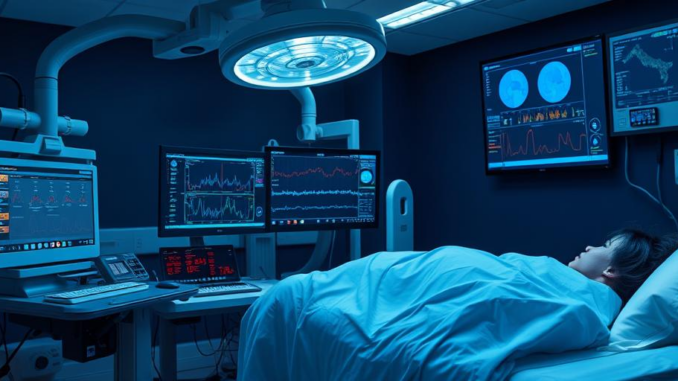
Abstract
Polysomnography (PSG) remains the gold standard for assessing sleep architecture and diagnosing sleep disorders. However, its inherent limitations and the growing demand for more accessible and comprehensive sleep monitoring have spurred significant technological and methodological advancements. This research report provides an in-depth overview of PSG, encompassing its fundamental principles, established applications, current challenges, and emerging trends. We delve into the intricate details of PSG’s various signal modalities (EEG, EOG, EMG, ECG, respiratory effort, oximetry), focusing on the physiological bases of these signals and the standardized scoring criteria used in clinical practice. We critically evaluate the accuracy and limitations of traditional PSG, particularly its first-night effect, high cost, and labor-intensive nature. Finally, we explore the promising avenues of research and development, including the integration of artificial intelligence for automated sleep scoring, the development of novel sensors and wearable technologies for home sleep monitoring, and the application of PSG in elucidating the neurobiological mechanisms underlying sleep disorders and cognitive function. This report aims to provide a comprehensive resource for experts in sleep medicine and neuroscience, fostering a deeper understanding of PSG’s current state and its future trajectory.
Many thanks to our sponsor Esdebe who helped us prepare this research report.
1. Introduction
Sleep is a fundamental physiological process crucial for a wide range of biological functions, including cognitive performance, immune function, and metabolic regulation. Disruptions in sleep, whether due to intrinsic sleep disorders or extrinsic factors, can have profound and far-reaching consequences on an individual’s health and well-being. Polysomnography (PSG), often referred to as a sleep study, stands as the cornerstone of sleep disorder diagnosis and management. It is a comprehensive multi-parametric recording of physiological signals during sleep, providing a detailed assessment of sleep architecture, sleep-related events, and underlying physiological abnormalities.
Since its inception, PSG has evolved from a relatively simple EEG-based assessment of sleep stages to a sophisticated multi-channel recording incorporating a diverse array of physiological measures. This evolution has been driven by advances in sensor technology, signal processing techniques, and a deeper understanding of the neurophysiological mechanisms governing sleep. However, despite its widespread adoption and clinical utility, PSG faces several challenges, including its high cost, limited accessibility, and the inherent limitations of a laboratory-based setting. These challenges have spurred significant research efforts aimed at developing alternative sleep monitoring technologies and improving the efficiency and accuracy of PSG.
This report aims to provide a comprehensive overview of PSG, addressing its fundamental principles, established applications, current limitations, and emerging trends. We will delve into the intricacies of PSG signal acquisition and analysis, critically evaluate its accuracy and limitations, and explore the promising avenues of research and development aimed at overcoming these challenges and expanding the application of PSG in sleep medicine and neuroscience.
Many thanks to our sponsor Esdebe who helped us prepare this research report.
2. Principles of Polysomnography
At its core, PSG involves the simultaneous and continuous recording of multiple physiological parameters during sleep. These parameters are carefully selected to provide a comprehensive assessment of sleep architecture, sleep-related events, and underlying physiological abnormalities. The key signal modalities employed in PSG include electroencephalography (EEG), electrooculography (EOG), electromyography (EMG), electrocardiography (ECG), respiratory effort and airflow, and pulse oximetry.
