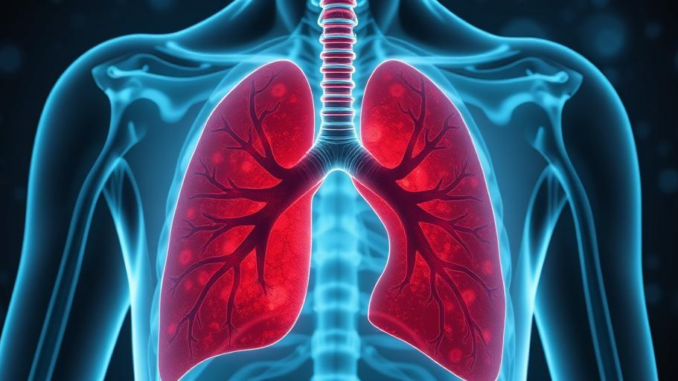
Abstract
Tuberculosis (TB), caused by Mycobacterium tuberculosis, remains a significant global health challenge despite a century of dedicated research and intervention efforts. This report provides a comprehensive overview of TB, encompassing the intricate interplay of host-pathogen interactions, the global burden and epidemiological trends, the alarming rise of drug-resistant strains, the evolution of diagnostic methodologies, and the promise of emerging therapeutic interventions. Furthermore, the report delves into the socio-economic factors that exacerbate the TB epidemic, highlighting the urgent need for integrated strategies that address both the biological and societal determinants of this disease. Special attention is given to the complex mechanisms underlying drug resistance, cutting edge approaches for rapid diagnostics, and the pipeline of novel drugs and vaccines that are being developed to combat this persistent threat. The report culminates with a discussion of the challenges that remain in achieving global TB control and eradication, emphasizing the need for continued research, increased funding, and strengthened global collaborations.
Many thanks to our sponsor Esdebe who helped us prepare this research report.
1. Introduction
Tuberculosis (TB), primarily caused by Mycobacterium tuberculosis (Mtb), is an infectious disease that predominantly affects the lungs but can also disseminate to other organs. Despite significant advancements in diagnosis and treatment, TB remains a major global health burden, particularly in low- and middle-income countries (LMICs). The World Health Organization (WHO) estimates that approximately 10 million people fell ill with TB in 2022, and 1.3 million died from the disease [1]. The emergence and spread of drug-resistant strains of Mtb, coupled with co-infection with HIV, pose significant challenges to TB control efforts. This report aims to provide a comprehensive overview of TB, examining its pathogenesis, global epidemiology, drug resistance mechanisms, diagnostic innovations, and novel therapeutic strategies. Furthermore, the report will explore the socio-economic factors that contribute to the TB epidemic and highlight the ongoing research efforts aimed at developing more effective prevention and treatment strategies.
Many thanks to our sponsor Esdebe who helped us prepare this research report.
2. Pathogenesis of Mycobacterium tuberculosis Infection
The pathogenesis of Mtb infection is a complex process involving intricate interactions between the pathogen and the host immune system. Mtb is typically transmitted via airborne droplets containing the bacteria. Upon inhalation, the bacteria reach the alveoli of the lungs, where they are phagocytosed by alveolar macrophages. The ability of Mtb to survive and replicate within macrophages is crucial for establishing infection. Several virulence factors contribute to this intracellular survival, including the bacterial cell wall components lipoarabinomannan (LAM) and mycolic acids [2]. These components interfere with macrophage activation and inhibit phagosome maturation, preventing the fusion of lysosomes with the phagosome, which is essential for bacterial killing.
Once inside macrophages, Mtb replicates, eventually leading to macrophage lysis and the release of bacteria into the surrounding tissue. This triggers an inflammatory response, recruiting additional immune cells, including monocytes, dendritic cells, and T lymphocytes, to the site of infection. The accumulation of these immune cells leads to the formation of a granuloma, a characteristic pathological hallmark of TB. The granuloma serves to contain the infection, preventing the spread of bacteria to other parts of the body. Within the granuloma, Mtb can persist in a dormant or latent state for many years. The host’s immune system plays a crucial role in maintaining this latent infection. However, if the immune system becomes compromised, for example, due to HIV infection, malnutrition, or immunosuppressive therapy, Mtb can reactivate, leading to active TB disease.
