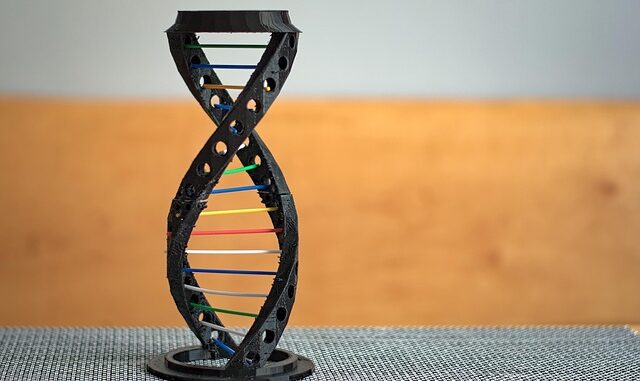
Abstract
Personalized medicine, also known as precision medicine, represents a paradigm shift in healthcare, moving away from a one-size-fits-all approach to tailored treatments based on an individual’s unique genetic, environmental, and lifestyle factors. This report provides a comprehensive overview of the current state of personalized medicine, focusing on the advances in targeted therapies across various disease areas, the challenges encountered in translating genetic and genomic information into clinical practice, and the future directions that hold promise for revolutionizing healthcare. We explore the ethical, regulatory, and economic considerations that accompany the widespread adoption of personalized medicine, highlighting the need for interdisciplinary collaboration and innovative solutions to overcome existing barriers. This report aims to provide insights for researchers, clinicians, policymakers, and industry stakeholders involved in shaping the future of personalized medicine.
Many thanks to our sponsor Esdebe who helped us prepare this research report.
1. Introduction
The traditional medical model often relies on broad-spectrum treatments that may not be equally effective for all patients. Personalized medicine seeks to address this limitation by leveraging advances in genomics, proteomics, metabolomics, and other omics technologies to gain a deeper understanding of the molecular mechanisms underlying disease and individual patient variability. By identifying specific biomarkers and genetic variants that influence disease susceptibility, progression, and treatment response, personalized medicine aims to develop targeted therapies that are more effective, safer, and more cost-effective than conventional approaches [1].
The Human Genome Project, completed in 2003, served as a catalyst for the development of personalized medicine, providing a foundational understanding of the human genome and paving the way for the identification of disease-associated genes and genetic variants [2]. Since then, technological advancements in DNA sequencing, high-throughput screening, and bioinformatics have accelerated the pace of discovery and enabled the development of sophisticated diagnostic tools and therapeutic strategies.
This report will delve into the key areas of personalized medicine, including: (1) the application of pharmacogenomics to optimize drug selection and dosing; (2) the development of targeted therapies for cancer based on tumor-specific mutations; (3) the use of genetic risk scores to predict disease susceptibility and guide preventive interventions; (4) the challenges in translating genomic findings into clinical practice; and (5) the ethical, regulatory, and economic considerations surrounding personalized medicine.
Many thanks to our sponsor Esdebe who helped us prepare this research report.
2. Pharmacogenomics: Tailoring Drug Therapy to Individual Genotypes
Pharmacogenomics, a cornerstone of personalized medicine, examines the influence of genetic variations on drug response [3]. By identifying genes that encode drug-metabolizing enzymes, transporters, and receptors, pharmacogenomics aims to predict how individuals will respond to specific medications and optimize drug selection and dosing accordingly. This approach can help minimize adverse drug reactions, improve treatment efficacy, and reduce healthcare costs.
2.1. Cytochrome P450 Enzymes and Drug Metabolism
The cytochrome P450 (CYP) family of enzymes plays a critical role in the metabolism of many commonly prescribed drugs. Genetic variations in CYP genes, such as CYP2C9, CYP2C19, and CYP2D6, can affect enzyme activity, leading to differences in drug clearance and plasma concentrations. For example, individuals with CYP2C19 loss-of-function alleles may exhibit reduced metabolism of clopidogrel, an antiplatelet drug, increasing their risk of cardiovascular events [4]. Conversely, individuals with CYP2D6 ultrarapid metabolizer genotypes may require higher doses of certain antidepressants, such as selective serotonin reuptake inhibitors (SSRIs), to achieve therapeutic efficacy [5].