2.1. Electroencephalography (EEG)
EEG is the cornerstone of PSG, providing a direct measure of brain electrical activity. Scalp electrodes are strategically placed according to the international 10-20 system to record voltage fluctuations generated by neuronal activity. These fluctuations are characterized by distinct frequency bands, amplitude patterns, and spatial distributions, which are used to define different sleep stages. The standard EEG montage typically includes derivations from frontal (F), central (C), parietal (P), and occipital (O) regions of the scalp, allowing for a comprehensive assessment of brain activity across different cortical areas.
The EEG signals recorded during PSG are used to differentiate between wakefulness, non-rapid eye movement (NREM) sleep stages (N1, N2, N3), and rapid eye movement (REM) sleep. Wakefulness is characterized by alpha activity (8-12 Hz) when eyes are closed and beta activity (>13 Hz) when eyes are open and alert. N1 sleep is marked by a slowing of the EEG frequency and the appearance of vertex waves, sharp negative deflections occurring maximally over the central scalp. N2 sleep is characterized by the presence of sleep spindles (11-16 Hz) and K-complexes, large biphasic waves that are often triggered by external stimuli. N3 sleep, also known as slow-wave sleep or deep sleep, is dominated by high-amplitude slow waves (0.5-2 Hz). REM sleep is characterized by a relatively low-amplitude, mixed-frequency EEG pattern, similar to wakefulness, but accompanied by rapid eye movements and muscle atonia.
2.2. Electrooculography (EOG)
EOG measures the electrical potential generated by eye movements. Electrodes are placed near the outer canthus of each eye to record horizontal eye movements and above and below one eye to record vertical eye movements. EOG is essential for identifying REM sleep, which is characterized by bursts of rapid eye movements. EOG also plays a role in detecting eye movements associated with other sleep disorders, such as periodic limb movements during sleep (PLMS).
2.3. Electromyography (EMG)
EMG measures muscle activity. In PSG, EMG electrodes are typically placed on the chin (submental EMG) and on the legs (tibialis anterior EMG). The submental EMG is used to assess muscle tone during sleep, which is typically reduced in NREM sleep and absent in REM sleep (muscle atonia). Leg EMG is used to detect PLMS, which are repetitive movements of the legs that can disrupt sleep and lead to daytime sleepiness.
2.4. Electrocardiography (ECG)
ECG measures the electrical activity of the heart. ECG is used to monitor heart rate and rhythm during sleep and to detect any cardiac arrhythmias that may be associated with sleep apnea or other sleep disorders.
2.5. Respiratory Effort and Airflow
Respiratory effort is measured using chest and abdominal belts that detect changes in circumference associated with breathing. Airflow is measured using a nasal cannula or thermistor that detects changes in airflow at the nose and mouth. These measures are used to identify apneas (cessation of breathing) and hypopneas (reduction in airflow) that are characteristic of sleep apnea.
2.6. Pulse Oximetry
Pulse oximetry measures the oxygen saturation of the blood. A sensor is typically placed on a finger or earlobe to measure the percentage of hemoglobin that is saturated with oxygen. Pulse oximetry is used to detect desaturations (drops in oxygen saturation) that may be associated with sleep apnea or other respiratory disorders.
Many thanks to our sponsor Esdebe who helped us prepare this research report.
3. Sleep Scoring and Interpretation
Following the completion of a PSG recording, the data is meticulously analyzed and scored by trained sleep technologists. The scoring process involves visually inspecting the EEG, EOG, and EMG signals and assigning each 30-second epoch to a specific sleep stage based on standardized scoring criteria. The American Academy of Sleep Medicine (AASM) Manual for the Scoring of Sleep and Associated Events is the definitive resource for sleep scoring guidelines and is widely used in clinical practice and research [1].
The AASM scoring rules define specific EEG, EOG, and EMG criteria for each sleep stage. For example, N1 sleep is characterized by a slowing of the EEG frequency and the appearance of vertex waves, while N2 sleep is characterized by the presence of sleep spindles and K-complexes. N3 sleep is defined by the presence of high-amplitude slow waves, and REM sleep is characterized by a low-amplitude, mixed-frequency EEG pattern, rapid eye movements, and muscle atonia. The sleep technologist also identifies and scores other sleep-related events, such as arousals, respiratory events (apneas, hypopneas), and limb movements.