The reactivation process involves the breakdown of the granuloma and the release of bacteria into the lungs, resulting in the characteristic symptoms of TB, such as cough, fever, weight loss, and night sweats. In some cases, Mtb can disseminate from the lungs to other organs, leading to extrapulmonary TB, which can affect the lymph nodes, bones, meninges, and other tissues.
The ability of Mtb to evade the host immune system and establish latent infection is a major obstacle to TB control. Understanding the mechanisms that regulate latency and reactivation is crucial for developing new strategies to prevent TB disease.
Many thanks to our sponsor Esdebe who helped us prepare this research report.
3. Global Epidemiology and Trends
TB remains a significant global health problem, with the highest burden in LMICs. The WHO estimates that the global incidence of TB is approximately 130 cases per 100,000 population [1]. The regions with the highest TB burden are Africa and Southeast Asia, which account for more than half of the global TB cases. India, Indonesia, China, the Philippines, Pakistan, Nigeria, Bangladesh and South Africa are among the countries with the highest number of TB cases.
The TB epidemic is closely linked to poverty, malnutrition, and poor living conditions. Overcrowding, inadequate ventilation, and limited access to healthcare services contribute to the transmission and spread of Mtb. In addition, co-infection with HIV significantly increases the risk of developing active TB disease. HIV weakens the immune system, making individuals more susceptible to Mtb infection and reactivation. The WHO estimates that approximately 8% of TB cases are co-infected with HIV [1].
Despite significant progress in TB control over the past few decades, the decline in TB incidence has slowed in recent years. This is partly due to the emergence and spread of drug-resistant strains of Mtb and the challenges in diagnosing and treating these strains. The COVID-19 pandemic has also had a negative impact on TB control efforts, with disruptions in TB diagnosis and treatment services leading to an increase in TB cases and deaths [3].
Addressing the TB epidemic requires a multi-pronged approach that includes improving living conditions, increasing access to healthcare services, strengthening TB control programs, and developing new diagnostic and therapeutic tools. Furthermore, it is crucial to address the social and economic factors that contribute to the TB epidemic, such as poverty, malnutrition, and discrimination.
Many thanks to our sponsor Esdebe who helped us prepare this research report.
4. Drug-Resistant Tuberculosis
The emergence and spread of drug-resistant strains of Mtb is a major threat to TB control. Drug-resistant TB is defined as TB that is resistant to at least one anti-TB drug. Multidrug-resistant TB (MDR-TB) is defined as TB that is resistant to at least isoniazid and rifampicin, the two most potent first-line anti-TB drugs. Extensively drug-resistant TB (XDR-TB) is defined as MDR-TB that is also resistant to any fluoroquinolone and at least one injectable second-line anti-TB drug (amikacin, kanamycin, or capreomycin).
Drug resistance in Mtb arises primarily through the acquisition of mutations in genes that encode drug targets or genes involved in drug metabolism. These mutations can occur spontaneously during bacterial replication or can be acquired through horizontal gene transfer. The use of inadequate or incomplete TB treatment regimens promotes the selection and spread of drug-resistant strains of Mtb.
The treatment of drug-resistant TB is more complex, expensive, and prolonged than the treatment of drug-susceptible TB. Second-line anti-TB drugs are less effective, more toxic, and require longer treatment durations. The treatment success rates for MDR-TB and XDR-TB are significantly lower than those for drug-susceptible TB.
Addressing the challenge of drug-resistant TB requires a comprehensive approach that includes strengthening TB control programs, improving TB diagnostic capacity, ensuring access to quality-assured anti-TB drugs, and promoting adherence to treatment regimens. Furthermore, it is crucial to develop new drugs and treatment regimens for drug-resistant TB. The development of shorter, less toxic, and more effective treatment regimens for drug-resistant TB is a major priority.
