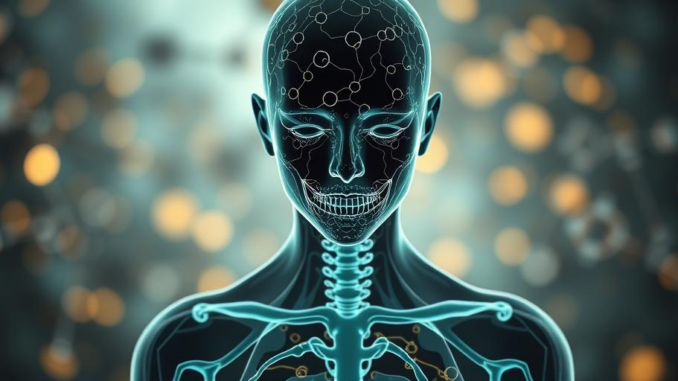
Abstract
Stem cell research stands at the forefront of regenerative medicine, offering potential cures and treatments for a vast array of diseases. This report provides a comprehensive overview of stem cell biology, encompassing various stem cell types (embryonic, adult, and induced pluripotent stem cells), their differentiation capabilities, and the underlying mechanisms governing their behavior. We delve into the applications of stem cell therapy in treating diseases such as type 1 diabetes, neurological disorders, cardiovascular diseases, and autoimmune conditions, highlighting both pre-clinical and clinical trial progress. Ethical considerations surrounding stem cell research, particularly concerning embryonic stem cells, are critically examined. Furthermore, we address the inherent challenges and limitations associated with stem cell therapies, including the risk of tumorigenicity, immune rejection, and the complexity of achieving precise tissue regeneration. Finally, we explore emerging technologies and future directions in stem cell research, focusing on strategies to enhance safety, efficacy, and personalized medicine approaches, ultimately shaping the trajectory of regenerative medicine.
Many thanks to our sponsor Esdebe who helped us prepare this research report.
1. Introduction
Stem cell biology has revolutionized our understanding of development, disease, and potential therapeutic interventions. Stem cells, characterized by their self-renewal capacity and pluripotency (or multipotency), hold immense promise for regenerative medicine. These remarkable cells can differentiate into various cell types, offering a potential source for repairing damaged tissues and organs. The discovery of stem cells and the ability to manipulate them have opened new avenues for treating a wide range of diseases, including degenerative conditions, genetic disorders, and injuries. The field has evolved rapidly, with advancements in stem cell isolation, culture, differentiation, and delivery methods. However, the application of stem cell therapies is not without its challenges, including ethical concerns, technical hurdles, and safety considerations. This review aims to provide a comprehensive overview of stem cell biology, their therapeutic applications, and the challenges that need to be addressed to realize their full potential.
The initial promise of stem cell therapy was largely tied to embryonic stem cells (ESCs), derived from the inner cell mass of blastocysts. However, the ethical considerations associated with ESC derivation spurred the development of alternative approaches. The groundbreaking discovery of induced pluripotent stem cells (iPSCs) by Yamanaka and colleagues [1] provided a powerful tool to generate pluripotent stem cells from adult somatic cells. This innovation circumvented the ethical concerns surrounding ESCs and opened new possibilities for personalized medicine. iPSCs can be generated from a patient’s own cells, eliminating the risk of immune rejection. Adult stem cells, residing in various tissues, represent another important source of stem cells for regenerative medicine. While adult stem cells are generally more restricted in their differentiation potential compared to ESCs and iPSCs, they offer the advantage of being readily available and pose fewer ethical concerns.
The central dogma of stem cell therapy revolves around harnessing the inherent regenerative capacity of these cells to repair or replace damaged tissues. This can be achieved through various strategies, including cell transplantation, stimulation of endogenous stem cell activity, and delivery of stem cell-derived factors. However, the complexity of tissue regeneration necessitates a thorough understanding of the underlying mechanisms governing stem cell behavior, including their interactions with the microenvironment and their response to various signaling cues.
Many thanks to our sponsor Esdebe who helped us prepare this research report.
