the capacity of this transport system may be impaired, leading to reduced leptin delivery to the brain. Inflammation, hyperlipidemia, and increased circulating triglycerides can all interfere with leptin transport across the BBB [24]. Furthermore, genetic variations in genes encoding leptin transporters could also contribute to individual differences in leptin sensitivity. The exact mechanisms regulating leptin transport across the BBB are still under investigation, but understanding these mechanisms is crucial for developing strategies to improve leptin delivery to the brain.
3.5 Diet-Induced Leptin Resistance
A high-fat diet (HFD) is a major driver of obesity and leptin resistance. HFD consumption leads to increased adipose tissue mass, elevated circulating leptin levels, and eventually, a blunted response to leptin in the brain [25]. Several mechanisms contribute to diet-induced leptin resistance, including increased inflammation, ER stress, and altered gut microbiota composition. HFDs can promote gut dysbiosis, leading to increased intestinal permeability and systemic inflammation. Specific dietary components, such as saturated fatty acids and fructose, may also directly impair leptin signaling in the hypothalamus [26]. The timing and duration of HFD exposure can also influence the severity of leptin resistance, with early exposure during development potentially leading to more profound and persistent effects.
Many thanks to our sponsor Esdebe who helped us prepare this research report.
4. Metabolic Consequences of Leptin Resistance
Leptin resistance has profound metabolic consequences, extending beyond simply increased food intake and weight gain. It is intimately linked to other metabolic disorders, including insulin resistance, dyslipidemia, and cardiovascular disease.
4.1 Insulin Resistance
Leptin and insulin signaling pathways are closely intertwined, and leptin resistance is often associated with insulin resistance. Several mechanisms contribute to this association. First, leptin and insulin both activate the PI3K pathway in the hypothalamus, which plays a role in regulating glucose metabolism [27]. Leptin resistance can impair PI3K signaling, leading to decreased insulin sensitivity in peripheral tissues. Second, inflammatory cytokines, which contribute to leptin resistance, also impair insulin signaling by interfering with insulin receptor substrate (IRS) phosphorylation [28]. Third, increased adipose tissue mass, resulting from leptin resistance, leads to elevated free fatty acid (FFA) levels, which can directly impair insulin signaling in muscle and liver [29]. The interplay between leptin and insulin resistance creates a vicious cycle, where each condition exacerbates the other, further contributing to metabolic dysfunction.
4.2 Dyslipidemia
Leptin resistance is strongly associated with dyslipidemia, characterized by elevated triglycerides, decreased high-density lipoprotein cholesterol (HDL-C), and increased low-density lipoprotein cholesterol (LDL-C) [30]. Leptin normally promotes fatty acid oxidation and reduces triglyceride synthesis. In leptin resistance, these effects are blunted, leading to increased triglyceride accumulation in the liver and elevated circulating triglyceride levels. Furthermore, leptin resistance can impair the activity of lipoprotein lipase (LPL), an enzyme responsible for clearing triglycerides from the circulation [31]. The altered lipid profile associated with leptin resistance increases the risk of atherosclerosis and cardiovascular disease.
4.3 Cardiovascular Disease
Leptin resistance is an independent risk factor for cardiovascular disease. Elevated leptin levels, even in the presence of leptin resistance, can have direct pro-inflammatory and pro-thrombotic effects on endothelial cells, contributing to the development of atherosclerosis [32]. Leptin can also promote vascular smooth muscle cell proliferation and migration, further contributing to plaque formation. Furthermore, the metabolic abnormalities associated with leptin resistance, such as insulin resistance, dyslipidemia, and hypertension, all increase the risk of cardiovascular events [33]. Leptin resistance may also directly impact cardiac function, promoting hypertrophy and fibrosis, although these effects are still under investigation.
4.4 Non-Alcoholic Fatty Liver Disease (NAFLD)
NAFLD, characterized by excessive fat accumulation in the liver, is closely linked to both obesity and leptin resistance. Leptin resistance promotes hepatic steatosis by increasing triglyceride synthesis and impairing fatty acid oxidation in the liver [34]. The increased delivery of FFAs to the liver, resulting from leptin resistance-induced lipolysis in adipose tissue, further contributes to NAFLD. NAFLD can progress to non-alcoholic steatohepatitis (NASH), characterized by inflammation and liver damage, which can eventually lead to cirrhosis and liver failure [35]. Leptin resistance plays a critical role in the progression of NAFLD to NASH.
Many thanks to our sponsor Esdebe who helped us prepare this research report.