4.1 Mechanisms of Drug Resistance
The mechanisms of drug resistance in Mtb are diverse and often involve multiple genetic mutations. Understanding these mechanisms is crucial for developing new drugs that can overcome resistance. Some of the key mechanisms of drug resistance are outlined below:
-
Rifampicin: Resistance to rifampicin is primarily due to mutations in the rpoB gene, which encodes the beta subunit of RNA polymerase. These mutations alter the structure of RNA polymerase, preventing rifampicin from binding and inhibiting its activity [4].
-
Isoniazid: Resistance to isoniazid is more complex and can involve mutations in several genes, including katG, inhA, and kasA. katG encodes catalase-peroxidase, an enzyme that activates isoniazid. Mutations in katG can reduce or abolish the activation of isoniazid, rendering the drug ineffective. inhA encodes enoyl-acyl carrier protein reductase, which is involved in mycolic acid biosynthesis. Mutations in inhA can increase the expression of the enzyme, making it less susceptible to inhibition by isoniazid. kasA encodes beta-ketoacyl-ACP synthase, another enzyme involved in mycolic acid biosynthesis. Mutations in kasA can also confer resistance to isoniazid [5].
-
Fluoroquinolones: Resistance to fluoroquinolones is primarily due to mutations in the gyrA and gyrB genes, which encode DNA gyrase, an enzyme essential for DNA replication. These mutations alter the structure of DNA gyrase, preventing fluoroquinolones from binding and inhibiting its activity [6].
-
Aminoglycosides: Resistance to aminoglycosides is primarily due to mutations in the rrs gene, which encodes 16S rRNA, a component of the ribosome. These mutations alter the structure of the ribosome, preventing aminoglycosides from binding and inhibiting protein synthesis [7]. Additionally, aminoglycoside resistance can be mediated by aminoglycoside modifying enzymes (AMEs) that inactivate the drug via acetylation, phosphorylation, or adenylation.
-
Ethambutol: Resistance to ethambutol is primarily due to mutations in the embB gene, which encodes arabinosyl transferase, an enzyme involved in arabinan biosynthesis. These mutations alter the structure of arabinosyl transferase, reducing its affinity for ethambutol [8].
The accumulation of multiple drug resistance mutations in Mtb results in multidrug-resistant (MDR) and extensively drug-resistant (XDR) strains. Understanding the specific mutations and their impact on drug efficacy is crucial for developing new diagnostic tools and treatment strategies.
Many thanks to our sponsor Esdebe who helped us prepare this research report.
5. Diagnostic Innovations
Early and accurate diagnosis of TB is essential for effective treatment and prevention of transmission. Traditional diagnostic methods, such as sputum smear microscopy and culture, have limitations in terms of sensitivity and turnaround time. Sputum smear microscopy has low sensitivity, particularly in patients with HIV co-infection or extrapulmonary TB. Culture is more sensitive but requires several weeks to obtain results.
In recent years, significant advances have been made in TB diagnostics, including the development of rapid molecular tests. The Xpert MTB/RIF assay, a nucleic acid amplification test (NAAT), is a rapid and sensitive test that can detect Mtb DNA and rifampicin resistance mutations in sputum samples within hours [9]. The Xpert MTB/RIF assay has been widely implemented in LMICs and has significantly improved TB diagnosis and treatment initiation. Newer versions of the Xpert assay, such as Xpert MTB/XDR, can detect resistance to additional anti-TB drugs, including isoniazid and fluoroquinolones.
Other molecular tests, such as line probe assays (LPAs), can also detect drug resistance mutations in Mtb. LPAs are particularly useful for detecting isoniazid resistance mutations. Furthermore, whole-genome sequencing (WGS) is increasingly being used for TB diagnostics and surveillance. WGS can provide comprehensive information about the Mtb strain, including its drug resistance profile and evolutionary history [10]. However, WGS is still relatively expensive and requires specialized expertise, limiting its widespread implementation in LMICs.
In addition to molecular tests, there is also ongoing research to develop new biomarkers for TB diagnosis. These biomarkers could be used to develop point-of-care tests that can be performed in resource-limited settings. Potential biomarkers include host immune responses, such as cytokines and antibodies, as well as bacterial metabolites.
Many thanks to our sponsor Esdebe who helped us prepare this research report.
