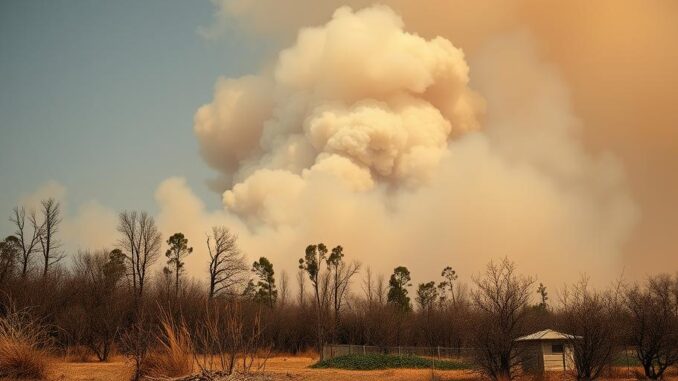
Abstract
Particulate matter with an aerodynamic diameter of 2.5 micrometers or less (PM2.5) poses a significant threat to global public health. This review provides a comprehensive overview of PM2.5, encompassing its diverse sources, including anthropogenic emissions (combustion processes, industrial activities, transportation) and natural events like wildfires and dust storms. We delve into the chemical composition of PM2.5 from different sources, highlighting the unique characteristics of wildfire smoke. The report comprehensively assesses the health impacts of PM2.5 exposure, ranging from acute respiratory and cardiovascular effects to chronic conditions like cancer and neurodegenerative diseases, emphasizing the heightened risks associated with wildfire-derived PM2.5. Furthermore, we examine the current state-of-the-art in PM2.5 monitoring techniques, encompassing both ground-based and satellite-based methods. Finally, the review explores various mitigation strategies, including emission control technologies, air quality management policies, and personal protective measures, with a particular focus on developing and implementing strategies to reduce exposure to wildfire smoke. The review identifies key knowledge gaps and suggests avenues for future research to better understand and address the challenges posed by PM2.5 pollution.
Many thanks to our sponsor Esdebe who helped us prepare this research report.
1. Introduction
Air pollution, particularly in the form of particulate matter, has emerged as a leading environmental threat to human health worldwide. Among the various sizes of particulate matter, PM2.5, defined as particles with an aerodynamic diameter of 2.5 micrometers or less, is of particular concern due to its ability to penetrate deep into the respiratory system and even enter the bloodstream. This deep penetration allows PM2.5 to directly interact with sensitive tissues, triggering a cascade of adverse health effects.
The sources of PM2.5 are diverse and can be broadly classified into anthropogenic and natural categories. Anthropogenic sources include combustion processes in vehicles, power plants, industrial facilities, and residential heating, as well as non-combustion sources like construction and agriculture. Natural sources encompass dust storms, volcanic eruptions, and wildfires. While anthropogenic sources often dominate PM2.5 pollution in urban and industrial areas, wildfires have become an increasingly significant contributor, particularly in regions experiencing climate change and increased drought conditions. The chemical composition of PM2.5 varies depending on the source and atmospheric conditions. PM2.5 can contain a complex mixture of organic and inorganic compounds, including elemental carbon, organic carbon, sulfate, nitrate, ammonium, metals, and polycyclic aromatic hydrocarbons (PAHs).
Wildfire smoke is a particularly complex and hazardous form of PM2.5. It is composed of a diverse range of combustion products, including fine particulate matter, volatile organic compounds (VOCs), and toxic gases. The composition of wildfire smoke PM2.5 varies depending on the type of vegetation burned, the combustion temperature, and atmospheric conditions. Wildfire PM2.5 often contains a higher proportion of organic carbon and elemental carbon compared to PM2.5 from other sources. This unique chemical composition can lead to different and potentially more severe health effects.
This review aims to provide a comprehensive overview of PM2.5, encompassing its sources, health impacts, monitoring techniques, and mitigation strategies. We will delve into the chemical composition differences between PM2.5 from wildfires and other sources of pollution, the specific health impacts of wildfire-derived PM2.5 on different organ systems, and the long-term consequences of chronic exposure, including potential links to cancer or neurodegenerative diseases. Additionally, we will cover the development of more effective air filtration technologies specifically designed to capture wildfire PM2.5. This review is intended for experts in the field and will provide a valuable resource for researchers, policymakers, and public health professionals working to address the challenges posed by PM2.5 pollution.
Many thanks to our sponsor Esdebe who helped us prepare this research report.
