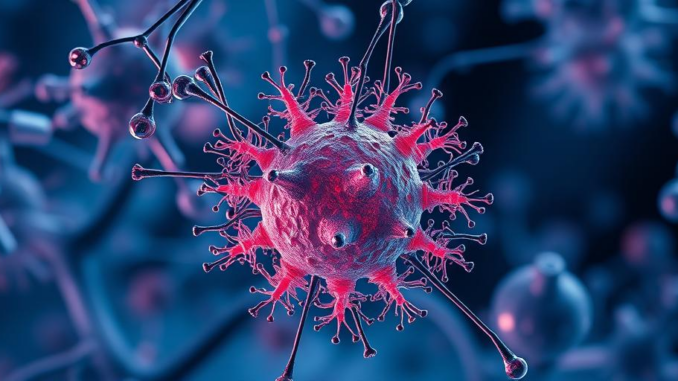
Abstract
Immunosuppression represents a cornerstone of modern medicine, enabling life-saving transplantation procedures and providing therapeutic avenues for autoimmune disorders. However, the inherent trade-off between preventing rejection or controlling autoimmunity and increasing susceptibility to infections and malignancies presents a persistent challenge. This research report delves into the multifaceted landscape of immunosuppression, examining its historical evolution from broad, non-specific approaches to the emerging era of targeted therapies and tolerance induction strategies. We explore the mechanisms of action, clinical applications, and limitations of various immunosuppressant drug classes, including calcineurin inhibitors, mTOR inhibitors, antimetabolites, and biologics. Furthermore, we critically analyze the advancements in personalized immunosuppression, leveraging genetic and immunological profiling to optimize treatment regimens and minimize adverse effects. Finally, we discuss the burgeoning field of tolerance induction, exploring both established and novel strategies aimed at achieving long-term graft acceptance or disease remission without the need for chronic immunosuppression. This review provides a comprehensive overview of the current state of immunosuppression and highlights promising future directions for safer and more effective immunomodulatory interventions.
Many thanks to our sponsor Esdebe who helped us prepare this research report.
1. Introduction
Immunosuppression, the deliberate suppression of the immune system, is a critical tool in modern medicine. Its necessity arises primarily in two distinct clinical scenarios: preventing organ rejection in transplantation and managing autoimmune diseases. In transplantation, the immune system’s natural tendency to recognize and attack foreign tissue necessitates the use of immunosuppressant drugs to prevent graft rejection. In autoimmune diseases, where the immune system mistakenly targets the body’s own tissues, immunosuppression aims to dampen the aberrant immune response and alleviate symptoms.
Historically, immunosuppression relied on broad, non-specific agents that targeted a wide range of immune cells and pathways. While these agents proved effective in preventing rejection and controlling autoimmune diseases, their lack of specificity resulted in significant side effects, including increased susceptibility to infections, malignancies, and metabolic complications. The development of more targeted immunosuppressants, such as monoclonal antibodies and fusion proteins, has represented a significant advance, allowing for more selective modulation of specific immune pathways. However, even these targeted therapies are not without their limitations, and the ideal of personalized immunosuppression remains a major goal in the field.
The ultimate goal of immunosuppression is to achieve long-term graft acceptance in transplantation or sustained disease remission in autoimmune diseases without the need for chronic immunosuppression. This concept, known as tolerance induction, involves re-educating the immune system to recognize the transplanted organ or self-antigens as harmless. While tolerance induction remains a major challenge, significant progress has been made in recent years, with several promising strategies showing potential in preclinical and clinical studies.
This research report will provide a comprehensive overview of the current state of immunosuppression, examining the different classes of immunosuppressant drugs, their mechanisms of action, and their clinical applications. We will also discuss the advancements in personalized immunosuppression and the emerging strategies for tolerance induction. Furthermore, we will critically analyze the limitations of current immunosuppressive approaches and highlight potential future directions for safer and more effective immunomodulatory interventions.
Many thanks to our sponsor Esdebe who helped us prepare this research report.
2. Classes of Immunosuppressant Drugs
A variety of immunosuppressant drugs are currently available, each with its own unique mechanism of action and spectrum of activity. These drugs can be broadly classified into the following categories:
2.1 Calcineurin Inhibitors (CNIs)
Calcineurin inhibitors, such as cyclosporine A (CsA) and tacrolimus (FK506), are among the most widely used immunosuppressants. They act by inhibiting calcineurin, a phosphatase enzyme that plays a critical role in T cell activation. Calcineurin is required for the dephosphorylation of the nuclear factor of activated T cells (NFAT), which then translocates to the nucleus and activates the transcription of genes encoding interleukin-2 (IL-2) and other cytokines. By inhibiting calcineurin, CNIs effectively block the production of IL-2, a key cytokine involved in T cell proliferation and differentiation.
