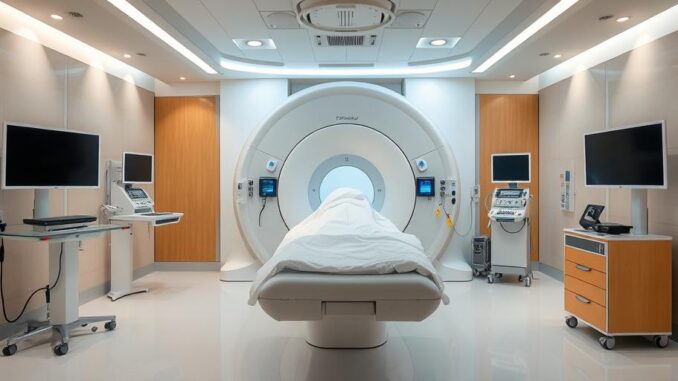
Abstract
Proton therapy (PT) represents a significant advancement in radiation oncology, offering the potential for improved dose conformity and reduced off-target radiation exposure compared to conventional photon-based radiotherapy. This report provides a comprehensive review of PT, encompassing its physical principles, clinical applications across various cancer types, technological developments, economic considerations, and future research directions. We delve into the advantages and limitations of PT, focusing on its unique dose distribution characteristics and its implications for treatment planning and clinical outcomes. The report explores the different types of PT delivery systems, including passively scattered and pencil beam scanning techniques, and examines the current landscape of PT facilities worldwide. Furthermore, we analyze the cost-effectiveness of PT and discuss the challenges associated with access and reimbursement. Finally, we highlight emerging research areas, such as FLASH radiotherapy, adaptive PT, and the use of PT in combination with immunotherapy, and discuss the potential of these advancements to further improve cancer treatment outcomes.
Many thanks to our sponsor Esdebe who helped us prepare this research report.
1. Introduction
Radiation therapy plays a crucial role in the treatment of a wide range of cancers. Conventional radiotherapy utilizes photons (X-rays and gamma rays), which deposit energy along their path through the body, resulting in radiation exposure to tissues both before and after the tumor target. This can lead to acute and late toxicities, impacting quality of life and potentially increasing the risk of secondary malignancies. Proton therapy (PT) offers a distinct advantage by virtue of its unique dose deposition characteristics. Protons deposit most of their energy at a specific depth, known as the Bragg peak, allowing for precise targeting of the tumor while minimizing radiation exposure to surrounding healthy tissues. This feature is particularly beneficial in treating tumors located near critical organs or in pediatric patients, where minimizing long-term side effects is paramount.
While the concept of particle therapy dates back to the 1940s, the clinical implementation of PT has been slower compared to photon therapy due to the higher cost and complexity of proton accelerator technology. However, significant advancements in PT technology over the past few decades have led to increased accessibility and improved treatment techniques. The development of pencil beam scanning (PBS) technology, in particular, has enabled highly conformal dose distributions, further enhancing the therapeutic potential of PT.
This report aims to provide a comprehensive overview of PT, covering its underlying principles, clinical applications, technological advancements, economic considerations, and future directions. We will explore the advantages and limitations of PT compared to conventional radiotherapy and discuss the challenges associated with its widespread adoption.
Many thanks to our sponsor Esdebe who helped us prepare this research report.
2. Physical Principles of Proton Therapy
The fundamental difference between proton therapy and photon therapy lies in the way these particles interact with matter and deposit energy. Photons are electrically neutral and interact with tissue through a combination of photoelectric effect, Compton scattering, and pair production. As photons traverse the body, they gradually lose energy, resulting in an exponential decrease in dose with increasing depth. This means that tissues both before and after the target volume receive significant radiation exposure.
Protons, on the other hand, are positively charged particles that lose energy primarily through ionization and excitation of atoms. The energy loss per unit path length, known as the linear energy transfer (LET), increases as the proton slows down. This results in a characteristic depth-dose profile with a low entrance dose, a plateau region of relatively constant dose, and a sharp peak of high dose deposition at the end of the proton’s range, called the Bragg peak. Beyond the Bragg peak, the dose drops off rapidly to near zero. This unique property allows for highly conformal dose distributions, where the majority of the radiation dose is delivered to the tumor while sparing surrounding healthy tissues.