2. Types of Stem Cells
2.1 Embryonic Stem Cells (ESCs)
ESCs are derived from the inner cell mass of blastocysts, the pre-implantation embryo at the blastocyst stage. They are characterized by their pluripotency, meaning they can differentiate into any cell type in the body, including germ cells [2]. ESCs are maintained in a pluripotent state by a complex network of transcription factors, including Oct4, Sox2, and Nanog [3]. These transcription factors regulate the expression of genes involved in self-renewal and pluripotency, while repressing genes involved in differentiation. ESCs can be cultured indefinitely in vitro, providing a continuous source of pluripotent cells. However, the use of ESCs raises ethical concerns due to the destruction of embryos during their derivation. Furthermore, ESC-derived therapies carry the risk of teratoma formation, as the undifferentiated cells can form tumors. Careful differentiation and purification of ESC-derived cells are crucial to mitigate this risk.
2.2 Adult Stem Cells
Adult stem cells, also known as somatic stem cells, reside in various tissues and organs throughout the body. They are responsible for tissue maintenance and repair [4]. Unlike ESCs, adult stem cells are generally multipotent, meaning they can differentiate into a limited number of cell types, typically within the tissue of origin. Examples of adult stem cells include hematopoietic stem cells (HSCs) in the bone marrow, mesenchymal stem cells (MSCs) in various tissues, and neural stem cells (NSCs) in the brain. HSCs give rise to all blood cell types, while MSCs can differentiate into bone, cartilage, fat, and muscle cells. NSCs can differentiate into neurons, astrocytes, and oligodendrocytes. Adult stem cells offer the advantage of being readily available and pose fewer ethical concerns compared to ESCs. However, they are often present in small numbers and are more difficult to culture and expand in vitro. Furthermore, their differentiation potential is more restricted compared to ESCs.
2.3 Induced Pluripotent Stem Cells (iPSCs)
iPSCs are generated from adult somatic cells through a process called reprogramming. Reprogramming involves introducing a set of transcription factors, typically Oct4, Sox2, Klf4, and c-Myc (OSKM), into adult cells [1]. These transcription factors induce epigenetic changes that revert the cells to a pluripotent state, similar to ESCs. iPSCs possess similar characteristics to ESCs, including pluripotency and self-renewal capacity. However, iPSCs are not derived from embryos, circumventing the ethical concerns associated with ESCs. Furthermore, iPSCs can be generated from a patient’s own cells, eliminating the risk of immune rejection. iPSCs have revolutionized stem cell research and offer immense potential for personalized medicine. However, the reprogramming process can be inefficient and can lead to epigenetic abnormalities and genetic mutations. Furthermore, iPSC-derived therapies carry the risk of teratoma formation, similar to ESCs. Careful characterization and quality control of iPSCs are crucial to ensure their safety and efficacy.
Many thanks to our sponsor Esdebe who helped us prepare this research report.
3. Applications of Stem Cell Therapy
3.1 Type 1 Diabetes
Type 1 diabetes (T1D) is an autoimmune disease characterized by the destruction of insulin-producing beta cells in the pancreas [5]. Stem cell therapy holds promise for T1D by replacing the damaged beta cells with functional cells. Several approaches are being explored, including transplantation of pancreatic progenitor cells derived from ESCs or iPSCs, and induction of beta cell regeneration from endogenous stem cells. Recent clinical trials have shown promising results using encapsulated stem cell-derived beta cells to protect them from immune attack, resulting in insulin independence in some patients [6]. However, long-term efficacy and safety need to be further evaluated.
The main challenge is generating fully functional, glucose-responsive beta cells in vitro. Researchers are employing sophisticated differentiation protocols, mimicking the developmental process of the pancreas, to generate beta cells with improved functionality. Immunosuppression is another critical aspect of stem cell therapy for T1D, as the transplanted cells are susceptible to immune rejection. Encapsulation strategies and genetic engineering approaches are being developed to protect the transplanted cells from immune attack. The development of personalized stem cell therapies using iPSCs offers the potential to eliminate the need for immunosuppression.