CNIs are highly effective in preventing organ rejection and controlling autoimmune diseases. However, they are also associated with a number of significant side effects, including nephrotoxicity, hypertension, neurotoxicity, and increased risk of infections and malignancies. The nephrotoxicity associated with CNIs is a major concern, as it can lead to chronic kidney disease and graft loss in transplant recipients. The mechanisms underlying CNI-induced nephrotoxicity are complex and involve both vasoconstriction of the afferent arterioles and direct tubular toxicity.
2.2 mTOR Inhibitors
mTOR (mammalian target of rapamycin) inhibitors, such as sirolimus (rapamycin) and everolimus, act by inhibiting mTOR, a serine/threonine kinase that plays a central role in cell growth, proliferation, and survival. mTOR is a key regulator of T cell and B cell activation, proliferation, and differentiation. By inhibiting mTOR, these drugs suppress the immune response by blocking the downstream signaling pathways involved in these processes. Unlike CNIs, mTOR inhibitors primarily affect T cell proliferation rather than IL-2 production, offering a different, though overlapping, mechanism of immunosuppression.
mTOR inhibitors are effective in preventing organ rejection, particularly in combination with CNIs. They are also used in the treatment of certain cancers. A potential advantage of mTOR inhibitors is their lack of nephrotoxicity, making them an attractive alternative to CNIs in patients with kidney disease. However, mTOR inhibitors are associated with other side effects, including hyperlipidemia, thrombocytopenia, and impaired wound healing.
2.3 Antimetabolites
Antimetabolites, such as azathioprine and mycophenolate mofetil (MMF), interfere with DNA and RNA synthesis, thereby inhibiting cell proliferation. Azathioprine is a purine analog that is converted to 6-mercaptopurine, which inhibits purine synthesis. MMF is a prodrug that is converted to mycophenolic acid, which inhibits inosine monophosphate dehydrogenase (IMPDH), an enzyme essential for guanine nucleotide synthesis. Because lymphocytes are highly dependent on de novo purine and pyrimidine synthesis for proliferation, antimetabolites selectively suppress lymphocyte proliferation.
Antimetabolites are widely used in combination with other immunosuppressants to prevent organ rejection and treat autoimmune diseases. They are relatively inexpensive and well-tolerated, but they can cause bone marrow suppression, leading to leukopenia, anemia, and thrombocytopenia. MMF is generally preferred over azathioprine due to its greater efficacy and fewer side effects.
2.4 Corticosteroids
Corticosteroids, such as prednisone and methylprednisolone, are potent anti-inflammatory and immunosuppressive agents. They act by binding to the glucocorticoid receptor, which then translocates to the nucleus and regulates the transcription of a wide range of genes involved in immune and inflammatory responses. Corticosteroids suppress the production of cytokines, chemokines, and adhesion molecules, and they also promote apoptosis of lymphocytes.
Corticosteroids are used in virtually all immunosuppressive regimens, particularly in the acute phase following transplantation or during flares of autoimmune diseases. However, they are associated with a wide range of side effects, including weight gain, hyperglycemia, hypertension, osteoporosis, and mood changes. Efforts are often made to minimize the use of corticosteroids and to taper the dose as quickly as possible.
2.5 Biologics
Biologics are a class of immunosuppressants that target specific molecules involved in the immune response. These include monoclonal antibodies and fusion proteins that block the activity of cytokines, adhesion molecules, or T cell co-stimulatory molecules.
- Anti-cytokine antibodies: Examples include anti-TNF-α antibodies (e.g., infliximab, etanercept, adalimumab), anti-IL-6 antibodies (e.g., tocilizumab), and anti-IL-12/23 antibodies (e.g., ustekinumab). These antibodies neutralize the activity of specific cytokines that play a critical role in inflammation and immune activation.
- Anti-adhesion molecule antibodies: Examples include anti-integrin antibodies (e.g., natalizumab, vedolizumab). These antibodies block the binding of adhesion molecules to their ligands, preventing the migration of leukocytes into tissues.
- T cell co-stimulation blockers: Examples include CTLA-4-Ig (abatacept). This fusion protein binds to B7 molecules on antigen-presenting cells, blocking the interaction between B7 and CD28 on T cells, which is required for T cell activation.
- Anti-CD20 antibodies: Rituximab is an antibody that targets CD20, a protein found on the surface of B cells. By binding to CD20, rituximab depletes B cells from the circulation, which is effective in treating B cell-mediated autoimmune diseases.
Biologics are generally well-tolerated, but they can increase the risk of infections, particularly opportunistic infections. They can also be expensive and may be associated with infusion reactions.
Many thanks to our sponsor Esdebe who helped us prepare this research report.