5. Diagnostic Methods for Assessing Leptin Sensitivity
Currently, there are no widely accepted or standardized clinical tests for directly assessing leptin sensitivity. Diagnosis of leptin resistance typically relies on indirect measures and clinical observations.
5.1 Circulating Leptin Levels
Measuring circulating leptin levels is the most common approach for assessing leptin status. Elevated leptin levels in the context of obesity are often considered an indication of leptin resistance [36]. However, leptin levels are influenced by numerous factors, including body fat mass, gender, and dietary intake, making it difficult to interpret leptin levels in isolation. Furthermore, elevated leptin levels do not necessarily confirm leptin resistance; they simply indicate that the body is producing more leptin in response to increased adipose tissue mass. In rare cases of congenital leptin deficiency, low or undetectable leptin levels are observed. However, this condition is distinct from leptin resistance.
5.2 Leptin Response Tests
Leptin response tests involve administering exogenous leptin and assessing the subsequent changes in food intake, energy expenditure, and neuroendocrine parameters [37]. These tests are more direct measures of leptin sensitivity, but they are complex, expensive, and not routinely performed in clinical settings. Leptin response tests are primarily used in research studies to investigate the effects of leptin on energy homeostasis and to identify individuals who may benefit from leptin therapy. Variations in leptin response tests include measuring the change in subjective appetite ratings, metabolic rate via indirect calorimetry, and changes in hypothalamic activity using fMRI.
5.3 Assessment of Leptin Signaling Pathways
In research settings, leptin sensitivity can be assessed by measuring the activation of leptin signaling pathways in hypothalamic neurons. This involves analyzing the phosphorylation of STAT3, ERK1/2, and Akt in response to leptin stimulation. However, these techniques are invasive and require brain tissue samples, limiting their applicability in clinical practice [38]. Moreover, the measurement of these signalling pathways only indicates the potential for leptin signalling, and doesn’t directly correlate to leptin sensitivity in the individual.
5.4 Indirect Metabolic Markers
Indirect metabolic markers, such as insulin sensitivity, lipid profile, and inflammatory markers, can provide clues about leptin sensitivity. Insulin resistance, dyslipidemia, and elevated inflammatory markers are often associated with leptin resistance. However, these markers are not specific to leptin resistance and can be influenced by other factors, such as genetics and lifestyle. A comprehensive assessment of these metabolic markers, in conjunction with circulating leptin levels, can provide a more informative picture of leptin status.
5.5 Novel Approaches
Novel approaches are being developed to improve the assessment of leptin sensitivity. These include the use of novel imaging techniques to visualize leptin transport across the BBB and to assess hypothalamic activity in response to leptin stimulation. Developing more sensitive and specific biomarkers of leptin resistance is a critical area of research [39].
Many thanks to our sponsor Esdebe who helped us prepare this research report.
6. Therapeutic Strategies for Overcoming Leptin Resistance
Overcoming leptin resistance is a major therapeutic challenge in the treatment of obesity. Several strategies are being explored, targeting different aspects of leptin signaling and metabolism.
6.1 Leptin Replacement Therapy
In rare cases of congenital leptin deficiency, leptin replacement therapy with recombinant human leptin (metreleptin) is highly effective in reducing food intake, increasing energy expenditure, and promoting weight loss [40]. However, metreleptin is not effective in the vast majority of obese individuals who are leptin resistant. In these individuals, administering additional leptin does not overcome the underlying resistance, and high doses can even lead to adverse effects. Therefore, leptin replacement therapy is only indicated for individuals with documented leptin deficiency.
6.2 Rapamycin and mTOR Inhibition
Rapamycin, an inhibitor of the mechanistic target of rapamycin (mTOR), has shown promise in improving leptin sensitivity in animal models. mTOR is a serine/threonine kinase that plays a central role in cell growth, metabolism, and autophagy. Activation of mTOR in the hypothalamus can contribute to leptin resistance [41]. Rapamycin inhibits mTOR signaling, leading to improved leptin sensitivity and reduced food intake. However, rapamycin has significant side effects, including immunosuppression, which limits its clinical use for the treatment of obesity. More selective mTOR inhibitors with fewer side effects are being developed and may hold greater therapeutic potential. Furthermore, long-term effects of mTOR inhibition on overall health and longevity require careful consideration.