3.2 Neurological Disorders
Stem cell therapy holds promise for treating various neurological disorders, including Parkinson’s disease, Alzheimer’s disease, spinal cord injury, and stroke [7]. In Parkinson’s disease, dopamine-producing neurons are lost, leading to motor dysfunction. Stem cell therapy aims to replace the lost dopamine neurons with functional cells. Clinical trials using ESC-derived dopamine neurons have shown promising results, with some patients experiencing significant improvements in motor function [8]. However, long-term efficacy and safety need to be further evaluated.
In spinal cord injury, stem cell therapy aims to promote axonal regeneration and functional recovery. Several approaches are being explored, including transplantation of NSCs, MSCs, and olfactory ensheathing cells. While some clinical trials have shown modest improvements in motor function and sensory perception, the results have been variable. The complex nature of spinal cord injury and the challenges of promoting axonal regeneration pose significant hurdles.
Stem cell therapy for Alzheimer’s disease aims to replace lost neurons and protect remaining neurons from further damage. Transplantation of NSCs and MSCs has shown some promise in pre-clinical studies, but clinical trials are still in early stages. The development of effective stem cell therapies for neurological disorders requires a thorough understanding of the underlying disease mechanisms and the development of strategies to promote neuronal survival, differentiation, and integration into the existing neural circuitry.
3.3 Cardiovascular Diseases
Stem cell therapy has emerged as a potential treatment for cardiovascular diseases, including heart failure, myocardial infarction, and peripheral artery disease [9]. In heart failure, the heart muscle is damaged and weakened, leading to impaired cardiac function. Stem cell therapy aims to regenerate damaged heart muscle and improve cardiac function. Several approaches are being explored, including transplantation of MSCs, cardiac stem cells, and iPSC-derived cardiomyocytes. Clinical trials have shown some improvements in cardiac function and exercise capacity, but the results have been variable. The challenges include poor engraftment of transplanted cells, limited differentiation into functional cardiomyocytes, and the complex nature of the heart microenvironment.
In myocardial infarction, the heart muscle is damaged due to a lack of blood supply. Stem cell therapy aims to regenerate damaged heart muscle and prevent further damage. Transplantation of MSCs and cardiac stem cells has shown some promise in pre-clinical studies and clinical trials. The development of strategies to improve cell engraftment, survival, and differentiation is crucial for the success of stem cell therapy for cardiovascular diseases. Furthermore, the use of biomaterials and tissue engineering approaches can enhance the regenerative potential of stem cell therapies.
3.4 Autoimmune Conditions
Stem cell therapy is being investigated as a potential treatment for various autoimmune conditions, including multiple sclerosis, rheumatoid arthritis, and Crohn’s disease [10]. In autoimmune diseases, the immune system attacks the body’s own tissues, leading to chronic inflammation and tissue damage. Stem cell therapy aims to reset the immune system and promote immune tolerance. Several approaches are being explored, including hematopoietic stem cell transplantation (HSCT) and transplantation of MSCs. HSCT involves ablating the patient’s immune system with chemotherapy and then transplanting autologous or allogeneic HSCs. This can lead to long-term remission in some patients, but it also carries the risk of serious complications, including infections and graft-versus-host disease. MSCs have immunomodulatory properties and can suppress the immune response. Transplantation of MSCs has shown some promise in clinical trials for various autoimmune conditions, but the results have been variable. The mechanisms by which MSCs exert their immunomodulatory effects are not fully understood. Further research is needed to optimize MSC-based therapies for autoimmune diseases.
Many thanks to our sponsor Esdebe who helped us prepare this research report.
4. Ethical Considerations
The ethical considerations surrounding stem cell research, particularly concerning ESCs, have been a subject of intense debate. The destruction of embryos during ESC derivation raises concerns about the moral status of the embryo and the ethical permissibility of using embryos for research purposes [11]. Proponents of ESC research argue that the potential benefits of ESC therapies outweigh the ethical concerns, particularly for diseases that lack effective treatments. They argue that embryos created for in vitro fertilization (IVF) that are no longer needed for reproductive purposes would otherwise be discarded and that using them for research is a morally justifiable way to maximize their potential benefit.