2. Sources and Chemical Composition of PM2.5
2.1. Anthropogenic Sources
Anthropogenic activities are a major source of PM2.5 pollution, particularly in urban and industrial areas. Combustion processes are a primary contributor, releasing PM2.5 from the burning of fossil fuels in vehicles, power plants, and industrial facilities. Road transport remains a critical source, with diesel engines being particularly problematic due to their higher PM emissions. Industrial activities, such as manufacturing, construction, and mining, also generate significant amounts of PM2.5. Residential heating, especially in colder climates where wood or coal is burned, can be a substantial source of PM2.5 during winter months. The chemical composition of PM2.5 from anthropogenic sources typically includes elemental carbon, organic carbon, sulfate, nitrate, ammonium, metals, and PAHs. The specific composition varies depending on the type of combustion process and the fuels used.
2.2. Natural Sources
Natural sources of PM2.5 include dust storms, volcanic eruptions, and wildfires. Dust storms, particularly in arid and semi-arid regions, can generate large quantities of PM2.5, consisting primarily of mineral dust particles. Volcanic eruptions release PM2.5 containing ash, gases, and aerosols. Wildfires, as discussed previously, are an increasingly important source of PM2.5, particularly in regions experiencing climate change and increased drought conditions. The contribution of natural sources to PM2.5 pollution can vary significantly depending on the region and time of year.
2.3. Chemical Composition Differences Between PM2.5 from Wildfires and Other Sources
While PM2.5 from all sources poses a health risk, the chemical composition of wildfire-derived PM2.5 often differs significantly from that of PM2.5 from other sources, potentially leading to different and more severe health effects. Wildfire smoke PM2.5 typically contains a higher proportion of organic carbon and elemental carbon compared to PM2.5 from anthropogenic sources. This is due to the incomplete combustion of biomass during wildfires. The organic carbon fraction of wildfire PM2.5 can contain a wide range of organic compounds, including VOCs, oxygenated organic compounds, and PAHs. Elemental carbon, also known as black carbon, is a strong absorber of sunlight and contributes to climate warming. Furthermore, wildfire smoke PM2.5 can contain metals and other trace elements, depending on the type of vegetation burned and the soil composition. The presence of elevated levels of organic carbon, elemental carbon, and specific organic compounds in wildfire smoke PM2.5 may contribute to its enhanced toxicity compared to PM2.5 from other sources. Some studies suggest that wildfire PM2.5 can cause increased inflammation and oxidative stress in the lungs compared to PM2.5 from other sources.
The chemical composition of wildfire smoke is extremely variable and dependent on a number of factors, including fuel type, combustion efficiency and weather conditions. The variability of wildfire smoke PM2.5 composition complicates the toxicological studies aimed at disentangling the specific effects of it on human health. Research also suggests that the aging of wildfire smoke in the atmosphere can alter its chemical composition and toxicity. During atmospheric transport, wildfire smoke PM2.5 can undergo chemical reactions with other pollutants, such as ozone and nitrogen oxides, leading to the formation of secondary organic aerosols (SOA) and other products. These transformations can alter the size distribution, chemical composition, and toxicity of wildfire smoke PM2.5. A pressing challenge for future research is the need to develop more accurate methods for characterizing the chemical composition and toxicity of wildfire smoke PM2.5 under different environmental conditions.
Many thanks to our sponsor Esdebe who helped us prepare this research report.
3. Health Impacts of PM2.5 Exposure
3.1. Acute Health Effects
Exposure to PM2.5 can trigger a range of acute health effects, particularly in susceptible populations such as children, the elderly, and individuals with pre-existing respiratory or cardiovascular diseases. Short-term exposure to PM2.5 can lead to respiratory symptoms such as coughing, wheezing, shortness of breath, and increased asthma exacerbations. PM2.5 can also irritate the eyes, nose, and throat. In individuals with cardiovascular disease, short-term PM2.5 exposure can increase the risk of heart attacks, strokes, and arrhythmias. Studies have shown that emergency room visits and hospitalizations for respiratory and cardiovascular conditions increase during periods of high PM2.5 pollution. The mechanisms by which PM2.5 causes these acute health effects include inflammation, oxidative stress, and direct damage to lung tissue and blood vessels.