The depth of the Bragg peak is determined by the initial energy of the proton beam. By modulating the energy of the protons, it is possible to spread the Bragg peak over the entire tumor volume, creating a uniform dose distribution within the target. This technique is known as spread-out Bragg peak (SOBP). Modern PT systems utilize PBS technology, which allows for precise scanning of a narrow proton beam across the tumor volume, further improving dose conformity and sparing of normal tissues.
Many thanks to our sponsor Esdebe who helped us prepare this research report.
3. Clinical Applications of Proton Therapy
Proton therapy has been used to treat a wide range of cancers, with the most compelling clinical benefits observed in tumors located near critical structures or in pediatric patients. The ability to deliver high doses to the tumor while minimizing exposure to surrounding healthy tissues makes PT an attractive option for treating tumors of the brain, head and neck, lung, prostate, and gastrointestinal tract. Pediatric cancers are a particularly important area of focus for PT due to the increased risk of long-term side effects from radiation exposure in young patients.
3.1. Pediatric Cancers
Children are more susceptible to the long-term effects of radiation exposure than adults. These effects can include growth retardation, endocrine dysfunction, cognitive impairment, and an increased risk of secondary malignancies. Proton therapy can significantly reduce the risk of these complications by minimizing radiation exposure to developing organs and tissues. Several studies have demonstrated the benefits of PT in pediatric cancers, including medulloblastoma, ependymoma, rhabdomyosarcoma, and Ewing sarcoma [1, 2]. For example, a study comparing PT to photon therapy in children with medulloblastoma showed a significant reduction in radiation exposure to the brainstem and other critical structures [3]. This resulted in improved neurocognitive outcomes and a reduced risk of long-term complications.
3.2. Brain Tumors
Proton therapy has shown promise in the treatment of various brain tumors, including gliomas, meningiomas, and acoustic neuromas. The precise dose delivery of PT can minimize damage to critical structures such as the optic nerves, brainstem, and hippocampus, potentially reducing the risk of vision loss, neurological deficits, and cognitive impairment. Studies have shown that PT can improve local control rates and overall survival in patients with certain types of brain tumors [4, 5].
3.3. Head and Neck Cancers
Head and neck cancers are often located near critical structures such as the spinal cord, salivary glands, and swallowing muscles. Proton therapy can help to spare these structures, reducing the risk of xerostomia (dry mouth), dysphagia (difficulty swallowing), and other debilitating side effects. Several studies have demonstrated the benefits of PT in head and neck cancers, including improved quality of life and reduced rates of severe toxicities [6, 7].
3.4. Lung Cancer
Lung cancer is a leading cause of cancer-related deaths worldwide. Proton therapy can be used to treat both early-stage and locally advanced lung cancer. The ability to deliver high doses to the tumor while sparing the surrounding lung tissue and heart can potentially improve local control rates and reduce the risk of radiation-induced pneumonitis and cardiac toxicity. While randomized trials are ongoing, retrospective studies have suggested a benefit of PT compared to photon therapy in select patients with lung cancer [8, 9].
3.5. Prostate Cancer
Prostate cancer is one of the most common cancers in men. Proton therapy can be used as an alternative to conventional radiotherapy or surgery in the treatment of localized prostate cancer. The ability to precisely target the prostate gland while sparing surrounding tissues such as the rectum and bladder can potentially reduce the risk of urinary and bowel complications. Several studies have shown that PT can achieve comparable or even better outcomes than photon therapy in terms of biochemical control and toxicity [10, 11]. However, the cost-effectiveness of PT for prostate cancer remains a subject of debate.
3.6 Other Cancers
PT is also used to treat other cancers in areas such as the pancreas, liver and eye.
Many thanks to our sponsor Esdebe who helped us prepare this research report.
4. Technological Advancements in Proton Therapy
Significant advancements in PT technology have occurred over the past few decades, leading to improved treatment techniques and increased accessibility. These advancements include the development of PBS technology, image-guided PT, and adaptive PT.