Once the sleep scoring is complete, a sleep report is generated that summarizes the patient’s sleep architecture, including the total sleep time, sleep latency (time to fall asleep), REM latency (time to first REM sleep episode), sleep efficiency (percentage of time in bed spent asleep), and the percentage of time spent in each sleep stage. The sleep report also includes information about the number and duration of respiratory events and limb movements, as well as the patient’s oxygen saturation levels. The sleep report is then reviewed by a sleep physician, who interprets the data in the context of the patient’s clinical history and physical examination findings to arrive at a diagnosis and develop a treatment plan.
Many thanks to our sponsor Esdebe who helped us prepare this research report.
4. Applications of Polysomnography
PSG is widely used in the diagnosis and management of a variety of sleep disorders, including:
- Sleep Apnea: PSG is the gold standard for diagnosing obstructive sleep apnea (OSA) and central sleep apnea (CSA). PSG can quantify the severity of sleep apnea by measuring the apnea-hypopnea index (AHI), which is the number of apneas and hypopneas per hour of sleep. PSG can also identify the type of sleep apnea (obstructive, central, or mixed) and assess the impact of sleep apnea on oxygen saturation levels and cardiac function.
- Insomnia: PSG can be used to evaluate patients with chronic insomnia to rule out other sleep disorders, such as sleep apnea or restless legs syndrome (RLS), and to assess the severity of insomnia by measuring sleep latency, total sleep time, and sleep efficiency. While not always necessary for diagnosis, it can be useful in complex cases or when other conditions are suspected.
- Narcolepsy: PSG is essential for diagnosing narcolepsy. In addition to the overnight PSG, a multiple sleep latency test (MSLT) is typically performed the following day. The MSLT measures the time it takes to fall asleep (sleep latency) and the occurrence of REM sleep during daytime naps. Patients with narcolepsy typically have short sleep latencies and enter REM sleep rapidly during the MSLT.
- Restless Legs Syndrome (RLS) and Periodic Limb Movement Disorder (PLMD): PSG can be used to diagnose RLS and PLMD by measuring the number of PLMS per hour of sleep (PLMS index). While RLS is often diagnosed clinically, PSG helps confirm PLMD as a contributing factor to sleep disturbance.
- Parasomnias: PSG can be helpful in evaluating patients with parasomnias, such as sleepwalking, sleep terrors, and REM sleep behavior disorder (RBD). PSG can document the occurrence of these events and rule out other sleep disorders that may mimic parasomnias.
- Circadian Rhythm Disorders: PSG can be used to assess circadian rhythm disorders, such as delayed sleep phase syndrome (DSPS) and advanced sleep phase syndrome (ASPS). By monitoring sleep patterns over several days, PSG can help determine the patient’s endogenous circadian rhythm and identify any discrepancies between the patient’s desired sleep time and their actual sleep time.
- Seizure Disorders: Polysomnography can be helpful to distinguish between nocturnal seizures and other sleep disorders, and can provide information about the relationship between sleep stages and seizure activity. The EEG component of PSG is critical in identifying seizure activity and characterizing its features during sleep.
Beyond diagnostic applications, PSG is also a valuable tool for research. It is used to study the neurophysiological mechanisms of sleep, the effects of sleep deprivation on cognitive function and health, and the impact of various interventions on sleep quality. PSG is also used in clinical trials to evaluate the efficacy of new treatments for sleep disorders.
Many thanks to our sponsor Esdebe who helped us prepare this research report.
5. Limitations of Traditional Polysomnography
Despite its status as the gold standard for sleep assessment, traditional PSG is not without its limitations. These limitations can impact the accuracy and reliability of PSG results and may hinder its widespread adoption and accessibility.
- First-Night Effect: The first-night effect refers to the phenomenon whereby sleep architecture is altered on the first night of PSG due to the unfamiliar environment and the presence of recording equipment. This can result in reduced sleep time, increased sleep latency, and reduced REM sleep [2]. To mitigate the first-night effect, some sleep laboratories routinely discard the data from the first night of PSG and only analyze the data from the second night. However, this increases the cost and inconvenience of PSG.