2.2. Clinical Applications of Pharmacogenomics
Pharmacogenomic testing is increasingly being incorporated into clinical practice to guide drug therapy in various disease areas. For instance, TPMT genotyping is recommended before initiating treatment with azathioprine or 6-mercaptopurine, immunosuppressant drugs used in the treatment of inflammatory bowel disease and leukemia, to identify individuals at risk of severe myelosuppression [6]. Similarly, UGT1A1 genotyping can help predict the risk of toxicity associated with irinotecan, a chemotherapy drug used in the treatment of colorectal cancer [7].
2.3. Challenges and Future Directions in Pharmacogenomics
Despite the growing evidence supporting the clinical utility of pharmacogenomics, several challenges remain. These include the limited availability of pharmacogenomic testing in some healthcare settings, the lack of standardization in testing methodologies and result interpretation, and the need for more robust clinical trials to demonstrate the cost-effectiveness of pharmacogenomic-guided therapy [8]. Future directions in pharmacogenomics include the development of polygenic risk scores that incorporate multiple genetic variants to predict drug response, the integration of pharmacogenomic data into electronic health records to facilitate clinical decision-making, and the use of artificial intelligence and machine learning to identify novel pharmacogenomic biomarkers [9].
Many thanks to our sponsor Esdebe who helped us prepare this research report.
3. Targeted Cancer Therapies: Precision Oncology
Cancer is a heterogeneous disease characterized by a complex interplay of genetic and environmental factors. Targeted cancer therapies aim to selectively inhibit the growth and survival of cancer cells by targeting specific molecular alterations that drive tumorigenesis [10]. These therapies offer the potential to improve treatment outcomes and reduce toxicity compared to traditional chemotherapy.
3.1. Identification of Cancer-Specific Mutations
Next-generation sequencing (NGS) technologies have revolutionized cancer genomics, enabling the rapid and cost-effective identification of somatic mutations and other genomic alterations in tumor tissue. These mutations can serve as targets for the development of personalized cancer therapies. For example, mutations in the EGFR gene are commonly found in non-small cell lung cancer (NSCLC) and predict sensitivity to EGFR tyrosine kinase inhibitors (TKIs) such as gefitinib, erlotinib, and osimertinib [11]. Similarly, mutations in the BRAF gene are frequently observed in melanoma and predict response to BRAF inhibitors such as vemurafenib and dabrafenib [12].
3.2. Development of Targeted Cancer Therapies
The identification of cancer-specific mutations has led to the development of a growing number of targeted cancer therapies. These therapies include small-molecule inhibitors that block the activity of oncogenic proteins, monoclonal antibodies that target cell-surface receptors, and antibody-drug conjugates that deliver cytotoxic agents directly to cancer cells [13]. Immune checkpoint inhibitors, such as pembrolizumab and nivolumab, represent another class of targeted cancer therapies that unleash the body’s own immune system to attack cancer cells [14].
3.3. Challenges and Future Directions in Precision Oncology
While targeted cancer therapies have shown remarkable success in some patients, several challenges remain. These include the development of resistance to targeted therapies, the heterogeneity of tumors, and the limited availability of targeted therapies for certain cancer types [15]. Future directions in precision oncology include the development of combination therapies that target multiple oncogenic pathways, the use of liquid biopsies to monitor treatment response and detect resistance mutations, and the development of personalized cancer vaccines that stimulate an immune response against tumor-specific antigens [16]. Moreover, advances in CRISPR-Cas9 gene editing technology offer the potential to correct cancer-causing mutations directly in cancer cells [17]. This approach, while still in its early stages, holds immense promise for the future of cancer therapy.
Many thanks to our sponsor Esdebe who helped us prepare this research report.
4. Genetic Risk Scores and Preventive Interventions
Genetic risk scores (GRS) combine the effects of multiple genetic variants to estimate an individual’s overall risk of developing a particular disease [18]. By identifying individuals at high risk, GRS can be used to guide preventive interventions and improve health outcomes.
4.1. Construction of Genetic Risk Scores
GRS are typically constructed by summing the number of risk alleles an individual carries, weighted by the effect size of each allele. The effect size is usually estimated from genome-wide association studies (GWAS), which identify genetic variants associated with disease risk in large populations. GRS can be used to predict the risk of various diseases, including cardiovascular disease, type 2 diabetes, and breast cancer [19].