4.1. Pencil Beam Scanning (PBS)
PBS is a modern PT technique that allows for precise scanning of a narrow proton beam across the tumor volume. Unlike passively scattered PT, which uses scattering foils and collimators to shape the proton beam, PBS directly modulates the proton beam energy and position to conform to the tumor shape. This results in highly conformal dose distributions and improved sparing of normal tissues. PBS also allows for intensity-modulated proton therapy (IMPT), where the proton beam intensity is varied across the tumor volume to further optimize the dose distribution.
4.2. Image-Guided Proton Therapy (IGPT)
IGPT uses advanced imaging techniques such as cone-beam computed tomography (CBCT) and magnetic resonance imaging (MRI) to visualize the tumor and surrounding tissues before and during treatment. This allows for precise alignment of the proton beam with the tumor and ensures that the radiation dose is delivered accurately. IGPT can also be used to monitor tumor shrinkage and changes in patient anatomy during treatment, allowing for adaptive adjustments to the treatment plan.
4.3. Adaptive Proton Therapy (APT)
APT involves modifying the treatment plan during the course of treatment to account for changes in tumor size, shape, or position. This can be achieved by repeating imaging scans and re-optimizing the treatment plan based on the updated information. APT can help to improve treatment outcomes by ensuring that the radiation dose is delivered accurately to the tumor, even if the tumor changes during treatment.
4.4 FLASH Radiotherapy
FLASH radiotherapy is an experimental form of radiation therapy that delivers very high doses of radiation in extremely short periods of time (typically less than 1 second). Preclinical studies have shown that FLASH radiotherapy can spare normal tissues from radiation damage while maintaining or even enhancing tumor control. Proton therapy is well-suited for FLASH radiotherapy due to its ability to deliver high doses of radiation with precise targeting. While FLASH radiotherapy is still in its early stages of development, it has the potential to revolutionize cancer treatment.
Many thanks to our sponsor Esdebe who helped us prepare this research report.
5. Proton Therapy Facilities and Accessibility
The number of PT facilities worldwide has been steadily increasing over the past few decades. As of 2023, there are over 100 operational PT centers globally, with the majority located in the United States, Europe, and Asia. However, access to PT remains limited due to the high cost and complexity of the technology.
5.1. Location and Distribution
The distribution of PT facilities is uneven, with most centers concentrated in wealthier countries and regions. This disparity in access raises ethical concerns about equitable distribution of healthcare resources. Efforts are underway to expand access to PT in underserved areas, but significant challenges remain.
5.2. Types of Facilities
PT facilities can be broadly categorized into hospital-based and freestanding centers. Hospital-based centers are integrated within a larger hospital complex, providing access to a wide range of medical specialties and resources. Freestanding centers are independent facilities that focus solely on PT. The choice between hospital-based and freestanding centers depends on various factors, including patient volume, cost, and staffing considerations.
Many thanks to our sponsor Esdebe who helped us prepare this research report.
6. Cost and Reimbursement of Proton Therapy
Proton therapy is significantly more expensive than conventional photon therapy. The higher cost is due to the capital investment required for the proton accelerator and associated infrastructure, as well as the operational costs of maintaining the facility and staffing it with specialized personnel. The cost of PT can vary depending on the type of cancer being treated, the complexity of the treatment plan, and the location of the facility.
6.1. Cost-Effectiveness Analysis
The cost-effectiveness of PT has been a subject of debate. While PT may be more expensive upfront, it has the potential to reduce long-term healthcare costs by minimizing radiation-induced complications and improving quality of life. Several studies have attempted to assess the cost-effectiveness of PT for different cancer types, but the results have been mixed. A comprehensive cost-effectiveness analysis should consider not only the direct costs of treatment but also the indirect costs associated with side effects, lost productivity, and long-term care.
6.2. Reimbursement Policies
Reimbursement policies for PT vary widely across different countries and healthcare systems. In some countries, PT is fully covered by public or private insurance, while in others, coverage may be limited or unavailable. The lack of consistent reimbursement policies can create barriers to access for patients who could benefit from PT. Efforts are needed to develop evidence-based guidelines for PT reimbursement and to ensure that patients have access to the treatment they need.