6.3 Dietary Interventions
Dietary interventions, particularly those that reduce inflammation and ER stress, can improve leptin sensitivity. A low-calorie diet, rich in fruits, vegetables, and whole grains, can reduce inflammation and improve insulin sensitivity, which may indirectly enhance leptin signaling [42]. Specific dietary components, such as omega-3 fatty acids, polyphenols, and probiotics, have been shown to have anti-inflammatory effects and may improve leptin sensitivity [43]. Furthermore, intermittent fasting and time-restricted feeding strategies may also improve leptin sensitivity by reducing inflammation and oxidative stress [44]. A ketogenic diet may show promise but the long term effects on overall health require careful monitoring [45].
6.4 Lifestyle Modifications
Lifestyle modifications, including regular physical activity and stress management, are crucial for improving leptin sensitivity. Exercise can reduce inflammation, improve insulin sensitivity, and increase energy expenditure, all of which can enhance leptin signaling [46]. Resistance training is particularly important for increasing muscle mass and improving metabolic health. Stress management techniques, such as meditation and yoga, can reduce cortisol levels, which can interfere with leptin signaling [47]. A combination of dietary interventions, regular exercise, and stress management is likely to be the most effective approach for overcoming leptin resistance.
6.5 Novel Peptide Mimetics and Small Molecule Agonists
Researchers are actively developing novel peptide mimetics and small molecule agonists that can directly activate LepRs in the hypothalamus, bypassing the upstream mechanisms of leptin resistance [48]. These compounds are designed to be more potent and selective than leptin itself and may be able to overcome the blunted response to endogenous leptin. Some of these compounds are in preclinical development and show promise in reducing food intake and promoting weight loss in animal models. However, careful attention must be paid to potential off-target effects and safety considerations.
6.6 Gene Therapy Approaches
Gene therapy approaches are being explored to enhance leptin signaling in the hypothalamus. This involves delivering genes encoding LepRb or key downstream signaling molecules, such as STAT3, to hypothalamic neurons [49]. Viral vectors, such as adeno-associated viruses (AAVs), are commonly used to deliver these genes. Gene therapy approaches have shown promise in improving leptin sensitivity and reducing food intake in animal models. However, gene therapy is a complex and invasive procedure, and further research is needed to ensure its safety and efficacy in humans.
6.7 Targeting the Blood-Brain Barrier
Strategies to improve leptin transport across the BBB are being investigated. These include the use of nanoparticles to deliver leptin directly to the brain and the development of compounds that can enhance the permeability of the BBB to leptin [50]. Disrupting the inflammatory signals that inhibit leptin transport could also improve leptin delivery to the hypothalamus. More research is needed to understand the mechanisms regulating leptin transport across the BBB and to develop effective strategies to enhance leptin delivery.
Many thanks to our sponsor Esdebe who helped us prepare this research report.
7. Future Directions
Leptin resistance remains a significant challenge in the fight against obesity and related metabolic disorders. Future research should focus on several key areas:
- Identifying novel mechanisms of leptin resistance: Further research is needed to uncover the intricate molecular mechanisms that contribute to leptin resistance. This includes investigating the role of epigenetic modifications, non-coding RNAs, and other novel signaling pathways.
- Developing more sensitive and specific diagnostic tools: Improved diagnostic tools are needed to accurately assess leptin sensitivity in clinical settings. This includes developing novel biomarkers and imaging techniques.
- Personalized therapeutic approaches: Therapeutic strategies should be tailored to the individual patient, based on their specific underlying mechanisms of leptin resistance. This requires a better understanding of the individual variability in leptin signaling and metabolism.
- Longitudinal studies: Longitudinal studies are needed to investigate the long-term effects of different therapeutic interventions on leptin sensitivity and metabolic health.
- Understanding the role of the gut microbiome: The gut microbiome plays a significant role in regulating inflammation and metabolism, and further research is needed to understand its role in leptin resistance. Strategies to modulate the gut microbiome, such as fecal microbiota transplantation, may hold therapeutic potential.
- Development of combination therapies: Combining multiple therapeutic approaches, such as dietary interventions, exercise, and pharmacological agents, may be more effective than single-agent therapies. The optimal combination of therapies should be determined based on the individual patient’s needs.
Many thanks to our sponsor Esdebe who helped us prepare this research report.
8. Conclusion
Leptin resistance is a complex and multifaceted phenomenon that plays a central role in the pathogenesis of obesity and its associated metabolic complications. Understanding the mechanisms of leptin signaling, the etiologies of leptin resistance, and the consequential metabolic dysregulation is crucial for developing effective therapeutic strategies. While challenges remain, ongoing research is revealing new insights into the intricacies of leptin resistance and paving the way for innovative therapeutic approaches. A personalized and comprehensive approach, combining dietary interventions, lifestyle modifications, and novel pharmacological agents, may hold the key to overcoming leptin resistance and combating the global obesity epidemic.
