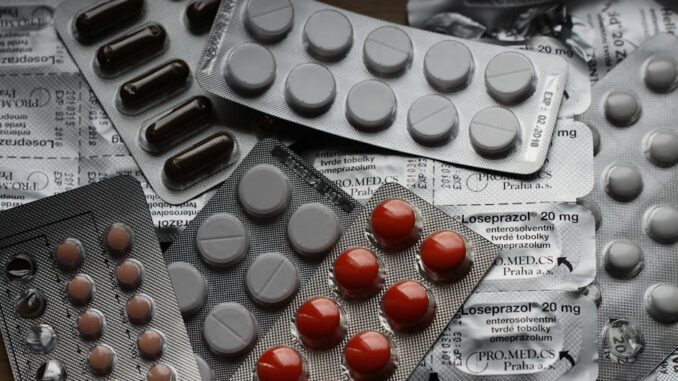
Abstract
Pharmacogenomics, the study of how genes affect a person’s response to drugs, has emerged as a transformative field in medicine, promising to tailor drug therapy to individual genetic profiles. This research report provides a comprehensive overview of pharmacogenomics, exploring its underlying principles, key applications across various therapeutic areas, technological advancements, ethical considerations, and future directions. We delve into the mechanisms by which genetic variations influence drug metabolism, transport, and target interactions, ultimately impacting drug efficacy and toxicity. Furthermore, we examine the clinical implementation of pharmacogenomic testing, highlighting successful examples and addressing the challenges associated with integrating genetic information into routine clinical practice. The report also discusses the evolving landscape of pharmacogenomic research, including the use of advanced technologies like next-generation sequencing and artificial intelligence to identify novel gene-drug associations and predict individual drug responses. Finally, we address the ethical and societal implications of pharmacogenomics, emphasizing the importance of ensuring equitable access to genetic testing, protecting patient privacy, and promoting informed consent.
Many thanks to our sponsor Esdebe who helped us prepare this research report.
1. Introduction
Pharmacogenomics represents a paradigm shift in drug therapy, moving away from a “one-size-fits-all” approach towards personalized medicine based on an individual’s genetic makeup. The field leverages the knowledge that genetic variations can significantly influence drug response, leading to differences in drug efficacy, toxicity, and metabolism among individuals. By understanding how genes affect drug disposition and action, clinicians can tailor drug selection and dosage to optimize therapeutic outcomes and minimize adverse effects. This approach holds particular promise for improving treatment outcomes in a variety of diseases, including cancer, cardiovascular disease, psychiatric disorders, and infectious diseases.
The concept of personalized medicine is not entirely new. Factors such as age, weight, sex, and kidney and liver function have long been considered when prescribing medications. However, pharmacogenomics adds a new dimension to personalized medicine by providing a deeper understanding of the genetic basis of drug response. This understanding allows for a more precise and individualized approach to drug therapy, potentially leading to more effective and safer treatments.
This report aims to provide a comprehensive overview of the field of pharmacogenomics, covering its fundamental principles, key applications, technological advancements, ethical considerations, and future directions. We will explore the mechanisms by which genetic variations influence drug response, examine the clinical implementation of pharmacogenomic testing, and discuss the evolving landscape of pharmacogenomic research. Ultimately, this report seeks to highlight the potential of pharmacogenomics to revolutionize drug therapy and improve patient outcomes.
Many thanks to our sponsor Esdebe who helped us prepare this research report.
2. Genetic Basis of Drug Response
The foundation of pharmacogenomics lies in understanding the genetic factors that influence drug response. These factors can be broadly categorized into genes involved in drug metabolism, drug transport, and drug targets. Variations in these genes can lead to significant differences in how individuals process and respond to medications.
2.1 Drug Metabolism
Drug metabolism, also known as biotransformation, is the process by which the body chemically modifies drugs to facilitate their elimination. This process is primarily carried out by enzymes in the liver, particularly cytochrome P450 (CYP) enzymes. CYP enzymes are a family of heme-containing monooxygenases that catalyze the oxidation of a wide range of drugs. Genetic variations in CYP genes can significantly affect enzyme activity, leading to differences in drug metabolism rates. For example, individuals with certain CYP2C19 variants may be poor metabolizers of clopidogrel, a platelet aggregation inhibitor used to prevent blood clots. This can result in reduced drug efficacy and an increased risk of cardiovascular events. Conversely, individuals with other CYP2C19 variants may be ultra-rapid metabolizers, leading to decreased drug exposure and a potential need for higher dosages.
Other important drug-metabolizing enzymes include uridine diphosphate glucuronosyltransferases (UGTs), sulfotransferases (SULTs), and N-acetyltransferases (NATs). Genetic variations in these enzymes can also influence drug metabolism and response.