3. Personalized Immunosuppression
The concept of personalized immunosuppression aims to tailor immunosuppressive regimens to the individual patient based on their genetic and immunological profile. This approach has the potential to optimize treatment efficacy and minimize adverse effects. Several factors can be considered in personalizing immunosuppression, including:
3.1 Genetic Factors
Genetic variations in genes encoding drug-metabolizing enzymes, drug transporters, and immune-related molecules can influence the response to immunosuppressant drugs. For example, genetic polymorphisms in the CYP3A5 gene, which encodes a cytochrome P450 enzyme involved in the metabolism of tacrolimus, can significantly affect tacrolimus drug levels. Patients with certain CYP3A5 genotypes require higher doses of tacrolimus to achieve therapeutic drug levels. Similarly, genetic variations in genes encoding IL-2, TNF-α, and other cytokines can influence the risk of rejection and the susceptibility to autoimmune diseases. Testing for these polymorphisms can help guide drug selection and dosing.
3.2 Immunological Monitoring
Monitoring the immune response can help to identify patients who are at high risk of rejection or infection. This can be achieved by measuring the levels of specific cytokines, chemokines, and immune cells in the blood. For example, measuring donor-specific antibodies (DSAs) in transplant recipients can help to identify patients who are at risk of antibody-mediated rejection. Monitoring T cell function, such as the ability to respond to donor antigens, can also provide valuable information about the risk of rejection. In autoimmune diseases, monitoring autoantibody levels and T cell subsets can help to assess disease activity and predict response to therapy. Flow cytometry is a key tool in monitoring immune cell populations and their activation status.
3.3 Biomarkers of Drug Response
Identifying biomarkers that predict response to specific immunosuppressant drugs can help to guide drug selection and avoid unnecessary exposure to ineffective therapies. For example, gene expression profiling of kidney biopsies can help to distinguish between different types of rejection and predict response to anti-rejection therapy. Similarly, measuring the levels of specific proteins in the blood can help to predict response to anti-TNF-α therapy in patients with rheumatoid arthritis. Such approaches help move away from a trial-and-error method to a more informed, predictive approach.
3.4 Risk Stratification
Integrating genetic, immunological, and clinical data to stratify patients into different risk groups can help to tailor immunosuppressive regimens to the individual patient’s risk profile. For example, patients at high risk of rejection may require more intensive immunosuppression, while patients at low risk of rejection may be able to tolerate reduced immunosuppression. Similarly, patients at high risk of infection may require prophylactic antiviral or antifungal therapy. Risk stratification models are increasingly used in clinical practice to guide immunosuppressive management.
Many thanks to our sponsor Esdebe who helped us prepare this research report.
4. Tolerance Induction
Tolerance induction is the holy grail of immunosuppression, aiming to achieve long-term graft acceptance or sustained disease remission without the need for chronic immunosuppression. Several strategies have been developed to induce tolerance, including:
4.1 Mixed Chimerism
Mixed chimerism involves the transplantation of hematopoietic stem cells (HSCs) from the donor into the recipient, resulting in the establishment of a mixed population of donor and recipient immune cells. This approach has been shown to induce tolerance in both preclinical and clinical studies. The mechanisms underlying tolerance induction by mixed chimerism are complex and involve both central and peripheral tolerance mechanisms.
4.2 Costimulatory Blockade
Blocking costimulatory signals, such as the interaction between CD28 and B7 molecules, can prevent T cell activation and promote tolerance. CTLA-4-Ig (abatacept) is a fusion protein that blocks the interaction between B7 and CD28, and it has been shown to induce tolerance in preclinical models. Clinical trials using costimulatory blockade to induce tolerance in transplant recipients are ongoing.
4.3 Regulatory T Cells (Tregs)
Regulatory T cells (Tregs) are a subset of T cells that suppress the activity of other immune cells, thereby maintaining immune homeostasis and preventing autoimmunity. Infusion of ex vivo expanded Tregs has been shown to induce tolerance in preclinical models. Clinical trials using Treg therapy to induce tolerance in transplant recipients and patients with autoimmune diseases are ongoing. Manipulating the Treg/Teff (effector T cell) balance is a key focus area.
4.4 Gene Therapy
Gene therapy approaches are being developed to introduce genes encoding immunosuppressive molecules into immune cells. For example, gene therapy has been used to express CTLA-4-Ig in T cells, which can then suppress the activity of other immune cells. Gene therapy also offers the potential to correct genetic defects that contribute to autoimmune diseases.
4.5 Emerging Strategies
Several other strategies for tolerance induction are currently under investigation, including:
- Induction of T cell exhaustion: Prolonged exposure to antigen can lead to T cell exhaustion, a state of T cell dysfunction characterized by reduced cytokine production and impaired effector function. Inducing T cell exhaustion can be a strategy for suppressing the immune response to transplanted organs or self-antigens.
- Targeting dendritic cells (DCs): Dendritic cells are antigen-presenting cells that play a critical role in initiating the immune response. Targeting DCs with immunosuppressive agents or tolerogenic signals can promote tolerance.
