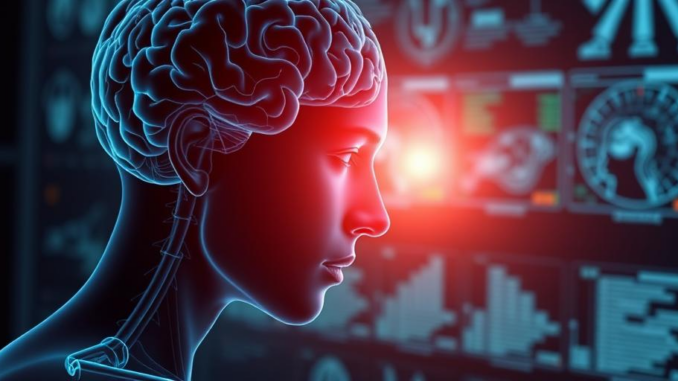
Abstract
Transcranial Magnetic Stimulation (TMS) has emerged as a powerful non-invasive neuromodulation technique with therapeutic potential for a range of neuropsychiatric disorders. Initially developed as a diagnostic tool, TMS has since gained FDA clearance for the treatment of major depressive disorder (MDD) and obsessive-compulsive disorder (OCD), with ongoing research exploring its efficacy in treating other conditions such as anxiety disorders, post-traumatic stress disorder (PTSD), and even neurological disorders like Parkinson’s disease and stroke. This report provides a comprehensive overview of TMS, delving into the underlying principles of the technology, its diverse applications, mechanisms of action, safety profile, and emerging research directions. We explore the technological advancements in TMS coil design and stimulation protocols, focusing on their impact on treatment efficacy and specificity. Furthermore, we discuss the challenges and opportunities in identifying reliable biomarkers to predict individual treatment response and personalize TMS therapy. Finally, we consider the future of TMS in integrated treatment paradigms, highlighting the potential of combining TMS with other therapeutic modalities, such as psychotherapy and pharmacological interventions, to achieve optimal clinical outcomes.
Many thanks to our sponsor Esdebe who helped us prepare this research report.
1. Introduction
Neuromodulation techniques are revolutionizing the field of neuropsychiatry by offering targeted interventions that can modulate brain activity and restore neural circuits implicated in mental disorders. Among these techniques, transcranial magnetic stimulation (TMS) stands out as a non-invasive and versatile tool with a growing body of evidence supporting its clinical efficacy. TMS utilizes electromagnetic induction to generate pulsed magnetic fields that pass through the scalp and skull, inducing electrical currents in the underlying brain tissue. These induced currents can depolarize or hyperpolarize neurons, depending on the stimulation parameters, and thereby modulate neuronal excitability and synaptic plasticity. This capability makes TMS a unique tool for investigating brain function and treating a variety of neurological and psychiatric conditions.
The initial development of TMS focused primarily on its diagnostic applications, allowing researchers and clinicians to map cortical excitability and assess motor pathways. However, the observation that repeated TMS (rTMS) could induce longer-lasting changes in brain activity led to its exploration as a potential therapeutic intervention. This marked a significant shift in the application of TMS, transforming it from a diagnostic tool to a powerful therapeutic modality.
Since receiving FDA clearance for the treatment of MDD in 2008, TMS has become increasingly integrated into clinical practice, offering a valuable alternative for patients who have not responded adequately to conventional treatments like medication and psychotherapy. Its non-invasive nature, coupled with its relatively benign side effect profile compared to other brain stimulation techniques like electroconvulsive therapy (ECT), has further contributed to its growing popularity. However, despite its established clinical efficacy in MDD, significant challenges remain in optimizing TMS protocols, identifying patient-specific predictors of treatment response, and expanding its applications to other neuropsychiatric disorders.
This report aims to provide a comprehensive overview of TMS, covering its technological underpinnings, mechanisms of action, clinical applications, safety profile, and future directions. We will discuss the key advancements in TMS technology, including coil design and stimulation protocols, and their impact on treatment efficacy and specificity. Furthermore, we will explore the challenges and opportunities in personalizing TMS therapy by identifying reliable biomarkers that can predict individual treatment response. Finally, we will consider the future of TMS in integrated treatment paradigms, highlighting the potential of combining TMS with other therapeutic modalities to achieve optimal clinical outcomes.
Many thanks to our sponsor Esdebe who helped us prepare this research report.