3.2. Chronic Health Effects
Chronic exposure to PM2.5 can lead to more serious long-term health problems, including respiratory diseases, cardiovascular diseases, cancer, and neurodegenerative diseases. Long-term PM2.5 exposure has been linked to increased risk of chronic bronchitis, emphysema, and asthma. Studies have also shown that chronic PM2.5 exposure can accelerate the progression of cardiovascular disease, increasing the risk of heart attacks, strokes, and heart failure. There is growing evidence that chronic PM2.5 exposure can increase the risk of lung cancer and other cancers. Furthermore, recent research suggests that chronic PM2.5 exposure may contribute to the development of neurodegenerative diseases such as Alzheimer’s disease and Parkinson’s disease. The mechanisms by which chronic PM2.5 exposure leads to these adverse health effects are complex and involve chronic inflammation, oxidative stress, epigenetic changes, and disruption of cellular signaling pathways.
3.3. Specific Health Impacts of Wildfire-Derived PM2.5
While the health impacts of PM2.5 in general are well-established, there is growing evidence that wildfire-derived PM2.5 may have unique and potentially more severe health effects compared to PM2.5 from other sources. Studies have shown that exposure to wildfire smoke is associated with increased respiratory symptoms, hospitalizations, and mortality. Some research suggests that wildfire PM2.5 can cause increased inflammation and oxidative stress in the lungs compared to PM2.5 from other sources. This may be due to the unique chemical composition of wildfire PM2.5, including the presence of elevated levels of organic carbon, elemental carbon, and specific organic compounds. Additionally, wildfire smoke contains a mixture of gases, such as carbon monoxide and acrolein, which can further exacerbate respiratory symptoms. Emerging evidence suggests that wildfire smoke exposure may also have adverse effects on cardiovascular health and mental health. Further research is needed to fully understand the specific health impacts of wildfire-derived PM2.5 and to develop effective strategies for protecting public health during wildfire events. The health impacts of wildfire smoke extend beyond immediate respiratory distress, potentially affecting cardiovascular health, mental well-being, and even cognitive function. Vulnerable populations, including children, the elderly, and individuals with pre-existing conditions, face heightened risks during wildfire events, underscoring the need for targeted interventions and public health campaigns.
3.4. Long-Term Consequences and Potential Links to Cancer and Neurodegenerative Diseases
The potential long-term consequences of chronic PM2.5 exposure, particularly from wildfire smoke, are a growing area of concern. While the evidence is still emerging, studies suggest that chronic PM2.5 exposure may increase the risk of cancer, particularly lung cancer. The mechanisms by which PM2.5 may contribute to cancer development include DNA damage, inflammation, and disruption of cellular signaling pathways. Some studies have also found associations between chronic PM2.5 exposure and other types of cancer, such as breast cancer and bladder cancer. Furthermore, there is growing evidence that chronic PM2.5 exposure may contribute to the development of neurodegenerative diseases such as Alzheimer’s disease and Parkinson’s disease. PM2.5 can enter the brain through the olfactory nerve or the bloodstream, potentially causing inflammation, oxidative stress, and damage to brain cells. More research is needed to fully understand the long-term consequences of chronic PM2.5 exposure and to identify the specific mechanisms by which PM2.5 may contribute to cancer and neurodegenerative diseases. This will require large-scale epidemiological studies with long follow-up periods, as well as mechanistic studies in animal models and cell cultures. The investigation of long-term health consequences requires detailed exposure assessments and sophisticated statistical methods to account for confounding factors. The development of biomarkers that can accurately reflect cumulative PM2.5 exposure and early signs of disease is essential for improving risk assessment and prevention strategies.
Many thanks to our sponsor Esdebe who helped us prepare this research report.
4. PM2.5 Monitoring Techniques
4.1. Ground-Based Monitoring
Ground-based monitoring networks provide continuous measurements of PM2.5 concentrations at fixed locations. These networks typically consist of air quality monitoring stations equipped with sophisticated instruments that measure PM2.5 mass concentrations using techniques such as beta attenuation and tapered element oscillating microbalance (TEOM). Ground-based monitoring networks provide high-resolution data that can be used to track PM2.5 pollution levels over time and to assess compliance with air quality standards. However, ground-based monitoring networks are often limited in their spatial coverage, particularly in rural and remote areas. Furthermore, the cost of establishing and maintaining ground-based monitoring stations can be substantial.