- High Cost and Limited Accessibility: PSG is a relatively expensive and labor-intensive procedure. It requires specialized equipment, trained sleep technologists, and a dedicated sleep laboratory. This limits the accessibility of PSG, particularly in rural areas and for patients with limited financial resources. The cost of PSG can be a significant barrier to diagnosis and treatment for many patients.
- Labor-Intensive Nature: The manual scoring of PSG data is a time-consuming and labor-intensive process. It requires trained sleep technologists to visually inspect the EEG, EOG, and EMG signals and assign each epoch to a specific sleep stage. The manual scoring process is also prone to inter-scorer variability, which can affect the reliability of PSG results. AI is being applied to address this issue.
- Artificial Environment: PSG is typically performed in a sleep laboratory, which is an artificial environment that may not accurately reflect the patient’s typical sleep environment. This can affect the patient’s sleep patterns and may not provide an accurate representation of their sleep quality at home. Home sleep apnea testing (HSAT) addresses this limitation, but is limited in its ability to detect a broad spectrum of sleep disorders.
- Limited Ecological Validity: Traditional PSG provides a snapshot of sleep over a single night, which may not be representative of the patient’s typical sleep patterns over a longer period. Sleep patterns can vary from night to night due to a variety of factors, such as stress, travel, and illness. Long term monitoring can address this limitation.
Many thanks to our sponsor Esdebe who helped us prepare this research report.
6. Advancements and Emerging Technologies
The limitations of traditional PSG have spurred significant research and development efforts aimed at improving the accuracy, accessibility, and efficiency of sleep monitoring. These efforts have led to the development of several promising advancements and emerging technologies.
6.1. Automated Sleep Scoring
Automated sleep scoring algorithms use machine learning and artificial intelligence to automatically score PSG data. These algorithms can analyze the EEG, EOG, and EMG signals and assign each epoch to a specific sleep stage without the need for manual scoring by a sleep technologist. Automated sleep scoring algorithms have the potential to significantly reduce the labor and cost associated with PSG and to improve the reliability and consistency of sleep scoring [3].
While automated sleep scoring algorithms have shown promising results, they are not yet perfect. The accuracy of these algorithms can vary depending on the complexity of the sleep disorder and the quality of the PSG data. Furthermore, automated sleep scoring algorithms are not able to detect all sleep-related events, such as arousals and respiratory events. Therefore, it is important for a sleep physician to review the automated sleep scoring results and to make any necessary corrections.
6.2. Home Sleep Monitoring
Home sleep monitoring devices allow patients to record their sleep data in the comfort of their own homes. These devices typically use wearable sensors to measure physiological parameters, such as EEG, EOG, EMG, heart rate, and respiratory effort. Home sleep monitoring devices have the potential to improve the accessibility and convenience of sleep monitoring and to provide a more accurate representation of the patient’s typical sleep patterns [4].
There are several different types of home sleep monitoring devices available, ranging from simple actigraphy devices that measure movement to more sophisticated devices that record multiple physiological parameters. The choice of device depends on the specific clinical question being asked and the patient’s individual needs. While home sleep apnea testing (HSAT) is widely accepted, the use of home monitoring for other conditions is an area of active research.
6.3. Novel Sensors and Wearable Technologies
Researchers are actively developing novel sensors and wearable technologies for sleep monitoring. These include:
- Single-Channel EEG Devices: These devices use a single EEG electrode to record brain activity and estimate sleep stages. They offer a simplified and more comfortable alternative to traditional multi-channel EEG recordings.
- Ballistocardiography (BCG): BCG measures the mechanical forces generated by the heart as it pumps blood throughout the body. BCG can be used to estimate heart rate, respiratory rate, and sleep stages.
- Photoplethysmography (PPG): PPG measures changes in blood volume in the skin. PPG can be used to estimate heart rate, respiratory rate, and sleep stages. Often found in wearables for convenience.
- Acoustic Sensors: Acoustic sensors can be used to detect snoring and other sleep-related sounds, which can provide information about respiratory events and sleep quality.
