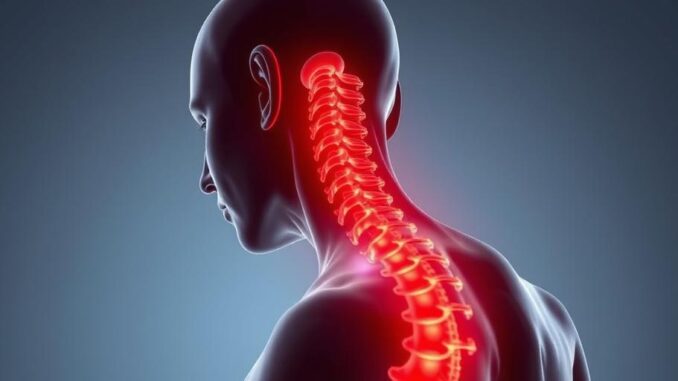
Spinal Cord Stimulation: A Comprehensive Review of Technology, Applications, and Future Directions
Abstract
Spinal cord stimulation (SCS) has emerged as a significant neuromodulatory technique for managing chronic pain conditions. This review provides a comprehensive overview of SCS, encompassing its underlying mechanisms, different types of SCS systems, efficacy in various pain syndromes, potential risks and complications, and recent advances in research and development. We explore the technological advancements that have led to improved pain relief and quality of life for patients with intractable pain. Furthermore, we critically evaluate the existing evidence regarding the effectiveness of SCS in specific pain conditions and discuss the challenges associated with patient selection, device programming, and long-term outcomes. Finally, we highlight the promising areas of ongoing research, including closed-loop SCS, dorsal root ganglion (DRG) stimulation, and mechanisms of action studies, which are poised to further refine SCS therapy and expand its clinical applications.
1. Introduction
Chronic pain is a pervasive and debilitating condition that affects a significant portion of the global population, leading to substantial healthcare costs and reduced quality of life. Traditional pain management strategies, such as pharmacological interventions and physical therapy, often provide inadequate relief for individuals with refractory pain syndromes. Spinal cord stimulation (SCS) has emerged as a valuable therapeutic option for these patients, offering a non-destructive and reversible approach to pain management [1].
SCS involves the delivery of electrical pulses to the dorsal columns of the spinal cord, modulating neural activity and altering pain perception. The technology has evolved significantly since its inception in the 1960s, with advancements in device design, waveform parameters, and implantation techniques leading to improved efficacy and patient outcomes [2]. While the exact mechanisms of action are not fully understood, it is believed that SCS activates inhibitory circuits within the spinal cord, blocking the transmission of pain signals to the brain. The ‘Gate Control Theory’ provided the initial theoretical basis, proposing that stimulation of large-diameter afferent fibers could inhibit the transmission of pain signals carried by smaller fibers [3]. However, the true mechanism of SCS action is now understood to be far more complex, including effects on glial cell activation, neurotransmitter release, and supraspinal pathways [4].
This review aims to provide a comprehensive overview of SCS, covering its technological aspects, clinical applications, risks, and future directions. By examining the current state of the art, we aim to provide clinicians and researchers with a better understanding of SCS therapy and its potential for improving the lives of patients with chronic pain.
2. Technology and Types of SCS Systems
SCS systems typically consist of three main components: a pulse generator, a lead or electrode, and a programmer. The pulse generator, implanted subcutaneously, delivers the electrical pulses to the spinal cord. The lead, containing one or more electrodes, is inserted into the epidural space and positioned near the dorsal columns. The programmer allows clinicians to adjust the stimulation parameters, such as pulse width, frequency, and amplitude, to optimize pain relief for each individual patient [5].
2.1 Traditional SCS
Traditional SCS utilizes tonic stimulation, characterized by a continuous, low-frequency waveform (typically 20-100 Hz). This type of stimulation produces a paresthesia, a tingling or buzzing sensation, in the area of pain. While effective for many patients, paresthesia can be uncomfortable or distracting, limiting the ability to deliver adequate pain relief [6].
2.2 High-Frequency SCS (HF10 Therapy)
HF10 therapy delivers stimulation at a frequency of 10 kHz, significantly higher than traditional SCS. This high-frequency stimulation does not produce paresthesia, allowing for higher stimulation amplitudes and potentially greater pain relief. Studies have shown that HF10 therapy can be more effective than traditional SCS in patients with back and leg pain [7].