4.2. Satellite-Based Monitoring
Satellite-based monitoring provides a complementary approach to ground-based monitoring, offering broader spatial coverage and the ability to monitor PM2.5 pollution over large areas. Satellites equipped with remote sensing instruments can measure the concentration of aerosols in the atmosphere, which can then be used to estimate PM2.5 concentrations at the ground level. Satellite-based PM2.5 estimates are typically based on measurements of aerosol optical depth (AOD), which is a measure of the amount of light that is absorbed or scattered by aerosols in the atmosphere. Statistical models are then used to relate AOD to PM2.5 concentrations at the ground level. Satellite-based PM2.5 monitoring has several advantages over ground-based monitoring, including its ability to provide global coverage and its relatively low cost. However, satellite-based PM2.5 estimates are subject to uncertainties due to factors such as cloud cover, surface reflectance, and the vertical distribution of aerosols.
4.3. Emerging Monitoring Technologies
In addition to traditional ground-based and satellite-based monitoring techniques, there are several emerging monitoring technologies that show promise for improving PM2.5 monitoring. These include low-cost sensors, mobile monitoring platforms, and advanced data fusion techniques. Low-cost sensors are small, portable devices that can measure PM2.5 concentrations at a fraction of the cost of traditional monitoring instruments. These sensors can be deployed in large numbers to provide high-resolution spatial maps of PM2.5 pollution. Mobile monitoring platforms, such as vehicles equipped with air quality monitoring instruments, can be used to measure PM2.5 concentrations along roadways and in other areas where fixed monitoring stations are not available. Advanced data fusion techniques can be used to combine data from multiple sources, such as ground-based monitors, satellites, and low-cost sensors, to improve the accuracy and spatial coverage of PM2.5 estimates. The integration of these technologies with advanced machine learning techniques is leading to more accurate and timely air quality forecasts, enabling better public health protection.
Many thanks to our sponsor Esdebe who helped us prepare this research report.
5. Mitigation Strategies
5.1. Emission Control Technologies
Emission control technologies are essential for reducing PM2.5 pollution from anthropogenic sources. These technologies include filters, scrubbers, and catalytic converters that remove PM2.5 from exhaust gases. For example, diesel particulate filters (DPFs) can effectively remove PM2.5 from diesel engine exhaust. Scrubbers can remove PM2.5 from industrial emissions by using water or other liquids to trap the particles. Catalytic converters can reduce PM2.5 emissions from vehicles by oxidizing hydrocarbons and carbon monoxide into less harmful substances. In addition to these end-of-pipe control technologies, cleaner fuels and more efficient combustion technologies can also reduce PM2.5 emissions. The development of advanced materials for filters and catalysts is ongoing, with the goal of achieving higher efficiency and lower cost.
5.2. Air Quality Management Policies
Air quality management policies are crucial for reducing PM2.5 pollution at the regional and national levels. These policies can include emission standards for vehicles and industries, regulations on the use of wood stoves and fireplaces, and incentives for the adoption of cleaner technologies. For example, many countries have implemented emission standards for vehicles that limit the amount of PM2.5 that can be emitted. Regulations on the use of wood stoves and fireplaces can reduce PM2.5 emissions from residential heating. Incentives for the adoption of cleaner technologies, such as subsidies for electric vehicles or tax credits for renewable energy, can encourage the transition to cleaner energy sources. Effective air quality management policies require strong enforcement mechanisms and public awareness campaigns to ensure compliance and promote behavior change. Integrating air quality considerations into urban planning and transportation policies is also essential for creating sustainable and healthy cities.
5.3. Personal Protective Measures
Personal protective measures can help to reduce individual exposure to PM2.5, particularly during periods of high pollution or wildfire smoke events. These measures include wearing respirators, using air purifiers, and avoiding outdoor activities. Respirators, such as N95 masks, can effectively filter out PM2.5 particles from the air that is breathed in. Air purifiers with HEPA filters can remove PM2.5 from indoor air. Avoiding outdoor activities during periods of high pollution or wildfire smoke can reduce exposure to PM2.5. It is important to note that personal protective measures are not a substitute for broader emission control and air quality management policies. Rather, they are a temporary measure that can be used to protect individuals from short-term exposure to PM2.5. Educating the public about the risks of PM2.5 exposure and the effectiveness of different protective measures is crucial for empowering individuals to make informed decisions about their health. Furthermore, providing access to affordable and effective respirators and air purifiers is essential for protecting vulnerable populations.