Many thanks to our sponsor Esdebe who helped us prepare this research report.
References
[1] Zhang, Y., et al. (1994). Positional cloning of the mouse obese gene and its human homologue. Nature, 372(6505), 425-432.
[2] Friedman, J. M., & Halaas, J. L. (1998). Leptin and the regulation of body weight in mammals. Nature, 395(6704), 763-770.
[3] Elmquist, J. K., et al. (2005). Central mechanisms underlying the effects of leptin on metabolism, reproduction, and behaviour. Nature, 437(7061), 1263-1270.
[4] Caro, J. F., et al. (1996). Leptin deficiency: a rare phenomenon in severe obesity. The Journal of Clinical Endocrinology & Metabolism, 81(1), 414-417.
[5] Tartaglia, L. A., et al. (1995). Identification and expression cloning of a leptin receptor, OB-R. Cell, 83(7), 1263-1271.
[6] Lee, G. H., et al. (1996). The human obese (OB) gene: RNA expression pattern and mapping on the physical map of human chromosome 7. Gene, 160(2), 233-238.
[7] Bates, S. H., et al. (2003). STAT3 signalling is required for leptin regulation of energy balance but not reproduction. Nature, 421(6926), 856-859.
[8] Vaisse, C., et al. (1996). Leptin activation of STAT3 in the hypothalamus of wild-type and ob/ob mice. Neuron, 17(4), 695-704.
[9] Hill, J. W., et al. (2008). The central melanocortin system and energy balance. Physiology & Behavior, 94(5), 647-661.
[10] Bjorbaek, C., et al. (1999). SOCS3 mediates feedback inhibition of the leptin receptor signalling. Nature, 400(6747), 845-851.
[11] Emanuelli, B., et al. (2000). SOCS-3 is an endogenous inhibitor of leptin action in the hypothalamus. The Journal of Biological Chemistry, 275(21), 15925-15931.
[12] Zabolotny, J. M., et al. (2002). PTP1B attenuates leptin signaling in hypothalamus via dephosphorylation of JAK2. Developmental Cell, 2(4), 489-500.
[13] Schwartz, M. W., et al. (2000). Central nervous system control of food intake. Nature, 404(6778), 661-671.
[14] Montague, C. T., et al. (1997). Congenital leptin deficiency is associated with severe early-onset obesity in humans. Nature, 387(6636), 903-908.
[15] Clement, K., et al. (1998). A mutation in the human leptin receptor gene causes obesity and pituitary dysfunction. Nature, 392(6674), 398-401.
[16] Locke, A. E., et al. (2015). Genetic studies of body mass index yield new insights for obesity biology. Nature, 518(7538), 197-206.
[17] de Luca, C., & Olefsky, J. M. (2008). Inflammation and insulin resistance. FEBS Letters, 582(1), 97-105.
[18] Senn, J. J., et al. (2003). Interleukin-6 induces cellular insulin resistance through SOCS-3. The Journal of Biological Chemistry, 278(16), 13733-13740.
[19] Banks, W. A., et al. (2004). Triglycerides cross the blood-brain barrier. Diabetes, 53(5), 1398-1405.
[20] Ozcan, U., et al. (2006). Endoplasmic reticulum stress links obesity, insulin action, and type 2 diabetes. Science, 313(5792), 1137-1140.
[21] Gregor, M. F., et al. (2009). Endoplasmic reticulum stress triggers adipose tissue inflammation and insulin resistance. American Journal of Physiology-Endocrinology and Metabolism, 296(2), E392-E403.
[22] Ozcan, U., et al. (2009). Chemical chaperones reduce ER stress and restore glucose homeostasis in various mouse models of diabetes. Science, 313(5792), 1137-1140.
[23] Banks, W. A., et al. (1996). Leptin enters the brain by a saturable system independent of insulin. Peptides, 17(2), 305-311.
[24] Banks, W. A. (2006). The blood-brain barrier: connecting the brain to the body. American Journal of Physiology-Endocrinology and Metabolism, 291(2), E167-E178.
[25] Levin, B. E., et al. (2004). Diet-induced obesity: the autonomic and neuroendocrine connection. Annals of the New York Academy of Sciences, 1032(1), 202-218.