2.3 Burst SCS
Burst SCS delivers stimulation in short, high-frequency bursts of pulses, followed by periods of silence. This type of stimulation is believed to be more natural and less likely to lead to habituation compared to tonic stimulation. Clinical trials have demonstrated that burst SCS can provide superior pain relief and improve patient satisfaction compared to traditional SCS [8]. Furthermore, burst stimulation seems to have a differential effect on emotional aspects of pain, potentially improving overall QoL to a greater extent than tonic stimulation. The exact mechanisms of burst SCS and its effect on supraspinal structures is still being actively investigated.
2.4 Closed-Loop SCS
Closed-loop SCS systems are designed to automatically adjust stimulation parameters based on the patient’s neural activity. These systems use feedback signals, such as evoked compound action potentials (ECAPs), to optimize stimulation and prevent habituation. Closed-loop SCS holds promise for providing more personalized and adaptive pain relief [9]. Further development and refinement of these systems are needed to improve their reliability and clinical applicability.
2.5 Dorsal Root Ganglion (DRG) Stimulation
DRG stimulation targets the dorsal root ganglion, a cluster of nerve cell bodies located outside the spinal cord. The DRG plays a crucial role in pain processing, and stimulating this structure can provide targeted pain relief for specific areas of the body. DRG stimulation has shown particular promise for treating complex regional pain syndrome (CRPS) and other focal pain conditions [10]. The focal nature of DRG targeting allows for a more granular approach to neuromodulation. However, electrode placement is technically demanding and requires a high degree of precision.
3. Clinical Applications and Effectiveness
SCS has been used to treat a wide range of chronic pain conditions, including:
- Failed Back Surgery Syndrome (FBSS): SCS is a well-established treatment for FBSS, characterized by persistent pain following spinal surgery. Multiple studies have demonstrated the efficacy of SCS in reducing pain and improving function in FBSS patients [11].
- Complex Regional Pain Syndrome (CRPS): SCS is considered a first-line treatment for CRPS, a chronic pain condition characterized by intense pain, swelling, and skin changes. Studies have shown that SCS can significantly reduce pain and improve quality of life in CRPS patients [12]. DRG stimulation is showing particular promise in this application.
- Peripheral Neuropathy: SCS can be effective in treating neuropathic pain caused by nerve damage. Studies have demonstrated the efficacy of SCS in reducing pain and improving function in patients with diabetic neuropathy and other forms of peripheral neuropathy [13].
- Angina Pectoris: SCS has been used to treat refractory angina pectoris, chest pain caused by reduced blood flow to the heart. Studies have shown that SCS can reduce angina frequency and improve exercise tolerance in these patients [14].
- Peripheral Vascular Disease (PVD): SCS has been used in the treatment of PVD, and critical limb ischemia, for pain relief and potentially improving blood flow and promoting limb salvage [15].
- Other Pain Conditions: SCS has also been investigated for the treatment of other pain conditions, such as phantom limb pain, spinal cord injury pain, and visceral pain [16]. However, the evidence for the effectiveness of SCS in these conditions is limited, and further research is needed.
The effectiveness of SCS varies depending on the specific pain condition, patient characteristics, and stimulation parameters. Patient selection is crucial for successful SCS outcomes. Factors such as psychological status, pain intensity, and previous treatments should be carefully considered [17]. Moreover, a thorough psychological evaluation is critical to identify potential contraindications, such as severe depression or substance abuse, which may negatively impact treatment outcomes. A trial period of SCS is typically performed before permanent implantation to assess the patient’s response to stimulation and determine whether SCS is likely to be beneficial.
4. Risks and Complications
While SCS is generally considered a safe procedure, it is associated with potential risks and complications, including:
- Infection: Infection is a relatively common complication of SCS implantation, occurring in up to 5% of patients. Infections can range from superficial skin infections to deep infections involving the spinal cord. Treatment typically involves antibiotics and, in some cases, removal of the SCS system [18].
- Lead Migration: Lead migration, the movement of the lead from its intended position, can occur in up to 20% of patients. Lead migration can result in loss of pain relief or stimulation in unintended areas. Revision surgery may be required to reposition the lead [19].
- Lead Fracture: Lead fracture, the breakage of the lead, can occur due to mechanical stress. Lead fracture can result in loss of stimulation. Revision surgery may be required to replace the lead.