Many thanks to our sponsor Esdebe who helped us prepare this research report.
7. Future Directions and Research
Proton therapy is a rapidly evolving field with ongoing research focused on improving treatment techniques, expanding clinical applications, and reducing costs. Emerging research areas include adaptive PT, FLASH radiotherapy, and the use of PT in combination with immunotherapy.
7.1. Adaptive Proton Therapy
Adaptive PT holds great promise for improving treatment outcomes by accounting for changes in tumor size, shape, or position during treatment. Future research should focus on developing more sophisticated imaging techniques and treatment planning algorithms to enable real-time adaptation of the PT plan.
7.2. FLASH Radiotherapy
FLASH radiotherapy has the potential to revolutionize cancer treatment by selectively sparing normal tissues from radiation damage. Further preclinical and clinical studies are needed to optimize FLASH radiotherapy techniques and to identify the patient populations that are most likely to benefit from this approach.
7.3. Proton Therapy and Immunotherapy
Combining PT with immunotherapy may enhance the anti-tumor immune response and improve treatment outcomes. Preclinical studies have shown that radiation can stimulate the release of tumor-associated antigens, making the tumor more susceptible to immunotherapy. Clinical trials are underway to evaluate the safety and efficacy of combining PT with various immunotherapy agents.
7.4 In-Vivo Dosimetry
One of the most critical areas of current PT research and future development lies in the ability to accurately measure the radiation dose delivered in-vivo. Current methods rely on pre-treatment planning and simulation, which are subject to uncertainties due to patient movement, anatomical changes, and variations in tissue density. Developing real-time, in-vivo dosimetry methods would allow for immediate verification of the delivered dose, enabling more accurate treatment and adaptive planning. Techniques such as prompt gamma imaging and positron emission tomography (PET) are being explored for this purpose [12]. However, significant challenges remain in terms of sensitivity, resolution, and practicality for routine clinical use.
7.5. Artificial Intelligence and Machine Learning
AI and machine learning (ML) are increasingly being explored to automate and optimize various aspects of PT treatment planning and delivery [13]. These include automatic target volume delineation, dose optimization, and treatment plan quality assurance. AI/ML can also be used to predict treatment outcomes and identify patients who are most likely to benefit from PT. Furthermore, AI/ML can assist in improving treatment plan robustness, mitigating the impact of uncertainties, and facilitating adaptive planning workflows. The application of AI/ML in PT has the potential to significantly improve treatment efficiency, accuracy, and personalization, ultimately leading to better clinical outcomes.
Many thanks to our sponsor Esdebe who helped us prepare this research report.
8. Conclusion
Proton therapy represents a significant advancement in radiation oncology, offering the potential for improved dose conformity and reduced off-target radiation exposure compared to conventional photon therapy. PT has shown promise in the treatment of a wide range of cancers, particularly in pediatric patients and tumors located near critical structures. Technological advancements such as PBS, IGPT, and adaptive PT have further enhanced the therapeutic potential of PT. However, PT remains more expensive and less accessible than photon therapy. Ongoing research is focused on improving treatment techniques, expanding clinical applications, and reducing costs. Future directions include the development of adaptive PT, FLASH radiotherapy, and the use of PT in combination with immunotherapy. The application of AI/ML in PT also holds great promise for improving treatment efficiency, accuracy, and personalization. As PT technology continues to evolve and become more widely available, it is poised to play an increasingly important role in cancer treatment.
Many thanks to our sponsor Esdebe who helped us prepare this research report.
References
[1] Eaton, B. R., MacDonald, S. M., & Tarbell, N. J. (2016). Proton radiation for pediatric malignancies: state of the art. International Journal of Radiation Oncology, Biology, Physics, 94(5), 877-887.
[2] Weber, D. C., Lomax, A. J., Cella, L., Miralbell, R., & Rutz, H. P. (2003). Spot-scanning proton therapy for chordomas and chondrosarcomas of the skull base: first clinical experience. International Journal of Radiation Oncology, Biology, Physics, 55(5), 1396-1408.