2.2 Drug Transport
Drug transporters play a crucial role in drug absorption, distribution, and excretion. These proteins facilitate the movement of drugs across cell membranes, influencing their concentration at the site of action and their elimination from the body. ATP-binding cassette (ABC) transporters and solute carrier (SLC) transporters are two major families of drug transporters.
Genetic variations in drug transporter genes can affect transporter expression or function, leading to altered drug concentrations in the body. For example, variations in the ABCB1 gene, which encodes P-glycoprotein, a major efflux transporter, can affect the transport of various drugs, including chemotherapeutic agents and immunosuppressants. Individuals with certain ABCB1 variants may have reduced P-glycoprotein activity, leading to increased drug concentrations in tissues and potentially increased toxicity. Conversely, individuals with other ABCB1 variants may have increased P-glycoprotein activity, leading to decreased drug concentrations in tissues and potentially reduced efficacy.
2.3 Drug Targets
Drug targets are the molecules or structures that drugs interact with to produce their therapeutic effects. These targets can be enzymes, receptors, ion channels, or other proteins involved in disease pathways. Genetic variations in drug target genes can affect the structure or function of the target, leading to altered drug binding affinity or downstream signaling. For example, variations in the VKORC1 gene, which encodes vitamin K epoxide reductase complex subunit 1, the target of warfarin, can affect warfarin sensitivity. Individuals with certain VKORC1 variants may require lower doses of warfarin to achieve therapeutic anticoagulation, while individuals with other VKORC1 variants may require higher doses.
Variations in genes encoding drug targets can also influence the development of drug resistance. For example, mutations in the EGFR gene, which encodes epidermal growth factor receptor, can lead to resistance to EGFR inhibitors in patients with non-small cell lung cancer.
Many thanks to our sponsor Esdebe who helped us prepare this research report.
3. Technological Advancements in Pharmacogenomics
The advancement of pharmacogenomics is deeply intertwined with technological progress, particularly in the fields of genomics, bioinformatics, and data analysis. These technologies have enabled the identification of novel gene-drug associations, the development of pharmacogenomic tests, and the integration of genetic information into clinical decision-making.
3.1 Genotyping and Sequencing Technologies
Genotyping technologies, such as microarrays and polymerase chain reaction (PCR)-based assays, are used to detect known genetic variants in specific genes. These technologies are relatively inexpensive and high-throughput, making them suitable for routine pharmacogenomic testing. Next-generation sequencing (NGS) technologies, such as whole-genome sequencing (WGS) and whole-exome sequencing (WES), allow for the comprehensive sequencing of an individual’s entire genome or exome, respectively. NGS technologies can identify both known and novel genetic variants, providing a more complete picture of an individual’s genetic makeup. While NGS technologies are more expensive and time-consuming than genotyping technologies, they are becoming increasingly accessible and are being used to discover novel gene-drug associations and personalize drug therapy for complex diseases.
3.2 Bioinformatics and Data Analysis
Bioinformatics and data analysis play a crucial role in interpreting pharmacogenomic data and translating it into clinically actionable information. Bioinformatics tools are used to analyze large datasets of genomic and clinical data, identify gene-drug associations, and predict individual drug responses. Machine learning algorithms are increasingly being used to develop predictive models that can integrate genetic information with other clinical and demographic factors to personalize drug therapy. These models can help clinicians select the most appropriate drug and dosage for each patient, minimizing the risk of adverse effects and maximizing therapeutic efficacy.
3.3 Electronic Health Records and Clinical Decision Support Systems
The integration of pharmacogenomic information into electronic health records (EHRs) and clinical decision support systems (CDSSs) is essential for the widespread implementation of pharmacogenomics in clinical practice. EHRs can store and manage an individual’s genetic information, making it readily accessible to clinicians. CDSSs can use this information to provide alerts and recommendations to clinicians regarding drug selection and dosage based on the patient’s genetic profile. This can help clinicians make more informed decisions and improve patient outcomes. However, the integration of pharmacogenomic information into EHRs and CDSSs poses several challenges, including the need for standardized data formats, interoperability between different systems, and user-friendly interfaces.
Many thanks to our sponsor Esdebe who helped us prepare this research report.
4. Clinical Applications of Pharmacogenomics
Pharmacogenomics has a wide range of clinical applications across various therapeutic areas. Several examples highlight the potential of pharmacogenomics to improve treatment outcomes and reduce adverse effects.
4.1 Oncology
Pharmacogenomics has made significant strides in oncology, particularly in the field of targeted therapy. Genetic testing can identify patients who are more likely to respond to specific targeted therapies, such as EGFR inhibitors in non-small cell lung cancer and HER2 inhibitors in breast cancer. For example, patients with EGFR mutations in their tumors are more likely to benefit from EGFR inhibitors, while patients with HER2 amplification in their tumors are more likely to benefit from HER2 inhibitors. Pharmacogenomics can also help predict the risk of adverse effects from chemotherapy. For example, patients with certain UGT1A1 variants are at increased risk of developing severe neutropenia from irinotecan, a chemotherapy drug used to treat colorectal cancer. By identifying these patients, clinicians can adjust the dosage of irinotecan or consider alternative treatments to minimize the risk of adverse effects.