2. Technology and Mechanisms of Action
2.1. TMS Technology
The core component of a TMS system is the stimulating coil, which generates the pulsed magnetic field. The shape and configuration of the coil determine the focality and depth of stimulation. Figure-8 coils are the most commonly used coil type in clinical TMS, as they offer greater focality compared to circular coils. The magnetic pulse generated by the coil induces an electrical current in the underlying brain tissue, primarily targeting neurons within a few centimeters of the coil.
The intensity of the magnetic pulse is typically expressed as a percentage of the stimulator’s maximum output. The frequency of stimulation, measured in Hertz (Hz), determines whether the TMS is considered to be excitatory or inhibitory. High-frequency stimulation (e.g., ≥5 Hz) is generally considered to be excitatory, increasing neuronal excitability, while low-frequency stimulation (e.g., ≤1 Hz) is typically inhibitory, decreasing neuronal excitability.
Recent advancements in TMS technology have focused on improving the focality and depth of stimulation. Deep TMS (dTMS) coils, for example, are designed to stimulate deeper brain regions compared to conventional figure-8 coils. These coils utilize a different coil geometry, such as the H-coil, to generate a more diffuse magnetic field that can penetrate deeper into the brain.
Another area of advancement is in the development of neuronavigation systems. These systems use real-time imaging to guide coil placement and ensure accurate targeting of specific brain regions. Neuronavigation can also be used to track coil position during treatment, minimizing variability and improving the consistency of stimulation.
2.2. Mechanisms of Action
The precise mechanisms by which TMS exerts its therapeutic effects are not fully understood, but a growing body of evidence suggests that TMS modulates neural circuits through a variety of mechanisms, including:
- Synaptic Plasticity: TMS can induce long-term potentiation (LTP) or long-term depression (LTD)-like changes in synaptic strength, depending on the stimulation parameters. LTP strengthens synaptic connections, while LTD weakens them. These changes in synaptic plasticity are believed to underlie the long-lasting therapeutic effects of TMS.
- Neurotransmitter Release: TMS can modulate the release of neurotransmitters such as dopamine, serotonin, and norepinephrine. These neurotransmitters play a critical role in regulating mood, motivation, and cognitive function. By modulating neurotransmitter release, TMS can potentially restore balance to dysregulated neurotransmitter systems in neuropsychiatric disorders.
- Neurogenesis: Some studies suggest that TMS may promote neurogenesis, the formation of new neurons, in specific brain regions. Neurogenesis is thought to play a role in learning, memory, and mood regulation. While the evidence for TMS-induced neurogenesis is still limited, it represents a potentially important mechanism of action.
- Brain Network Modulation: TMS can modulate the activity and connectivity of large-scale brain networks. For example, TMS applied to the dorsolateral prefrontal cortex (DLPFC) can modulate activity in the default mode network (DMN), a brain network that is implicated in rumination and self-referential thought. By modulating brain network activity, TMS can potentially disrupt maladaptive patterns of neural activity and promote more adaptive patterns.
These mechanisms are not mutually exclusive and likely interact in complex ways to mediate the therapeutic effects of TMS. Future research is needed to further elucidate the precise mechanisms of action and identify the specific neural circuits that are targeted by TMS in different neuropsychiatric disorders.
Many thanks to our sponsor Esdebe who helped us prepare this research report.
3. Clinical Applications
3.1. Major Depressive Disorder (MDD)
MDD is the most well-established clinical application of TMS. Numerous randomized controlled trials have demonstrated the efficacy of TMS in treating MDD, particularly in patients who have not responded adequately to antidepressant medications. TMS is typically administered to the left DLPFC, a brain region that is implicated in mood regulation. The standard protocol for TMS in MDD involves high-frequency (e.g., 10 Hz) stimulation for several weeks.
Meta-analyses of clinical trials have shown that TMS is significantly more effective than sham stimulation in reducing depressive symptoms. The response rates to TMS in MDD range from 30% to 50%, with remission rates ranging from 20% to 40%. While TMS is generally well-tolerated, some patients may experience mild side effects such as headache or scalp discomfort.
Theta Burst Stimulation (TBS) is a relatively newer TMS protocol that has shown promise in treating MDD. TBS involves delivering bursts of high-frequency stimulation at a lower overall intensity. Intermittent TBS (iTBS) is an excitatory protocol, while continuous TBS (cTBS) is an inhibitory protocol. Some studies suggest that iTBS may be as effective as traditional high-frequency TMS in treating MDD, but with shorter treatment times.