4.2. Application of Genetic Risk Scores in Clinical Practice
GRS have the potential to be used in clinical practice to identify individuals who would benefit most from preventive interventions. For example, individuals with a high GRS for cardiovascular disease may be more likely to benefit from lifestyle modifications, such as diet and exercise, or from preventive medications, such as statins [20]. Similarly, women with a high GRS for breast cancer may be more likely to benefit from increased surveillance, such as annual mammograms or MRI scans, or from risk-reducing medications, such as tamoxifen [21].
4.3. Challenges and Future Directions in Genetic Risk Scoring
Several challenges remain in the development and application of GRS. These include the need for larger and more diverse GWAS to identify more disease-associated genetic variants, the limited predictive accuracy of GRS for some diseases, and the potential for GRS to exacerbate health disparities [22]. Future directions in genetic risk scoring include the development of dynamic GRS that incorporate environmental and lifestyle factors, the use of machine learning to improve the accuracy of GRS, and the integration of GRS into electronic health records to facilitate clinical decision-making [23]. An important consideration is also the ethical implication of using GRS, particularly concerning potential discrimination and the need for robust data privacy measures.
Many thanks to our sponsor Esdebe who helped us prepare this research report.
5. Challenges in Translating Genomic Findings into Clinical Practice
Despite the remarkable advances in genomics and personalized medicine, several challenges remain in translating genomic findings into clinical practice [24]. These challenges include:
- Lack of Clinical Utility: Many genetic variants identified in GWAS have small effect sizes and limited predictive accuracy, making it difficult to translate them into clinically meaningful interventions.
- Limited Diversity in Genomic Studies: Most genomic studies have been conducted in populations of European ancestry, limiting the generalizability of findings to other ethnic groups.
- Regulatory Hurdles: The regulatory landscape for genomic testing and personalized medicine is complex and evolving, creating uncertainty for developers and clinicians.
- Cost Considerations: Genomic testing can be expensive, limiting its accessibility to many patients. Furthermore, the cost-effectiveness of personalized medicine interventions needs to be rigorously evaluated.
- Ethical, Legal, and Social Implications (ELSI): Personalized medicine raises several ethical concerns, including the privacy and security of genetic information, the potential for genetic discrimination, and the need for informed consent and genetic counseling.
Many thanks to our sponsor Esdebe who helped us prepare this research report.
6. Ethical, Regulatory, and Economic Considerations
The widespread adoption of personalized medicine raises several ethical, regulatory, and economic considerations that need to be addressed to ensure its responsible and equitable implementation [25].
6.1. Ethical Considerations
The ethical considerations surrounding personalized medicine include:
- Privacy and Security of Genetic Information: Genetic information is highly sensitive and personal, and its privacy and security must be protected to prevent unauthorized access and misuse. Strong data encryption and access controls are essential.
- Genetic Discrimination: Genetic information could be used to discriminate against individuals in employment, insurance, or other areas. Laws and policies are needed to protect individuals from genetic discrimination.
- Informed Consent: Patients must be fully informed about the risks and benefits of genomic testing and personalized medicine interventions before providing consent. Genetic counseling should be readily available to help patients understand the implications of genetic testing results.
- Equity of Access: Personalized medicine should be accessible to all individuals, regardless of their socioeconomic status or geographic location. Efforts are needed to reduce health disparities and ensure that all patients have equal access to the benefits of personalized medicine.
6.2. Regulatory Considerations
The regulatory landscape for genomic testing and personalized medicine is complex and evolving. The US Food and Drug Administration (FDA) has regulatory authority over genetic tests and personalized medicine interventions. The FDA has issued guidance on the regulation of laboratory-developed tests (LDTs), which are genetic tests developed and performed in a single laboratory. However, the regulation of LDTs remains a controversial issue [26]. There’s a debate about whether FDA oversight should be increased to ensure the accuracy and reliability of these tests. The European Medicines Agency (EMA) also plays a crucial role in regulating personalized medicine products in Europe.
6.3. Economic Considerations
The economic considerations surrounding personalized medicine include:
- Cost-Effectiveness: The cost-effectiveness of personalized medicine interventions needs to be rigorously evaluated to determine whether they provide sufficient value for the investment.
- Reimbursement Policies: Reimbursement policies need to be developed to ensure that personalized medicine interventions are adequately reimbursed by payers. There’s a need for healthcare systems to adapt their reimbursement models to accommodate the shift towards personalized treatments, often requiring sophisticated diagnostic tests.