4.2 Cardiology
Pharmacogenomics is also playing an increasingly important role in cardiology, particularly in the management of cardiovascular diseases such as hypertension, heart failure, and thromboembolic disorders. Genetic testing can help predict an individual’s response to various cardiovascular drugs, such as clopidogrel, warfarin, and statins. As mentioned earlier, CYP2C19 variants can affect clopidogrel metabolism and response. VKORC1 and CYP2C9 variants can affect warfarin sensitivity. SLCO1B1 variants can affect statin transport and the risk of statin-induced myopathy. By identifying these genetic variations, clinicians can personalize drug therapy to optimize efficacy and minimize the risk of adverse effects.
4.3 Psychiatry
Pharmacogenomics holds great promise for improving the treatment of psychiatric disorders, such as depression, anxiety, and schizophrenia. Psychiatric medications are often associated with significant inter-individual variability in response and adverse effects. Genetic testing can help predict an individual’s response to various psychiatric medications, such as antidepressants, antipsychotics, and mood stabilizers. For example, CYP2D6 and CYP2C19 variants can affect the metabolism of many antidepressants. By identifying these genetic variations, clinicians can personalize drug therapy to improve efficacy and reduce the risk of adverse effects. However, the clinical utility of pharmacogenomic testing in psychiatry is still being evaluated, and more research is needed to establish its role in routine clinical practice.
4.4 Pain Management
Pharmacogenomics can also be applied to pain management to personalize the selection and dosage of analgesics. Genetic variations can influence the metabolism and response to opioids, such as codeine, tramadol, and oxycodone. For example, CYP2D6 variants can affect the conversion of codeine to morphine, its active metabolite. Individuals with certain CYP2D6 variants may be poor metabolizers of codeine, resulting in reduced analgesia. Conversely, individuals with other CYP2D6 variants may be ultra-rapid metabolizers, leading to increased morphine levels and a higher risk of adverse effects. By identifying these genetic variations, clinicians can personalize opioid therapy to optimize pain relief and minimize the risk of adverse effects. However, the use of pharmacogenomics in pain management should be carefully considered, as it can be complex and may not always accurately predict individual responses to opioids.
Many thanks to our sponsor Esdebe who helped us prepare this research report.
5. Ethical Considerations in Pharmacogenomics
The ethical implications of pharmacogenomics are significant and multifaceted, raising concerns about privacy, confidentiality, informed consent, and equitable access to genetic testing.
5.1 Privacy and Confidentiality
Genetic information is highly sensitive and personal, raising concerns about privacy and confidentiality. It is essential to protect individuals’ genetic information from unauthorized access and disclosure. Strict security measures should be implemented to safeguard genetic data, and individuals should have control over how their genetic information is used and shared. Laws and regulations should be in place to prevent genetic discrimination in employment, insurance, and other areas.
5.2 Informed Consent
Informed consent is a fundamental ethical principle that requires individuals to be fully informed about the risks and benefits of genetic testing before making a decision. Individuals should be provided with clear and understandable information about the purpose of the test, the potential results, the implications of the results for their health and treatment, and the options available to them. They should also be informed about the potential risks to privacy and confidentiality. Individuals should have the right to refuse genetic testing and to withdraw their consent at any time.
5.3 Equitable Access
Ensuring equitable access to pharmacogenomic testing is crucial to prevent disparities in health care. Genetic testing should be accessible to all individuals, regardless of their socioeconomic status, race, ethnicity, or geographic location. Efforts should be made to reduce the cost of genetic testing and to provide education and outreach to underserved communities. Reimbursement policies should be developed to ensure that genetic testing is covered by insurance.
5.4 Genetic Counseling
Genetic counseling plays a vital role in helping individuals understand the implications of genetic testing and make informed decisions about their health care. Genetic counselors can provide education, support, and guidance to individuals and their families. They can help individuals understand the results of genetic tests, assess their risk of developing certain diseases, and discuss the options available to them. Genetic counseling should be integrated into the clinical implementation of pharmacogenomics to ensure that individuals receive the support and information they need.
Many thanks to our sponsor Esdebe who helped us prepare this research report.
6. Challenges and Future Directions
Despite its immense potential, pharmacogenomics faces several challenges that need to be addressed to facilitate its widespread implementation in clinical practice.