[3] MacDonald, S. M., et al. (2018). Proton radiotherapy for childhood medulloblastoma: comparing outcomes with photons. International Journal of Particle Therapy, 5(1), 20-29.
[4] Bush, D. A., Slater, J. D., Jiang, L., Shinohara, E. T., Booth, J., & Wang, P. C. (2009). Fractionated proton radiotherapy for chordomas and chondrosarcomas of the skull base: clinical outcomes for 21 patients. International Journal of Radiation Oncology, Biology, Physics, 75(3), 604-611.
[5] Combs, S. E., Volkmer, B., Dittmar, J. O., Schulz-Ertner, D., Welzel, G., Debus, J., & Haberer, T. (2009). High precision radiotherapy using proton beams: set-up and first clinical experiences at the Heidelberg Ion Therapy Center (HIT). Strahlentherapie und Onkologie, 185(8), 509-513.
[6] Holliday, E. B., Frank, S. J., Garden, A. S., Rosenthal, D. I., Barker, J. L., Gillin, M. T., … & Ang, K. K. (2014). Intensity-modulated proton therapy reduces treatment-related morbidity and improves quality of life among patients with oropharyngeal cancer: first results of a prospective trial. International Journal of Radiation Oncology, Biology, Physics, 89(1), 17-26.
[7] Blanchard, P., Garden, A. S., Gunn, G. B., Kies, M. S., Morrison, W. H., Schwartz, D. L., … & Ang, K. K. (2015). Dosimetric and clinical comparison of intensity-modulated photon therapy and passively scattered proton therapy for oropharyngeal cancer. Radiotherapy and Oncology, 115(3), 374-380.
[8] Sejpal, S. V., Komaki, R., Tsao, A. S., Chang, J. Y., Liao, Z., Lee, J. J., … & Cox, J. D. (2012). Early findings on toxicity of proton beam therapy with concurrent chemotherapy for non-small cell lung cancer. Cancer, 118(10), 2753-2760.
[9] Hoppe, B. S., Huh, S. H., Flampouri, S., Lynch, J., Montejo, J. L., Henderson, R., … & Mendenhall, N. P. (2014). Feasibility and early outcomes of proton beam therapy for locally advanced non-small cell lung cancer. American Journal of Clinical Oncology, 37(3), 255-261.
[10] Bryant, C. L., Smith, T. L., Henderson, R. H., Deville, C., Mendenhall, N. P., Nichols, R. C., & Hoppe, B. S. (2012). Biochemical and quality-of-life outcomes after proton beam therapy for prostate cancer. International Journal of Radiation Oncology, Biology, Physics, 84(2), e217-e222.
[11] Gray, P. J., Zietman, A. L., Choueiri, T. K., Sandler, H. M., Shipley, W. U., & Sweeney, C. J. (2011). Comparative effectiveness of proton therapy versus intensity-modulated radiation therapy for prostate cancer: a matched-pair analysis. Journal of Clinical Oncology, 29(12), 1607-1613.
[12] Polf, J. C., Peterson, S. W., & Taddei, P. J. (2018). A review of prompt gamma imaging in proton therapy. Physics in Medicine & Biology, 63(21), 21TR01.
[13] Mahmood, U., et al. (2021). Artificial intelligence in radiation oncology: current applications and future directions. Radiation Oncology, 16(1), 1-16.
Given the increasing number of proton therapy facilities, how is the industry addressing the challenge of ensuring a sufficiently trained workforce to operate and maintain these complex systems effectively?
That’s a great point! Workforce development is key. Many facilities are partnering with universities and professional organizations to create specialized training programs. Mentorships and apprenticeships within existing centers are also crucial for hands-on experience. It’s a multi-faceted approach to meet this growing demand.
Editor: MedTechNews.Uk
Thank you to our Sponsor Esdebe
Fascinating report! Given the complex interplay of factors in proton therapy, are we any closer to truly personalized treatment plans that consider not just the tumour, but also the individual patient’s genetic makeup and lifestyle? Perhaps one day, AI will tailor the beam *and* suggest the perfect post-therapy smoothie.