Opponents of ESC research argue that the embryo has a right to life from conception and that destroying it is morally wrong. They advocate for alternative approaches, such as adult stem cell research and iPSC technology, which do not involve the destruction of embryos. The development of iPSC technology has significantly reduced the ethical concerns surrounding stem cell research, as iPSCs can be generated from adult somatic cells without the need to destroy embryos.
However, iPSC technology also raises ethical considerations, including the potential for off-target effects during reprogramming and the risk of tumorigenicity. Furthermore, the generation of iPSCs from adult cells raises questions about the ownership and control of the resulting cells and tissues. The use of human stem cells in research and therapy raises concerns about informed consent, privacy, and the potential for commercial exploitation. Clear ethical guidelines and regulations are needed to ensure that stem cell research is conducted responsibly and ethically.
Many thanks to our sponsor Esdebe who helped us prepare this research report.
5. Challenges and Limitations
Stem cell therapies face several challenges and limitations that need to be addressed to realize their full potential. These include:
- Tumorigenicity: ESCs and iPSCs have the potential to form tumors, particularly teratomas, due to their pluripotency. Careful differentiation and purification of stem cell-derived cells are crucial to mitigate this risk. Strategies to eliminate residual undifferentiated cells and to prevent tumorigenesis are being actively explored.
- Immune Rejection: Transplanted stem cells can be recognized as foreign by the host immune system and rejected. Immunosuppression is often required to prevent immune rejection, but it can increase the risk of infections and other complications. The use of autologous iPSCs can eliminate the risk of immune rejection, but it is a costly and time-consuming process. Encapsulation strategies and genetic engineering approaches are being developed to protect transplanted cells from immune attack.
- Differentiation Control: Achieving precise and controlled differentiation of stem cells into the desired cell type is a major challenge. The differentiation process is influenced by a complex interplay of signaling pathways, growth factors, and extracellular matrix components. Optimizing differentiation protocols and developing strategies to promote cell maturation and functionality are crucial for the success of stem cell therapies.
- Cell Delivery and Engraftment: Efficient delivery of stem cells to the target tissue and their successful engraftment are essential for tissue regeneration. However, many factors can affect cell delivery and engraftment, including the route of administration, the cell density, and the microenvironment of the target tissue. The development of biomaterials and tissue engineering approaches can enhance cell delivery and engraftment.
- Long-Term Efficacy and Safety: The long-term efficacy and safety of stem cell therapies need to be carefully evaluated. Clinical trials with long-term follow-up are necessary to assess the durability of the therapeutic effects and to monitor for any adverse events.
Many thanks to our sponsor Esdebe who helped us prepare this research report.
6. Future Directions
Stem cell research is a rapidly evolving field, with continuous advancements in stem cell biology, differentiation techniques, and delivery methods. Future directions in stem cell research include:
- Personalized Medicine: The development of personalized stem cell therapies using iPSCs offers the potential to tailor treatments to individual patients, eliminating the risk of immune rejection and improving therapeutic efficacy.
- Genome Editing: Genome editing technologies, such as CRISPR-Cas9, can be used to correct genetic defects in stem cells and to enhance their therapeutic potential. Genome editing can also be used to engineer stem cells to express specific growth factors or to be resistant to immune attack.
- 3D Bioprinting: 3D bioprinting is an emerging technology that allows for the creation of complex three-dimensional tissues and organs using stem cells and biomaterials. 3D bioprinting holds promise for generating functional tissues for transplantation and for drug screening.
- Exosomes: Exosomes are small vesicles secreted by cells that contain proteins, RNA, and other molecules. Exosomes derived from stem cells have been shown to have therapeutic effects in various diseases. Exosomes offer a cell-free approach to stem cell therapy, avoiding the risks associated with cell transplantation.
- Artificial Intelligence (AI): AI is playing an increasing role in stem cell research, from optimising culture conditions and differentiation protocols to predicting treatment outcomes and analysing large datasets. Machine learning algorithms can be used to identify novel biomarkers and to develop personalized treatment strategies.
Many thanks to our sponsor Esdebe who helped us prepare this research report.