6.1 Lack of Evidence and Clinical Guidelines
One of the major challenges is the lack of robust evidence to support the clinical utility of many pharmacogenomic tests. More clinical trials are needed to demonstrate the benefits of pharmacogenomic testing in improving patient outcomes and reducing adverse effects. Clinical guidelines should be developed to provide clinicians with clear and evidence-based recommendations on how to use pharmacogenomic information in clinical decision-making. Organizations such as the Clinical Pharmacogenetics Implementation Consortium (CPIC) are actively working to develop and disseminate evidence-based guidelines for pharmacogenomic testing.
6.2 Education and Training
Another challenge is the lack of education and training among healthcare professionals regarding pharmacogenomics. Many clinicians are not familiar with the principles of pharmacogenomics or how to interpret and apply pharmacogenomic information in clinical practice. Education and training programs should be developed to equip clinicians with the knowledge and skills they need to effectively use pharmacogenomics to personalize drug therapy. These programs should be integrated into medical school curricula, residency training programs, and continuing medical education activities.
6.3 Cost and Reimbursement
The cost of genetic testing can be a barrier to its widespread implementation. Efforts should be made to reduce the cost of genetic testing and to develop reimbursement policies that ensure that genetic testing is covered by insurance. Economic analyses should be conducted to demonstrate the cost-effectiveness of pharmacogenomic testing.
6.4 Integration into Clinical Workflow
The integration of pharmacogenomic information into the clinical workflow can be challenging. It is essential to develop user-friendly systems that make it easy for clinicians to access and interpret pharmacogenomic information. Pharmacogenomic information should be seamlessly integrated into electronic health records and clinical decision support systems.
6.5 Future Directions
The future of pharmacogenomics is promising. Advances in genomics, bioinformatics, and data analysis are paving the way for the discovery of novel gene-drug associations and the development of more personalized drug therapies. The use of artificial intelligence and machine learning is likely to accelerate the discovery of novel gene-drug associations and the development of predictive models that can personalize drug therapy. The integration of pharmacogenomics with other omics technologies, such as proteomics and metabolomics, will provide a more comprehensive understanding of individual drug responses. The development of point-of-care pharmacogenomic testing devices will make it easier to perform genetic testing at the point of care, enabling rapid and personalized drug therapy.
Many thanks to our sponsor Esdebe who helped us prepare this research report.
7. Conclusion
Pharmacogenomics has emerged as a powerful tool for personalizing drug therapy and improving patient outcomes. By understanding the genetic factors that influence drug response, clinicians can tailor drug selection and dosage to optimize therapeutic efficacy and minimize adverse effects. While challenges remain, ongoing research, technological advancements, and increased education and training are paving the way for the widespread implementation of pharmacogenomics in clinical practice. As the field continues to evolve, it holds the potential to revolutionize drug therapy and improve the lives of countless individuals.
Many thanks to our sponsor Esdebe who helped us prepare this research report.
References
- Relling, M. V., & Evans, W. E. (2015). Pharmacogenomics in the clinic. Nature, 526(7573), 343-350.
- Ingelman-Sundberg, M. (2005). Genetic polymorphism of cytochrome P450 2D6 (CYP2D6): clinical consequences, evolutionary aspects and functional assessment. Trends in pharmacological sciences, 26(10), 487-494.
- Evans, W. E., & McLeod, H. L. (2003). Pharmacogenomics—drug disposition, drug targets, and disease. New England Journal of Medicine, 348(6), 538-549.
- Ramamoorthy, A., Flockhart, D. A., & Hosseini-Maaf, B. (2015). Clinical pharmacogenomics: past, present, and future. Molecular diagnosis & therapy, 19(6), 377-389.
- Swen, J. J., Nijenhuis, M., de Boer, A., Verster, A. D., Mulder, H., Rongen, G. A., … & Guchelaar, H. J. (2011). Pharmacogenetics: towards personalized medicine. The Netherlands Journal of Medicine, 69(9), 362.
- The Clinical Pharmacogenetics Implementation Consortium (CPIC). Retrieved from https://cpicpgx.org/
- Pirmohamed, M. (2011). Pharmacogenomics in the clinic: challenges and opportunities. British journal of clinical pharmacology, 72(6), 835-840.
- Manolopoulos, V. G., Ragia, G., Piperi, C., & Bagos, P. G. (2010). Clinical pharmacogenomics: what is the current status?. Current pharmaceutical biotechnology, 11(2), 154-162.
- Ginsburg, G. S., & Willard, H. F. (2009). Genomic and personalized medicine: foundations and applications. Translation research, 154(6), 247-259.
- Lee, C. R., Goldstein, J. A., & Pieper, J. A. (2003). Clinical relevance of genetic polymorphisms affecting drug metabolism. Trends in molecular medicine, 9(8), 340-349.
- Owen, R. P., & Ritchie, M. D. (2018). Integrating genetic and clinical data to improve pharmacogenomics. Clinical pharmacology & therapeutics, 103(5), 771-773.
Be the first to comment