3.2. Obsessive-Compulsive Disorder (OCD)
OCD is another neuropsychiatric disorder for which TMS has received FDA clearance. In OCD, TMS is typically administered to the medial prefrontal cortex (mPFC), specifically the supplementary motor area (SMA). The protocol typically involves low-frequency (e.g., 1 Hz) stimulation of the SMA.
Clinical trials have demonstrated that TMS is more effective than sham stimulation in reducing obsessive-compulsive symptoms. The response rates to TMS in OCD range from 30% to 45%. Similar to MDD, TMS is generally well-tolerated in OCD patients.
3.3. Other Mental Health Conditions
In addition to MDD and OCD, TMS is being investigated as a treatment for a variety of other mental health conditions, including:
- Anxiety Disorders: Studies have explored the use of TMS in treating generalized anxiety disorder (GAD), social anxiety disorder (SAD), and panic disorder. The targets and protocols vary depending on the specific anxiety disorder, but generally, the prefrontal cortex is the area of most interest.
- Post-Traumatic Stress Disorder (PTSD): TMS is being investigated as a potential treatment for PTSD, targeting areas such as the DLPFC and the amygdala, to modulate emotional processing and memory consolidation.
- Substance Use Disorders: TMS is being explored as a treatment for addiction, targeting brain regions involved in reward and craving, such as the DLPFC and the anterior cingulate cortex (ACC).
- Schizophrenia: TMS has been investigated as a treatment for negative symptoms of schizophrenia, such as apathy and social withdrawal, as well as auditory hallucinations. Results have been mixed, but further research is warranted.
3.4. Neurological Disorders
TMS is also being explored as a treatment for a variety of neurological disorders, including:
- Parkinson’s Disease: TMS has shown some promise in improving motor symptoms and cognitive function in Parkinson’s disease. The primary target is the motor cortex, where it can improve neural connectivity.
- Stroke: TMS is being investigated as a tool to promote motor recovery after stroke. Stimulation is usually focused on the motor cortex or premotor cortex.
- Chronic Pain: TMS has been investigated as a treatment for chronic pain conditions such as fibromyalgia and neuropathic pain. Targets vary depending on the specific type of pain.
While the evidence for TMS in these neurological disorders is still preliminary, it represents a promising avenue for future research.
Many thanks to our sponsor Esdebe who helped us prepare this research report.
4. Safety and Tolerability
TMS is generally considered to be a safe and well-tolerated treatment. The most common side effects are mild and transient, including headache, scalp discomfort, and muscle twitching. These side effects typically resolve within a few hours of treatment and can often be managed with over-the-counter pain relievers.
The most serious potential risk associated with TMS is seizure induction. However, the risk of seizure is very low, estimated to be less than 0.1% in clinical trials. To minimize the risk of seizure, clinicians follow strict safety guidelines, including screening patients for contraindications, such as a history of seizures or other neurological disorders. Additionally, the stimulation parameters are carefully adjusted to avoid exceeding the individual’s seizure threshold.
Other potential but rare side effects of TMS include changes in mood, cognition, or sleep. These side effects are typically transient and resolve after treatment is discontinued. However, it is important to monitor patients closely for any adverse effects and to adjust the treatment protocol accordingly.
Overall, the safety profile of TMS is favorable compared to other brain stimulation techniques such as ECT. TMS does not require anesthesia or muscle relaxants, and it is associated with fewer cognitive side effects than ECT.
Many thanks to our sponsor Esdebe who helped us prepare this research report.
5. Patient Selection and Treatment Process
5.1. Patient Selection Criteria
Careful patient selection is crucial for optimizing the efficacy and safety of TMS therapy. The ideal candidate for TMS is a patient with a diagnosis of MDD or OCD who has not responded adequately to conventional treatments such as medication and psychotherapy. Patients with certain contraindications, such as a history of seizures, implanted metallic devices in the head, or unstable medical conditions, are typically excluded from TMS therapy.
Other factors that may influence treatment response include the severity of depression, the duration of illness, and the presence of comorbid psychiatric conditions. Some studies suggest that patients with more severe depression or a longer duration of illness may be less likely to respond to TMS. Patients with comorbid anxiety disorders or personality disorders may also have a lower response rate.