5.4. Strategies to Reduce Exposure to Wildfire Smoke
Reducing exposure to wildfire smoke requires a multifaceted approach that includes prevention, preparedness, and mitigation strategies. Prevention measures include reducing the risk of wildfires through forest management practices, such as prescribed burns and thinning of vegetation. Preparedness measures include developing early warning systems to alert the public to approaching wildfires and providing information on how to protect themselves from smoke exposure. Mitigation strategies include providing clean air shelters for vulnerable populations, distributing respirators, and encouraging people to stay indoors with windows closed. Long-term strategies for mitigating the impacts of wildfire smoke include reducing greenhouse gas emissions to mitigate climate change and investing in research to better understand the health effects of wildfire smoke. A critical aspect of wildfire smoke management is the development of accurate and timely smoke forecasting systems that can predict the movement and concentration of smoke plumes. These systems can help public health officials to issue timely warnings and implement appropriate protective measures. Furthermore, there is a need for improved communication strategies to effectively inform the public about the risks of wildfire smoke exposure and the steps they can take to protect themselves. This includes developing culturally appropriate messages and targeting vulnerable populations with tailored information.
Many thanks to our sponsor Esdebe who helped us prepare this research report.
6. Conclusion
PM2.5 pollution poses a significant threat to global public health. This review has provided a comprehensive overview of PM2.5, encompassing its diverse sources, health impacts, monitoring techniques, and mitigation strategies. While significant progress has been made in understanding and addressing PM2.5 pollution, many challenges remain. These challenges include the need for more accurate and comprehensive monitoring of PM2.5, a better understanding of the specific health effects of PM2.5 from different sources, and the development of more effective mitigation strategies. In particular, the increasing frequency and intensity of wildfires pose a growing threat to public health, requiring targeted research and interventions to reduce exposure to wildfire smoke. Future research should focus on developing more advanced monitoring technologies, conducting detailed characterization of the chemical composition and toxicity of PM2.5 from different sources, and evaluating the effectiveness of different mitigation strategies. Furthermore, international cooperation is essential for addressing PM2.5 pollution, as air pollution knows no borders. By working together, researchers, policymakers, and public health professionals can develop and implement effective strategies to reduce PM2.5 pollution and protect public health.
Future research should also focus on the potential long-term health effects of chronic PM2.5 exposure, including links to cancer and neurodegenerative diseases. The development of biomarkers that can accurately reflect cumulative PM2.5 exposure and early signs of disease is essential for improving risk assessment and prevention strategies. Furthermore, there is a need for more research on the effectiveness of different personal protective measures, such as respirators and air purifiers, in reducing exposure to PM2.5. This research should consider the specific needs of vulnerable populations, such as children, the elderly, and individuals with pre-existing respiratory or cardiovascular diseases.
Many thanks to our sponsor Esdebe who helped us prepare this research report.
References
- Anderson, J. O., Thundiyil, J. G., & Stolbach, A. (2012). Clearing the air: A review of the effects of particulate matter air pollution on human health. Journal of Medical Toxicology, 8(2), 166-175.
- Pope, C. A., III, Burnett, R. T., Thun, M. J., Calle, E. E., Krewski, D., Ito, K., & Thurston, G. D. (2002). Lung cancer, cardiopulmonary mortality, and long-term exposure to fine particulate air pollution. JAMA, 287(9), 1132-1141.
- Lelieveld, J., Evans, J. S., Fnais, I., Giannadaki, D., & Pozzer, A. (2015). The contribution of outdoor air pollution sources to premature mortality on a global scale. Nature, 525(7569), 367-371.
- Reid, C. E., Brauer, M., Johnston, F. H., Jerrett, M., Balmes, J. R., Elliott, C. T., … & Abernethy, R. (2016). Critical review of health impacts of wildfire smoke exposure. Environmental Health Perspectives, 124(9), 1334-1343.
- Westerling, A. L. (2016). Increasing western US forest wildfire activity: sensitivity to changes in the timing of spring. Philosophical Transactions of the Royal Society B: Biological Sciences, 371(1696), 20150178.
- Liu, J. C., Pereira, G., Uhl, S. A., Bravo, M. A., & Bell, M. L. (2015). A systematic review of the impact of wildfire smoke on respiratory health. Environmental Research, 136, 553-562.
- Cascio, W. E. (2018). Cardiovascular health and air pollution. Journal of the American College of Cardiology, 72(5), 622-631.
- Calderón-Garcidueñas, L., González-Maciel, A., Reynoso-Robles, R., Delgado-Chávez, R., Torres-Jardón, R., Ávila-Ramírez, J., … & Dotigny, F. (2015). Air pollution and neuroinflammation: a clarifying review of the underlying mechanisms. Frontiers in Neurology, 5, 267.
- Malley, C. S., Kuylenstierna, J. C. I., Vallack, H. W., Tingey, D., & Pearson, R. G. (2017). Global non-compliance with World Health Organization air quality guidelines. Environmental Science & Technology, 51(21), 11998-12006.