These novel sensors and wearable technologies have the potential to further improve the accessibility and convenience of sleep monitoring and to provide more detailed information about sleep physiology.
6.4. Integration with Other Technologies
PSG is increasingly being integrated with other technologies, such as:
- Brain Stimulation: Transcranial magnetic stimulation (TMS) and transcranial direct current stimulation (tDCS) can be combined with PSG to study the effects of brain stimulation on sleep architecture and cognitive function. This combination can be used to treat sleep disorders and to improve cognitive performance.
- Neuroimaging: Functional magnetic resonance imaging (fMRI) and positron emission tomography (PET) can be combined with PSG to study the neural correlates of sleep and sleep disorders. This combination can provide insights into the neurobiological mechanisms underlying sleep and wakefulness.
- Genetics: Genetic studies are being combined with PSG to identify genes that are associated with sleep disorders. This combination can lead to the development of new diagnostic and therapeutic strategies for sleep disorders.
Many thanks to our sponsor Esdebe who helped us prepare this research report.
7. Future Directions and Conclusion
Polysomnography has revolutionized the field of sleep medicine, providing a comprehensive and objective assessment of sleep architecture and sleep-related events. However, traditional PSG is not without its limitations, including its high cost, limited accessibility, and labor-intensive nature. These limitations have spurred significant research and development efforts aimed at improving the accuracy, accessibility, and efficiency of sleep monitoring.
Emerging technologies, such as automated sleep scoring, home sleep monitoring, novel sensors and wearable technologies, and integration with other technologies, hold great promise for transforming the field of sleep medicine. These technologies have the potential to improve the diagnosis and management of sleep disorders, to enhance our understanding of the neurophysiological mechanisms of sleep, and to promote better sleep health for all.
Future research should focus on further refining and validating these emerging technologies, on developing new algorithms for automated sleep scoring, on improving the accuracy and reliability of home sleep monitoring devices, and on exploring the potential of these technologies to personalize sleep medicine. In my opinion, the development of robust, validated, and cost-effective home sleep monitoring solutions is crucial for addressing the growing burden of sleep disorders and for improving access to care for patients in underserved communities. This requires a concerted effort involving researchers, clinicians, industry partners, and regulatory agencies.
In conclusion, polysomnography remains the cornerstone of sleep medicine, but its future lies in the integration of emerging technologies and innovative approaches. By embracing these advancements, we can unlock new insights into the complexities of sleep and develop more effective strategies for promoting sleep health and improving the lives of individuals affected by sleep disorders.
Many thanks to our sponsor Esdebe who helped us prepare this research report.
References
[1] Berry, R. B., Brooks, R., Gamaldo, C. E., Harding, S. M., Lloyd, R. M., Quan, S. F., … & Vaughn, B. V. (2017). The AASM manual for the scoring of sleep and associated events: Rules, terminology and technical specifications. American Academy of Sleep Medicine.
[2] Agnew, H. W., Webb, W. B., & Williams, R. L. (1966). The first night effect: An EEG study of sleep. Psychophysiology, 2(4), 263-266.
[3] Stephansen, J. B., Olesen, A. N., Jennum, P., & Hansen, M. B. (2018). Automated sleep stage scoring with deep neural networks. Sleep, 41(3), zsx223.
[4] Bakker, J. P., Rijsman, R. M., Leijten, F. S., van Laar, J. O. E., van Wezel, R. J. A., & Overeem, S. (2020). Ambulatory polysomnography: A systematic review. Journal of Clinical Neurophysiology, 37(1), 1-13.
So, PSG is the gold standard, huh? Sounds like it’s time for a sleep-off between PSG and these new wearable technologies. Winner gets bragging rights and maybe a power nap! How long before home monitoring completely steals PSG’s crown, do you think?
That’s a fun way to look at it! I think wearable tech has made incredible strides and continues to improve, but PSG still offers a level of detail that’s hard to match, particularly when diagnosing complex sleep disorders. It will be exciting to see how the two approaches continue to evolve and complement each other in the future! I think the “crown” will be shared!
Editor: MedTechNews.Uk
Thank you to our Sponsor Esdebe