5.2. Treatment Process
The TMS treatment process typically involves the following steps:
- Initial Evaluation: The patient undergoes a thorough psychiatric evaluation to determine their suitability for TMS therapy.
- Motor Threshold Determination: The motor threshold (MT) is determined by stimulating the motor cortex and measuring the minimum intensity of stimulation required to elicit a muscle twitch in the hand. The MT is used to individualize the stimulation intensity for each patient.
- Coil Placement: The TMS coil is placed over the target brain region, such as the left DLPFC in MDD. Neuronavigation systems may be used to guide coil placement and ensure accurate targeting.
- Stimulation: The TMS pulses are delivered according to a specific protocol. The duration and frequency of stimulation vary depending on the protocol and the condition being treated. A typical course of TMS therapy involves daily treatments for several weeks.
- Monitoring: The patient is closely monitored for any side effects during and after each treatment session.
- Maintenance Therapy: After the initial course of TMS therapy, some patients may benefit from maintenance therapy to prevent relapse. Maintenance therapy may involve periodic TMS treatments or other forms of treatment, such as medication or psychotherapy.
Many thanks to our sponsor Esdebe who helped us prepare this research report.
6. Cost-Effectiveness
The cost-effectiveness of TMS is an important consideration for healthcare providers and patients. TMS therapy is generally more expensive than medication, but it may be more cost-effective than other treatments, such as ECT or hospitalization, particularly in patients who have not responded to medication.
Several studies have examined the cost-effectiveness of TMS in MDD. These studies have generally found that TMS is cost-effective compared to other treatments, particularly over the long term. The cost-effectiveness of TMS is influenced by factors such as the response rate, the remission rate, and the duration of treatment effects.
Furthermore, the integration of TMS into existing healthcare systems can impact its cost-effectiveness. Streamlining the treatment process and optimizing patient selection can help to reduce the overall cost of TMS therapy.
Many thanks to our sponsor Esdebe who helped us prepare this research report.
7. Biomarkers and Personalized TMS Therapy
One of the major challenges in TMS research is identifying reliable biomarkers that can predict individual treatment response. Currently, TMS therapy is often administered on a trial-and-error basis, with no way to predict in advance which patients will respond to treatment. Identifying biomarkers that can predict treatment response would allow clinicians to personalize TMS therapy and improve treatment outcomes.
Several potential biomarkers have been investigated, including:
- Electroencephalography (EEG): EEG can be used to measure brain activity at rest or in response to TMS. Some studies have found that specific EEG features, such as alpha power or theta power, are associated with treatment response. EEG can be used to assess cortical excitability before and after TMS.
- Functional Magnetic Resonance Imaging (fMRI): fMRI can be used to measure brain activity during cognitive tasks or at rest. Some studies have found that specific fMRI measures, such as functional connectivity or regional brain activity, are associated with treatment response. fMRI can be used to identify brain regions that respond to TMS.
- Genetic Markers: Some studies have investigated the role of genetic variations in predicting treatment response. For example, variations in genes related to serotonin or dopamine neurotransmission may influence treatment response.
- Neurotrophic Factors: Brain-derived neurotrophic factor (BDNF) and other neurotrophic factors play a role in neuronal growth and survival. Some studies have found that changes in BDNF levels are associated with treatment response.
While these biomarkers show promise, further research is needed to validate their predictive power and to develop clinically useful biomarker-based prediction models. Machine learning techniques can also be employed to integrate multiple biomarkers and improve prediction accuracy.
Many thanks to our sponsor Esdebe who helped us prepare this research report.
8. Future Directions
The field of TMS is rapidly evolving, with ongoing research focused on optimizing TMS protocols, expanding its applications, and personalizing treatment. Some of the key future directions in TMS research include:
- Optimizing Stimulation Protocols: Research is ongoing to optimize TMS protocols, including the intensity, frequency, duration, and location of stimulation. This includes development of adaptive protocols that change based on the individuals response.
- Combining TMS with Other Treatments: TMS is being investigated in combination with other treatments, such as medication, psychotherapy, and exercise. Combining TMS with other treatments may lead to synergistic effects and improved treatment outcomes.
- Developing New TMS Technologies: Researchers are developing new TMS technologies that offer improved focality, depth of stimulation, and patient comfort. Transcranial alternating current stimulation (tACS) and transcranial direct current stimulation (tDCS) are similar technologies which can be used to complement TMS.