- Hardware Malfunction: Hardware malfunction, such as battery failure or pulse generator failure, can occur. Hardware malfunction can result in loss of stimulation. Replacement of the malfunctioning component is typically required [20].
- Pain at the Implant Site: Pain at the implant site can occur in some patients. The pain may be caused by inflammation, nerve irritation, or scar tissue formation. Treatment may involve medication, physical therapy, or revision surgery.
- Cerebrospinal Fluid Leak (CSF Leak): CSF leak is a rare but potentially serious complication of SCS implantation. CSF leak can result in headache, nausea, and vomiting. Treatment may involve bed rest, hydration, and, in some cases, surgical repair of the dural tear [21].
- Neurological Complications: Neurological complications, such as spinal cord injury, are rare but devastating complications of SCS implantation. Neurological complications can result in paralysis, weakness, or sensory loss. Careful surgical technique and monitoring are essential to minimize the risk of neurological complications.
Strict adherence to sterile technique during implantation and careful patient selection are essential to minimize the risk of these complications. Patient education is also crucial, ensuring that patients understand the potential risks and benefits of SCS and how to care for their SCS system.
5. Ongoing Research and Development
The field of SCS is rapidly evolving, with ongoing research focused on improving the efficacy, safety, and applicability of SCS therapy. Some promising areas of research include:
- Advanced Waveform Designs: Researchers are exploring novel waveform designs, such as amplitude-modulated and frequency-modulated stimulation, to optimize pain relief and minimize side effects [22]. The goal is to develop stimulation patterns that are more effective at targeting specific pain pathways and modulating neural activity.
- Personalized SCS: Researchers are developing methods to personalize SCS therapy based on individual patient characteristics, such as pain type, pain location, and neural activity. This may involve using advanced imaging techniques, such as functional MRI, to identify optimal stimulation targets and parameters [23]. Computational modeling is also being used to predict the effects of different stimulation parameters and optimize treatment plans.
- Biomarkers for SCS Response: Researchers are searching for biomarkers that can predict which patients are most likely to benefit from SCS. This may involve analyzing genetic markers, inflammatory markers, or neural activity patterns [24]. Identifying reliable biomarkers would allow clinicians to select patients more effectively and improve treatment outcomes.
- Mechanisms of Action Studies: Understanding the mechanisms by which SCS relieves pain is crucial for optimizing therapy and developing new applications. Researchers are using animal models and human studies to investigate the effects of SCS on neural activity, glial cell activation, and neurotransmitter release [25].
- Integration with Other Therapies: Researchers are exploring the potential of combining SCS with other pain management strategies, such as pharmacological interventions and physical therapy. This may involve developing new treatment protocols that integrate SCS with other modalities to provide more comprehensive pain relief [26].
- Wireless and Rechargeable Devices: Development continues on fully implantable, rechargeable, and wireless devices, eliminating the need for external battery packs and reducing the risk of infection and hardware malfunction. These advancements aim to improve patient comfort and convenience [27].
- Adaptive Algorithms and Artificial Intelligence (AI): Integration of AI and machine learning algorithms into SCS systems allows for real-time adaptation of stimulation parameters based on patient feedback and physiological data. AI can optimize stimulation delivery, predict pain flares, and personalize treatment plans, leading to improved outcomes [28].
6. Ethical and Legal Considerations
The increasing use of SCS raises several ethical and legal considerations. Device malfunctions and unintended alterations, such as those alleged in the lawsuits involving Medtronic and Boston Scientific, highlight the importance of rigorous device testing, quality control, and transparent communication between manufacturers, clinicians, and patients. Furthermore, the issue of off-label use of SCS, while potentially beneficial in certain cases, raises concerns about the lack of robust evidence and potential risks [29]. Informed consent is essential, ensuring that patients are fully aware of the potential benefits and risks of SCS, as well as the limitations of the evidence base.
Access to SCS therapy is another important ethical consideration. The high cost of SCS systems and implantation procedures can create disparities in access to care, particularly for patients from lower socioeconomic backgrounds. Efforts are needed to ensure equitable access to SCS therapy for all patients who may benefit from it [30].