- Johnston, F. H., Henderson, S. B., Balmes, J. R., Shah, S. P., & Brauer, M. (2012). Estimating long-term mortality risks from wildfire smoke. Environmental Health Perspectives, 120(5), 695-701.
- Kelly, F. J., & Fussell, J. C. (2017). Air pollution and public health: emerging hazards and improved understanding of risk. Environmental Geochemistry and Health, 39(3), 631-649.
- Stockwell, C. E., McMeeking, G. R., Andreae, M. O., Kreidenweis, S. M., & Clements, C. B. (2016). Chemical and microphysical properties of wildfire smoke across the US Intermountain West. Atmospheric Chemistry and Physics, 16(18), 11893-11914.
- Ward, T. J., & Palmer, S. J. (2011). The efficiency of rural household coal combustion and associated pollutant emissions. Atmospheric Environment, 45(36), 6530-6538.
- National Ambient Air Quality Standards for Particulate Matter. United States Environmental Protection Agency (EPA), 40 CFR part 50.
- Gan, R. W., Allen, R. W., Brauer, M., & Davies, H. W. (2017). Ambient particle size and source apportionment of daily mortality in Vancouver, Canada. Environmental Health, 16(1), 67.
- Delfino, R. J., Brummel, S., Wu, J., Stern, H., Ostro, B., Lipsett, M., … & Tjoa, T. (2009). The relationship of respiratory and cardiovascular hospital admissions to the southern California wildfires of 2003. Occupational and Environmental Medicine, 66(3), 189-196.
- Reisen, F., & Hopke, P. K. (2008). Review of the atmospheric fate and transport of ultrafine particles. Atmospheric Environment, 42(28), 7005-7039.
- Aguilera, R., Corvalán, C. M., Pérez-García, F., & Barraza, F. (2016). Health effects of wildfire smoke exposure in the elderly: a systematic review. Environmental Research, 148, 576-585.
- Jones, N. R., McGranahan, D. P., Wheeler, A. J., & Johnston, F. H. (2020). Wildfire smoke and human health: A systematic review of exposure assessment approaches. Environmental Research, 186, 109569.
- Cascio, W. E. (2018). Cardiovascular health and air pollution. Journal of the American College of Cardiology, 72(5), 622–631.
The review highlights the increasing significance of wildfires as PM2.5 sources. What are the most promising strategies for communities to proactively manage vegetation and reduce fuel loads in fire-prone areas, considering both ecological impacts and cost-effectiveness?
That’s a great question! Proactive vegetation management is key. Thinning forests and prescribed burns, when carefully planned, are effective and can mimic natural fire cycles, promoting biodiversity. Balancing ecological needs with cost is crucial, and local solutions often work best!
Editor: MedTechNews.Uk
Thank you to our Sponsor Esdebe
Given that wildfire PM2.5 seems more toxic, shouldn’t we prioritize funding for specialized air filtration systems designed specifically for wildfire smoke in vulnerable community buildings like schools and hospitals? Or are we just supposed to accept the smoky skies as the new normal?
That’s a crucial point about specialized air filtration! Investing in these systems for vulnerable communities, as well as affordable home based systems is vital. We need to explore innovations in filtration technology and assess their cost-effectiveness. It’s about preparedness, not just acceptance. What are your thoughts on incentivizing adoption, especially for those most at risk?
Editor: MedTechNews.Uk
Thank you to our Sponsor Esdebe
So, PM2.5 is the bad guy we can’t see causing all sorts of mischief from respiratory issues to potential links with neurodegenerative diseases? Sounds like we need superhero air filtration systems, stat!
Absolutely! The potential links between PM2.5 and neurodegenerative diseases are a serious concern. Further research into this area is crucial. The development and deployment of advanced air filtration is critical, especially for vulnerable populations.
Editor: MedTechNews.Uk
Thank you to our Sponsor Esdebe
Considering the significant variability in the chemical composition of PM2.5 from wildfires, a standardized method for assessing and communicating its specific toxicity would be highly beneficial for public health messaging and individual risk assessment.
That’s an excellent point! Standardizing the communication of PM2.5 toxicity would greatly improve public understanding and empower individuals to make informed decisions. Perhaps a simple, tiered warning system, similar to UV index, could be effective for different wildfire smoke compositions. This could facilitate quicker responses. What are your thoughts?
Editor: MedTechNews.Uk
Thank you to our Sponsor Esdebe