7. Conclusion
Stem cell research has revolutionized our understanding of development, disease, and potential therapeutic interventions. Stem cells hold immense promise for regenerative medicine, offering potential cures and treatments for a vast array of diseases. However, the application of stem cell therapies is not without its challenges, including ethical concerns, technical hurdles, and safety considerations. Ongoing research and technological advancements are continuously addressing these challenges, paving the way for the development of safe and effective stem cell therapies for a wide range of diseases. Personalized medicine approaches, genome editing, 3D bioprinting, and exosomes represent exciting future directions in stem cell research. By addressing the ethical and technical challenges, stem cell therapy has the potential to transform healthcare and improve the lives of millions of people.
Many thanks to our sponsor Esdebe who helped us prepare this research report.
References
[1] Takahashi, K., Tanabe, K., Ohnuki, M., Narita, M., Ichisaka, T., Tomoda, K., & Yamanaka, S. (2007). Induction of pluripotent stem cells from adult human fibroblasts by defined factors. Cell, 131(5), 861-872.
[2] Thomson, J. A., Itskovitz-Eldor, J., Shapiro, S. S., Waknitz, M. A., Swiergiel, J. J., Marshall, V. S., & Jones, J. M. (1998). Embryonic stem cell lines derived from human blastocysts. Science, 282(5391), 1145-1147.
[3] Boyer, L. A., Lee, T. I., Cole, M. F., Johnstone, S. E., Levine, S. S., Zucker, J. P., … & Young, R. A. (2005). Core transcriptional regulatory circuitry in human embryonic stem cells. Cell, 122(6), 947-956.
[4] Fuchs, E., & Segre, J. A. (2000). Stem cells: a new lease on life. Cell, 100(1), 143-155.
[5] Shapiro, A. M., Ricordi, C., Hering, B. J., Auchincloss, H., Lindblad, R., Robertson, R. P., … & Lakey, J. R. (2000). Islet transplantation in seven patients with type 1 diabetes mellitus using a glucocorticoid-free immunosuppressive regimen. New England Journal of Medicine, 343(4), 230-238.
[6] Ramzy A, Thompson D, James R, Rushton S, van der Werf M, Senior P, Light PE, Rezania A, Kieffer TJ, Warnock GL. Stem cell-derived islets provide insulin independence in a patient with type 1 diabetes. Cell Stem Cell. 2023 Apr 6;30(4):370-381.e7. doi: 10.1016/j.stem.2023.03.005. PMID: 37023709; PMCID: PMC10083369.
[7] Lindvall, O., & Hagell, P. (2000). Clinical transplantation in Parkinson’s disease. Experimental neurology, 163(1), 1-16.
[8] Schweitzer, J. S., Song, B., Herrington, T. M., Park, T. Y., Vathsangam, H., Sullivan, A. M., … & Bankiewicz, K. S. (2020). Personalized iPSC-derived dopamine progenitor cells for Parkinson’s disease. New England Journal of Medicine, 382(20), 1926-1932.
[9] Murry, C. E., Soonpaa, M. H., Reinecke, H., Nakajima, H., Nakajima, Y., Rubart, M., … & Field, L. J. (2004). Human embryonic stem cells possess myocardial differentiation potential. Annals of the New York Academy of Sciences, 1015, 140-150.
[10] Fassas, A., Anagnostopoulos, A., Kazis, A., Kapetanidis, S., Sakellariou, G., Kim, Y. J., … & Griva, T. (2005). Peripheral blood stem cell transplantation in progressive multiple sclerosis: first results of a pilot study. Bone marrow transplantation, 35(7), 691-696.
[11] Robertson, J. A. (1999). Ethics and policy in embryo research. The Cambridge quarterly of healthcare ethics, 8(4), 429-442.
The discussion of ethical considerations is crucial. As iPSC technology advances, how can we ensure equitable access and prevent potential disparities in benefiting from personalized stem cell therapies?
That’s a vital point! Equitable access is key. As iPSC therapies become more refined, focusing on lowering production costs and establishing resource sharing networks could help bridge potential gaps in access. Perhaps government funding and collaborative research initiatives can play a role too? Let’s keep this conversation going!
Editor: MedTechNews.Uk
Thank you to our Sponsor Esdebe