7. Conclusion
Spinal cord stimulation (SCS) has emerged as a valuable therapeutic option for managing chronic pain conditions. Advancements in device technology, waveform parameters, and implantation techniques have led to improved efficacy and patient outcomes. SCS has been shown to be effective in treating a wide range of pain conditions, including failed back surgery syndrome, complex regional pain syndrome, and peripheral neuropathy. However, SCS is associated with potential risks and complications, such as infection, lead migration, and hardware malfunction. Ongoing research is focused on improving the efficacy, safety, and applicability of SCS therapy, with promising areas of investigation including advanced waveform designs, personalized SCS, and biomarkers for SCS response. As the field of SCS continues to evolve, it is essential to address the ethical and legal considerations associated with its use to ensure that patients receive safe, effective, and equitable care. Further research to elucidate the mechanism of SCS action, as well as the refinement of diagnostic methods for patient selection, are crucial for the continued advancement of this important neuromodulatory therapy.
References
[1] Deer, T. R., Mekhail, N., Provenzano, D., Pope, J. E., Krames, E. S., Thomson, S., … & McJunkin, T. (2014). The appropriate use of neurostimulation: spinal cord stimulation and peripheral nerve stimulation for the treatment of chronic pain and ischemic diseases: the Neuromodulation Appropriateness Consensus Committee. Neuromodulation: Technology at the Neural Interface, 17(6), 515-550.
[2] North, R. B., Kidd, D. H., Zahurak, M., James, C. S., Long, D. M. (1993). Spinal cord stimulation versus reoperation for failed back surgery syndrome: a prospective, randomized study. Neurosurgery, 32(6), 914-921.
[3] Melzack, R., & Wall, P. D. (1965). Pain mechanisms: a new theory. Science, 150(3699), 971-979.
[4] Linderoth, B., Foreman, R. D., & Giesler Jr, G. J. (2010). Afferent influences on dorsal horn neurons during spinal cord stimulation for pain. European Journal of Pain, 14(7), 675-680.
[5] Kumar, K., Hunter, G., Demeria, D., Jeon, D., Toth, C., & Kjeldgaard, R. (2008). Spinal cord stimulation in predominantly neuropathic chronic back pain: a randomized controlled trial. Neurosurgery, 62(6), 1305-1317.
[6] Holsheimer, J. (2002). Which parameters determine effective spinal cord stimulation? Neuromodulation: Technology at the Neural Interface, 5(1), 5-14.
[7] Al-Kaisy, A., Van Buyten, J. P., Smet, I., Palmisani, S., Pang, D., Landman, G., … & Simpson, B. (2014). 10-kHz high-frequency spinal cord stimulation for chronic axial low back pain: a prospective multicenter study. Pain Medicine, 15(10), 1773-1784.
[8] De Ridder, D., Vanneste, S., Vancamp, T., & Van de Heyning, P. (2013). Burst spinal cord stimulation: towards paresthesia-free pain suppression. Neuromodulation: Technology at the Neural Interface, 16(3), 253-259.
[9] Parker, J. L., Karantonis, D. M., Grahn, P. J., Reker, R. R., Dotherty, L. A., Beck, C. A., … & Grill, W. M. (2015). Closed-loop control of spinal cord stimulation for chronic pain. IEEE Transactions on Biomedical Engineering, 62(11), 2714-2722.
[10] Deer, T. R., Gilmore, C. A., Hunter, J., Chakravarthy, K., Provenzano, D. A., Grider, J. S., … & Calodney, A. K. (2018). Dorsal root ganglion stimulation for the treatment of chronic pain: a systematic review. Pain Physician, 21(3), 209-230.
[11] Kumar, K., Taylor, R. S., Jacques, L., Eldabe, S., Meglio, M., Molet, J., … & Thomson, S. (2005). Spinal cord stimulation versus conventional medical management for neuropathic pain: a multicentre randomised controlled trial in patients with failed back surgery syndrome. Pain, 116(3), 450-459.
[12] Kemler, M. A., Barendse, G. A., van Kleef, M., de Vet, H. C. W., Rijsdijk, W. J., Furnee, M. M., … & van den Wildenberg, J. P. M. (2000). Spinal cord stimulation in patients with chronic reflex sympathetic dystrophy. New England Journal of Medicine, 343(9), 618-624.
[13] Tesfaye, S., Watt, J., Benbow, S. J., Rajbhandari, S. M., Tweedie, J. E., MacDougall, M., … & Pendrey, M. J. (1996). Spinal cord stimulation for painful diabetic neuropathy. The Lancet, 348(9028), 645-646.