- Infrastructure Investments: Significant investments are needed in infrastructure, such as electronic health records, biobanks, and data analytics platforms, to support the implementation of personalized medicine.
Many thanks to our sponsor Esdebe who helped us prepare this research report.
7. Future Directions
Personalized medicine is a rapidly evolving field with immense potential to transform healthcare. Future directions in personalized medicine include:
- Integration of Multi-Omics Data: Integrating data from genomics, proteomics, metabolomics, and other omics technologies will provide a more comprehensive understanding of disease and individual patient variability.
- Development of Novel Biomarkers: Identifying novel biomarkers that predict disease risk, progression, and treatment response will enable the development of more targeted and effective therapies.
- Use of Artificial Intelligence and Machine Learning: Applying artificial intelligence and machine learning to analyze large datasets will accelerate the discovery of new insights and improve the accuracy of personalized medicine interventions.
- Expansion of Personalized Medicine to New Disease Areas: Expanding the application of personalized medicine to new disease areas, such as mental health, infectious diseases, and autoimmune disorders, will improve health outcomes for a wider range of patients.
- Patient Engagement and Empowerment: Engaging patients in the development and implementation of personalized medicine will ensure that treatments are aligned with their values and preferences.
Many thanks to our sponsor Esdebe who helped us prepare this research report.
8. Conclusion
Personalized medicine holds tremendous promise for revolutionizing healthcare by tailoring treatments to individual patients based on their unique characteristics. While significant progress has been made in recent years, several challenges remain in translating genomic findings into clinical practice and ensuring the responsible and equitable implementation of personalized medicine. By addressing these challenges and fostering interdisciplinary collaboration, personalized medicine can transform healthcare and improve the lives of patients worldwide.
Many thanks to our sponsor Esdebe who helped us prepare this research report.
References
[1] Hamburg, M. A., & Collins, F. S. (2010). The path to personalized medicine. New England Journal of Medicine, 363(4), 301-304.
[2] Lander, E. S., Linton, L. M., Birren, B., Nusbaum, C., Zody, M. C., Baldwin, J., … & Waterston, R. H. (2001). Initial sequencing and analysis of the human genome. Nature, 409(6822), 860-921.
[3] Pirmohamed, M. (2011). Pharmacogenomics: past, present and future. British Journal of Clinical Pharmacology, 73(1), 1-3.
[4] Mega, J. L., Close, S. L., Wiviott, S. D., Shen, L., Walker, P. L., Macias, W., … & Antman, E. M. (2009). Cytochrome p-450 polymorphisms and response to clopidogrel. New England Journal of Medicine, 360(4), 354-362.
[5] Hicks, J. K., Sangkuhl, K., Swen, J. J., Ellingrod, V. L., Müller, D. J., Shimoda, K., … & Gaedigk, A. (2015). Clinical pharmacogenetics implementation consortium guideline (CPIC) for CYP2D6 and certain antidepressants. Clinical Pharmacology & Therapeutics, 98(2), 127-134.
[6] Relling, M. V., Schwab, M., Whirl-Carrillo, M., Suarez-Kurtz, G., Pui, C. H., Stein, C. M., … & Caudle, K. E. (2019). Clinical Pharmacogenetics Implementation Consortium (CPIC) guideline for thiopurine methyltransferase genotype and thiopurine dosing: 2018 update. Clinical Pharmacology & Therapeutics, 105(5), 1091-1114.
[7] Innocenti, F., Schilsky, R. L., Ramirez, J., Janisch, L., Undevia, S., Krishna, M., … & Ratain, M. J. (2009). UGT1A1 genotype and irinotecan-induced neutropenia: prospective validation in a multicenter clinical trial. Journal of Clinical Oncology, 27(9), 1451-1457.
[8] Manolio, T. A., Abramson, J., Bult, C. J., Chakravarti, A., Collins, F. S., Crow, J. F., … & Veenstra, J. A. (2009). New models of collaboration in genome-wide association studies: the Genetic Association Information Network. Nature Genetics, 41(7), 701-707.
[9] Ritchie, M. D. (2012). Artificial intelligence and machine learning: opportunities and challenges in pharmacogenomics. Clinical Pharmacology & Therapeutics, 91(6), 978-981.