- Induction of apoptosis of autoreactive lymphocytes: Promoting apoptosis of autoreactive lymphocytes can eliminate the cells that are responsible for causing autoimmune diseases.
- Nanoparticle-based therapies: Nanoparticles can be used to deliver immunosuppressive drugs or tolerogenic antigens to specific immune cells, thereby enhancing their efficacy and minimizing side effects. This targeted drug delivery represents a sophisticated approach to immunosuppression.
Many thanks to our sponsor Esdebe who helped us prepare this research report.
5. Future Directions and Challenges
The field of immunosuppression is constantly evolving, with new drugs and strategies being developed to improve efficacy and minimize side effects. Some of the key future directions and challenges in this field include:
- Development of more selective immunosuppressants: The development of immunosuppressants that target specific immune pathways without affecting other parts of the immune system is a major goal. This would help to reduce the risk of infections and other side effects.
- Development of biomarkers for predicting drug response: Identifying biomarkers that predict response to specific immunosuppressant drugs would help to guide drug selection and avoid unnecessary exposure to ineffective therapies.
- Development of strategies for tolerance induction: Achieving tolerance induction remains a major challenge, but significant progress has been made in recent years. Continued research in this area is essential for developing therapies that can eliminate the need for chronic immunosuppression.
- Addressing the long-term complications of immunosuppression: Chronic immunosuppression is associated with a number of long-term complications, including infections, malignancies, and metabolic disorders. Developing strategies to prevent and manage these complications is a major challenge.
- Optimizing immunosuppression in special populations: Certain populations, such as children, the elderly, and patients with comorbidities, may require special consideration when it comes to immunosuppression. Optimizing immunosuppressive regimens in these populations is a major challenge.
Many thanks to our sponsor Esdebe who helped us prepare this research report.
6. Conclusion
Immunosuppression remains a critical component of transplantation and autoimmune disease management. While significant progress has been made in the development of more targeted and effective immunosuppressants, the inherent trade-off between preventing rejection or controlling autoimmunity and increasing susceptibility to infections and malignancies persists. Personalized immunosuppression, guided by genetic and immunological profiling, offers the potential to optimize treatment regimens and minimize adverse effects. The burgeoning field of tolerance induction holds promise for achieving long-term graft acceptance or sustained disease remission without the need for chronic immunosuppression. Overcoming the challenges associated with long-term immunosuppression and optimizing strategies for special populations remain crucial areas of focus. Continued research and innovation are essential for advancing the field of immunosuppression and improving the lives of patients who rely on these therapies.
Many thanks to our sponsor Esdebe who helped us prepare this research report.
References
- Abbas, A. K., Lichtman, A. H., & Pillai, S. (2018). Cellular and molecular immunology (9th ed.). Elsevier.
- Germain, R. N. (2011). The art of the possible: design principles for immune system intervention. Science, 334(6052), 45-49.
- Halloran, P. F. (2004). Immunosuppressive drugs for kidney transplantation. New England Journal of Medicine, 351(26), 2715-2729.
- Morris, R. E. (2003). Rapamycins: antifungal, antitumor, transplant, and antiretroviral drugs. Annals of the New York Academy of Sciences, 987, 28-36.
- Vincenti, F., Kirkland, T. B., & Hagopian, V. (2013). Strategies to optimize immunosuppression after kidney transplantation. American Journal of Transplantation, 13(S3), 33-46.
- Waldmann, H., & Cobbold, S. (2001). Regulating tolerance to transplants. Immunity, 14(4), 399-406.
- Webster, A. C., Chapman, J. R., Craig, J. C., Wong, G., & Oniscu, G. C. (2006). Mycophenolic acid versus azathioprine for immunosuppression in kidney transplant recipients. Cochrane Database of Systematic Reviews, *(2), CD002281.
- Tordesillas, L., Berglund, D., & D’Orsogna, L. (2022). Tolerance induction: emerging strategies and clinical applications. Frontiers in Immunology, 13, 974319.
- Lechler, R. I., Garden, O. A., & Turka, A. T. (2003). The promise of tolerance induction in transplantation. Immunity, 18(5), 627-638.
- Akdis, M., & Akdis, C. A. (2014). Mechanisms of immune tolerance. Annals of the New York Academy of Sciences, 1319(1), 36-64.
- Kawai, T., Sachs, D. H., & Cosimi, A. B. (2008). Mixed chimerism and transplantation tolerance. Current Opinion in Organ Transplantation, 13(4), 414-421.
- Bluestone, J. A., Buckner, J. H., Fitch, M., Gitelman, S. E., Gupta, S., Hellerstein, M. K., … & Tang, Q. (2015). Type 1 diabetes immunotherapy using polyclonal regulatory T cells. Science Translational Medicine, 7(315), 315ra189.
Be the first to comment