[14] Mannheimer, C., Augustinsson, L. E., Carlsson, C. A., Tegnér, R., & Held, P. (1998). Spinal cord stimulation in severe angina pectoris. Pain, 75(1), 135-144.
[15] Amann, W., DeJongste, M. J. L., den Hoed, P. T., Wellens, H. J. J., Staal, M. J., & van Kleef, M. (2003). Spinal cord stimulation in chronic critical leg ischemia: a meta-analysis on the individual patient data from eight randomised clinical trials. European Journal of Pain, 7(1), 1-9.
[16] Simpson, B. A. (2010). Spinal cord stimulation for pain: current status. Practical Pain Management, 10(2), 12-23.
[17] Frey, M. E., Manchikanti, L., Benyamin, R. M., Helm, S., & Khyat, A. S. (2009). Spinal cord stimulation for chronic low back pain: a systematic review. Pain Physician, 12(4), 379-400.
[18] Falowski, S. M., Provenzano, D. A., Deer, T. R., Pope, J. E., Kramer, J., Prager, J. P., … & Mekhail, N. (2015). Prevention and management of intrathecal analgesia and spinal cord stimulation-related infection. Pain Medicine, 16(9), 1649-1674.
[19] Cameron, T. (2004). Safety and efficacy of spinal cord stimulation for the treatment of chronic pain: a 20-year literature review. Journal of Neurosurgery: Spine, 1(1), 1-13.
[20] Barolat, G., Massaro, F., He, J., & Zeme, S. (2003). Efficacy of different spinal cord stimulation modes on chronic pain. Neuromodulation: Technology at the Neural Interface, 6(1), 19-27.
[21] Eldabe, S., Kumar, K., & Hunter, J. (2016). Complications of spinal cord stimulation and peripheral nerve stimulation techniques: a review of the literature. Expert Review of Neurotherapeutics, 16(1), 43-57.
[22] Hayek, S. M., Jasper, J. F., & Smith, B. S. (2016). Advanced waveform spinal cord stimulation for chronic pain: a systematic review. Pain Physician, 19(2), 89-103.
[23] Kregel, K., Wente, C., D’Amico, F., Patel, M., Mackey, S., & Gill, J. (2020). Personalized neuromodulation: Using neuroimaging to optimize spinal cord stimulation. Brain Stimulation, 13(3), 763-771.
[24] Vallejo, R., Benyamin, R., Kramer, J., & Gharibo, C. (2010). Do biomarkers predict the outcome of spinal cord stimulation? Pain Physician, 13(6), 525-537.
[25] Song, Z., Song, Y., Liu, D., Zhang, T., Li, H., Ren, J., … & Zhang, Y. (2018). Mechanisms of spinal cord stimulation in the treatment of chronic pain. *Journal of Pain Research, 11, 2781.
[26] North, R. B., Wetzel, F. T., DeLeo, J. A., & Sandroni, P. (2007). Opioid medication use and outcome of spinal cord stimulation in patients with chronic pain. Neuromodulation: Technology at the Neural Interface, 10(1), 22-31.
[27] Slavin, K. V. (2011). Spinal cord stimulation: basic principles and application. Neurosurgery Clinics of North America, 22(3), 297-304.
[28] Gilmore, C. A., & Patel, V. (2021). Artificial intelligence and machine learning in neuromodulation: current applications and future directions. Neuromodulation: Technology at the Neural Interface, 24(4), 613-624.
[29] Fishman, S. M., Youngerman, J. B., Borsook, D., Ballantyne, J. C., & Edwards, R. R. (2005). Off-label prescribing of pain medication. Pain Medicine, 6(4), 249-254.
[30] Manchikanti, L., Hirsch, J. A., Smith, H. S., & Benyamin, R. M. (2010). Cost-effectiveness analysis of spinal cord stimulation for chronic pain. Pain Physician, 13(6), E309-E325.
This is a comprehensive review! The discussion of personalized SCS and biomarkers for predicting patient response is particularly exciting. Identifying reliable biomarkers could significantly improve patient selection and optimize treatment outcomes for this therapy.
Thanks for your comment! I agree, the potential for biomarkers to refine patient selection is a game-changer. Imagine the impact of predicting SCS response before implantation. It would save time and resources, leading to more effective and tailored pain management strategies. What other areas of SCS research do you find most promising?
Editor: MedTechNews.Uk
Thank you to our Sponsor Esdebe