6. Novel Therapeutic Strategies
The current TB treatment regimen, consisting of isoniazid, rifampicin, pyrazinamide, and ethambutol for 6 months, is effective for drug-susceptible TB. However, the long duration of treatment and the potential for adverse drug reactions can lead to poor adherence and treatment failure. Furthermore, the treatment of drug-resistant TB requires longer, more complex, and more toxic regimens. Therefore, there is an urgent need to develop new drugs and treatment regimens for TB.
Several new anti-TB drugs have been developed in recent years, including bedaquiline, delamanid, and pretomanid. Bedaquiline is a diarylquinoline that inhibits ATP synthase, an enzyme essential for bacterial energy production [11]. Delamanid is a nitroimidazole that inhibits mycolic acid biosynthesis [12]. Pretomanid is also a nitroimidazole that inhibits both mycolic acid biosynthesis and respiratory metabolism [13]. These new drugs have been shown to be effective against drug-resistant TB and have been incorporated into shorter, all-oral regimens for MDR-TB and XDR-TB. The Nix-TB trial, which evaluated a 6-month regimen of bedaquiline, pretomanid, and linezolid (BPaL) for XDR-TB and treatment-intolerant or non-responsive MDR-TB, reported high success rates [14].
In addition to new drugs, there is also ongoing research to develop new treatment strategies, such as host-directed therapies. Host-directed therapies aim to enhance the host immune response to Mtb, thereby improving treatment outcomes. Examples of host-directed therapies include immunomodulators, such as vitamin D and interferon-gamma, as well as inhibitors of host cell signaling pathways.
Furthermore, there is ongoing research to develop new TB vaccines. The current TB vaccine, Bacillus Calmette-Guérin (BCG), provides limited protection against pulmonary TB in adults. Several new TB vaccine candidates are in clinical development, including subunit vaccines, viral-vectored vaccines, and whole-cell vaccines. These vaccines aim to provide better protection against TB disease and to prevent Mtb infection.
6.1 Drug Repurposing
Drug repurposing, also known as drug repositioning, involves identifying new uses for existing drugs. This approach can accelerate the development of new TB treatments by leveraging the existing safety and efficacy data of approved drugs. Several drugs approved for other indications have shown promising activity against Mtb in vitro and in vivo. Examples include clofazimine, linezolid, and macrolides. These drugs have been incorporated into treatment regimens for drug-resistant TB. However, further research is needed to identify additional drugs that can be repurposed for TB treatment.
Many thanks to our sponsor Esdebe who helped us prepare this research report.
7. Socio-Economic Impact of Tuberculosis
TB has a significant socio-economic impact, particularly in LMICs. TB disproportionately affects individuals living in poverty, who are more likely to be exposed to Mtb and to have compromised immune systems. TB can lead to lost productivity, reduced income, and increased healthcare costs. The WHO estimates that TB costs the global economy billions of dollars each year [1].
TB can also perpetuate poverty by reducing household income and increasing household debt. Families affected by TB may face stigma and discrimination, further exacerbating their socio-economic vulnerability. Children of TB patients are at increased risk of malnutrition and impaired development.
Addressing the socio-economic impact of TB requires a comprehensive approach that includes poverty reduction, improved living conditions, and increased access to healthcare services. Furthermore, it is crucial to address the social and economic determinants of TB, such as malnutrition, lack of education, and gender inequality. Social protection programs, such as cash transfers and food subsidies, can help to mitigate the socio-economic impact of TB on affected families. Furthermore, efforts to reduce stigma and discrimination associated with TB are essential for promoting TB control and improving the quality of life of TB patients. In some regions, targeted screening programmes of groups who are at high risk, such as migrant workers, prisoners, and people in contact with active TB cases, can be effective in reducing the TB burden.
Many thanks to our sponsor Esdebe who helped us prepare this research report.
8. Challenges and Future Directions
Despite significant progress in TB control, several challenges remain in achieving global TB control and eradication. These challenges include the emergence and spread of drug-resistant TB, the high burden of TB/HIV co-infection, the slow decline in TB incidence, the limitations of current diagnostic tools and treatment regimens, and the lack of a highly effective TB vaccine.