[10] Sawyers, C. L. (2004). The cancer biomarker problem. Nature, 432(7015), 294-298.
[11] Lynch, T. J., Bell, D. W., Sordella, R., Gurubhagavatula, S., Okimoto, R. A., Brannigan, B. W., … & Haber, D. A. (2004). Activating mutations in the epidermal growth factor receptor underlying responsiveness of non-small-cell lung cancer to gefitinib. New England Journal of Medicine, 350(21), 2129-2139.
[12] Chapman, P. B., Amoretti, S., Ribas, A., Flaherty, K. T., Kim, K. B., Sosman, J. A., … & Kirkwood, J. M. (2011). Improved survival with vemurafenib in melanoma with BRAF V600E mutation. New England Journal of Medicine, 364(26), 2507-2516.
[13] Jain, R. K. (2014). Normalizing tumor microenvironment to treat cancer: bench to bedside to bench. Nature Medicine, 20(5), 471-482.
[14] Topalian, S. L., Hodi, F. S., Brahmer, J. R., Gettinger, S. N., Smith, D. C., McDermott, D. F., … & Pardoll, D. M. (2012). Safety, activity, and immune correlates of anti-PD-1 antibody in cancer. New England Journal of Medicine, 366(26), 2443-2454.
[15] Holohan, C., Van Schaeybroeck, S., Longley, D. B., & Johnston, P. G. (2013). Cancer drug resistance: an evolving paradigm. Nature Reviews Cancer, 13(10), 714-726.
[16] Rosenberg, S. A., Restifo, N. P., Yang, J. C., Morgan, R. A., & Marincola, F. M. (2008). Adoptive cell transfer: a clinical path to effective cancer immunotherapy. Nature Reviews Cancer, 8(4), 299-308.
[17] Doudna, J. A., & Charpentier, E. (2014). Genome editing. Science, 346(6213), 1258096.
[18] Torkamani, A., Wineinger, N. E., & Topol, E. J. (2018). The personal and clinical utility of polygenic risk scores. Nature Reviews Genetics, 19(9), 581-590.
[19] Khera, A. V., Chaffin, M., Aragam, K. G., Haas, M. E., Roselli, C., Choi, S. H., … & Natarajan, P. (2018). Genome-wide polygenic scores for common diseases identify individuals with risk equivalent to monogenic mutations. Nature Genetics, 50(9), 1219-1224.
[20] Knowles, J. W., & Ashley, E. A. (2018). Cardiovascular disease: the coming age of genomic risk prediction. JAMA cardiology, 3(6), 574-575.
[21] Mavaddat, N., Michailidou, K., Dennis, J., Lush, M., Ahmed, S., Maranian, M. J., … & Easton, D. F. (2015). Polygenic risk scores for prediction of breast cancer and breast cancer subtypes. The American Journal of Human Genetics, 97(5), 672-681.
[22] Martin, A. R., Kanai, M., Kamatani, Y., Okada, Y., Neale, B. M., & Daly, M. J. (2019). Clinical use of human genetics in diverse global populations. Nature Genetics, 51(4), 584-591.
[23] Wald, N. J., Old, R., & Huttly, W. J. (2019). Polygenic risk scores: current status and prospects. Archives of Disease in Childhood, 104(1), 81-86.
[24] Khoury, M. J., Bowen, S., Clyne, M., & Dotson, W. D. (2013). From genome-wide association studies to personalized medicine: a call for network biology and systems approaches. American Journal of Epidemiology, 178(12), 1781-1787.
[25] Ashley, E. A. (2015). Towards precision medicine. Nature Reviews Genetics, 16(9), 507-522.
[26] Evans, J. P., Watson, M. S., & Javitt, G. H. (2015). Regulation of laboratory-developed tests: time for a change. JAMA, 313(7), 669-670.
Personalized medicine, eh? So, will my Spotify algorithm finally prescribe me the exact right song to cure Monday morning blues? I’m holding out hope for a genome-tailored playlist!
That’s a fantastic analogy! Imagine a future where your genome informs not just your medical treatments, but also your entertainment. Perhaps we’ll see AI algorithms crafting personalized art experiences based on our unique biological profiles. The possibilities are endless!
Editor: MedTechNews.Uk
Thank you to our Sponsor Esdebe