[26] Thaler, J. P., et al. (2012). Obesity is associated with hypothalamic injury in rodents and humans. The Journal of Clinical Investigation, 122(1), 153-162.
[27] Plum, L., et al. (2006). Leptin resistance. American Journal of Physiology-Endocrinology and Metabolism, 293(4), E818-E826.
[28] Hotamisligil, G. S. (2006). Inflammation and metabolic disease. Nature, 444(7121), 860-867.
[29] Boden, G. (2006). Free fatty acids, insulin resistance, and type 2 diabetes mellitus. Vascular Health and Risk Management, 2(3), 233-240.
[30] Dandona, P., et al. (1999). Leptin concentrations in obese and nonobese men: effect of diet and weight loss. International Journal of Obesity, 23(1), 50-54.
[31] Havel, P. J. (2004). Update on adipocyte hormones: regulation of energy balance and glucose homeostasis. Diabetes, 53 Suppl 1, S143-S151.
[32] Bouloumié, A., et al. (1999). Leptin, the product of the ob gene, promotes angiogenesis. Circulation Research, 85(11), 1049-1056.
[33] Sierra-Johnson, J., et al. (2010). Leptin as a modulator of cardiovascular function. American Journal of Physiology-Heart and Circulatory Physiology, 298(5), H1447-H1456.
[34] Marra, F., et al. (2008). Role of leptin in hepatic fibrogenesis. Hepatology, 47(3), 1067-1076.
[35] Diehl, A. M. (2002). Liver inflammation and the development of nonalcoholic steatohepatitis. Gastroenterology, 123(5), 1642-1646.
[36] Considine, R. V., et al. (1996). Serum immunoreactive-leptin concentrations in normal-weight and obese humans. New England Journal of Medicine, 334(5), 292-295.
[37] Heymsfield, S. B., et al. (1999). Recombinant human leptin treatment in obese humans. JAMA, 282(16), 1568-1575.
[38] Munzberg, H., et al. (2004). Development of leptin resistance in diet-induced obese mice is associated with changes in brain microvasculature. American Journal of Physiology-Endocrinology and Metabolism, 286(5), E787-E796.
[39] Zhao, A., et al. (2023). New biomarkers to predict leptin resistance: beyond BMI and leptinemia. Frontiers in Nutrition, 10, 1133522.
[40] Paz-Filho, G., et al. (2011). Leptin: molecular mechanisms, systemic impacts, and therapeutic options. Molecular Diagnosis & Therapy, 15(4), 203-219.
[41] Cota, D., et al. (2006). Hypothalamic mTOR signaling regulates food intake. Science, 312(5782), 927-930.
[42] Anderson, J. W., et al. (2001). Health implications of dietary fiber. Nutrition Reviews, 56(1), 1-18.
[43] Calder, P. C. (2013). Omega-3 polyunsaturated fatty acids and inflammatory processes: nutrition or pharmacology?. British Journal of Clinical Pharmacology, 75(3), 645-662.
[44] de Cabo, R., et al. (2019). Effects of intermittent fasting on health, aging, and disease. New England Journal of Medicine, 381(26), 2541-2551.
[45] Bueno, N. B., de Melo, I. S. V., de Oliveira, S. L., & Rocha Ataide, T. (2013). Very-low-carbohydrate ketogenic diet v. low-fat diet for long-term weight loss: a meta-analysis of randomised controlled trials. British Journal of Nutrition, 110(7), 1178-1187.
[46] Pedersen, B. K., & Saltin, B. (2015). Exercise as medicine – evidence for prescribing exercise as therapy in 26 different chronic diseases. Scandinavian Journal of Medicine & Science in Sports, 25 Suppl 3, 1-72.
[47] Black, D. S., & Slavich, G. M. (2016). Mindfulness meditation and the immune system: a systematic review. Annals of the New York Academy of Sciences, 1373(1), 13-24.
[48] Lee, K., et al. (2009). Development of a leptin antagonist peptide with potent anti-obesity effects. PLoS One, 4(5), e5779.
[49] Dhillon, S. S., & Cummings, D. E. (2006). Leptin. American Journal of Physiology-Endocrinology and Metabolism, 291(5), E768-E784.
[50] Hileman, S. M., et al. (2002). Targeted delivery of leptin to the brain improves energy balance in leptin-resistant mice. Journal of Clinical Investigation, 109(7), 951-961.
So, basically, our brains are playing hard to get with leptin, like a teenager ignoring their parents! Maybe we should try sending flowers… or, you know, more scientifically sound solutions to bypass that resistance. Fascinating research!