- Expanding Applications: TMS is being explored as a treatment for a wider range of neuropsychiatric disorders, including autism spectrum disorder (ASD), attention-deficit/hyperactivity disorder (ADHD), and eating disorders. Investigating the use of TMS in healthy volunteers may help to explain more about its functionality.
- Personalizing TMS Therapy: Research is focused on identifying biomarkers that can predict individual treatment response and personalize TMS therapy. This includes developing algorithms that automatically adjust stimulation parameters based on the individual’s brain activity and clinical response.
- Understanding Mechanisms of Action: Further research is needed to fully understand the mechanisms by which TMS exerts its therapeutic effects. This includes investigating the role of synaptic plasticity, neurotransmitter release, neurogenesis, and brain network modulation.
Many thanks to our sponsor Esdebe who helped us prepare this research report.
9. Conclusion
Transcranial magnetic stimulation (TMS) has emerged as a valuable neuromodulation technique with established efficacy in treating MDD and OCD. Ongoing research is expanding its applications to other neuropsychiatric and neurological disorders. While TMS is generally safe and well-tolerated, careful patient selection and adherence to safety guidelines are essential. Future research should focus on optimizing TMS protocols, identifying biomarkers to predict treatment response, and personalizing TMS therapy to maximize its clinical benefits. The integration of TMS into existing healthcare systems and the exploration of its combination with other therapeutic modalities hold great promise for improving the lives of individuals with a wide range of neuropsychiatric conditions.
Many thanks to our sponsor Esdebe who helped us prepare this research report.
References
- American Psychiatric Association. (2013). Diagnostic and statistical manual of mental disorders (5th ed.).
- George, M. S., & Aston, S. L. (2023). Transcranial Magnetic Stimulation (TMS) for Depression. The American Journal of Psychiatry, 180(1), 12-14.
- Hallett, M. (2007). Transcranial magnetic stimulation: a primer. Neuron, 55(2), 187-199.
- Lefaucheur, J. P., et al. (2020). Evidence-based guidelines on the therapeutic use of repetitive transcranial magnetic stimulation (rTMS): An update. Clinical Neurophysiology, 131(2), 474-521.
- O’Reardon, J. P., et al. (2007). Efficacy and safety of transcranial magnetic stimulation in the acute treatment of major depression: a multisite randomized controlled trial. Biological Psychiatry, 62(11), 1208-1216.
- Rossi, S., et al. (2020). Safety, ethical considerations, and application guidelines for the use of transcranial magnetic stimulation in clinical practice and research. Clinical Neurophysiology, 131(11), 2837-2866.
- Tang, W. K., et al. (2020). Deep transcranial magnetic stimulation for treatment-resistant depression: a meta-analysis of randomized controlled trials. Journal of Affective Disorders, 260, 162-170.
- Van den Eynde, F., et al. (2010). Repetitive transcranial magnetic stimulation in obsessive-compulsive disorder: a systematic review. Journal of Clinical Psychiatry, 71(5), 574-585.
- Brunelin, O., et al. (2014). Efficacy and safety of low frequency repetitive transcranial magnetic stimulation (rTMS) of the right orbitofrontal cortex in obsessive-compulsive disorder: a randomized controlled trial. Brain Stimulation, 7(2), 249-257.
- Mantovani, A., et al. (2010). Repetitive transcranial magnetic stimulation (rTMS) in the treatment of obsessive-compulsive disorder: a systematic review and meta-analysis. Clinical Neurophysiology, 121(4), 420-429.
- Roth, Y., Zangen, A., & Hallett, M. (2009). Transcranial magnetic stimulation and deep transcranial magnetic stimulation: what is the difference?. Journal of Clinical Neurophysiology, 26(2), 89-96.
- Downar, J., & Daskalakis, Z. J. (2013). New targets for rTMS in depression: rationale and review of antidepressant response. Journal of Affective Disorders, 148(1), 19-29.
- Fitzgerald, P. B., Fountain, S., & Daskalakis, Z. J. (2006). A comprehensive review of the effects of rTMS on motor cortical excitability and inhibition. Clinical Neurophysiology, 117(12), 2584-2616.
So, if I understand correctly, with the right magnetic pulses I could potentially *unlearn* my habit of checking my smart home security cameras every 15 minutes? Asking for a friend… who is definitely not me.