Addressing these challenges requires a concerted effort by researchers, policymakers, healthcare providers, and communities. Key priorities include:
-
Strengthening TB control programs: This includes improving TB surveillance, diagnosis, treatment, and prevention services.
-
Developing new diagnostic tools: This includes developing rapid, sensitive, and affordable point-of-care tests for TB and drug resistance.
-
Developing new drugs and treatment regimens: This includes developing shorter, less toxic, and more effective treatment regimens for drug-susceptible and drug-resistant TB.
-
Developing a highly effective TB vaccine: This includes developing vaccines that can prevent Mtb infection and TB disease.
-
Addressing the socio-economic determinants of TB: This includes poverty reduction, improved living conditions, and increased access to healthcare services.
-
Strengthening global collaborations: This includes sharing data, resources, and expertise to accelerate TB research and control efforts.
-
Combating anti-microbial resistance by improving diagnostic stewardship, and promoting rational use of antibiotics.
-
Implementing digital health technologies to improve TB care and monitoring.
Ultimately, achieving global TB control and eradication requires a multi-faceted approach that addresses both the biological and societal determinants of the disease. Continued research, increased funding, and strengthened global collaborations are essential for overcoming the challenges and achieving the goal of a TB-free world.
Many thanks to our sponsor Esdebe who helped us prepare this research report.
9. Conclusion
Tuberculosis remains a formidable global health challenge, necessitating a comprehensive and multifaceted approach. This report has highlighted the intricate interplay of host-pathogen interactions, the global burden and epidemiological trends, the alarming rise of drug-resistant strains, the evolution of diagnostic methodologies, and the promise of emerging therapeutic interventions. The socio-economic factors that exacerbate the TB epidemic necessitate integrated strategies that address both the biological and societal determinants of this disease. Continuous investment in research and development is vital for the advancement of diagnostic tools, therapeutic strategies, and preventive measures. Furthermore, sustainable global collaboration is essential to combat TB effectively and achieve the goal of a TB-free world.
Many thanks to our sponsor Esdebe who helped us prepare this research report.
References
[1] World Health Organization. Global Tuberculosis Report 2023. Geneva, Switzerland; 2023.
[2] Chatterjee D, Khoo KH. Mycobacterial lipoarabinomannan: an extraordinary glycolipid with profound immunomodulatory properties. Glycobiology. 1998;8(2):113-20.
[3] Hogan AB, Baker BM, Metcalf CJ, et al. The potential impact of the COVID-19 pandemic on global tuberculosis incidence and mortality. Elife. 2020;9:e59782.
[4] Ramaswamy S, Musser JM. Molecular genetic basis of antimicrobial agent resistance in Mycobacterium tuberculosis: 1998 update. Tuber Lung Dis. 1998;79(1):3-29.
[5] Campbell PJ, Morlock GP, Li R, et al. Heteroresistance to isoniazid in Mycobacterium tuberculosis. J Infect Dis. 2011;204(4):513-21.
[6] Malik S, Godfrey AJ, Weyer J, et al. Molecular mechanisms of fluoroquinolone resistance in Mycobacterium tuberculosis isolates from South Africa. Antimicrob Agents Chemother. 2008;52(7):2518-24.
[7] Sandgren A, Strong M, Muthukrishnan P, et al. Effect of rrs and eis mutations on Mycobacterium tuberculosis susceptibility to kanamycin and amikacin. Antimicrob Agents Chemother. 2009;53(5):2226-9.
[8] Somoskovi A, Parsons LM, Salfinger M. The molecular basis of resistance to isoniazid, rifampin, and ethambutol in Mycobacterium tuberculosis. Respir Res. 2001;2(3):164-8.
[9] Boehme CC, Nabeta P, Hillemann D, et al. Rapid molecular detection of tuberculosis and rifampicin resistance. N Engl J Med. 2010;363(11):1005-15.
[10] Walker TM, Kohl TA, Omar SV, et al. Whole-genome sequencing for prediction of Mycobacterium tuberculosis drug susceptibility and resistance: a retrospective cohort study. Lancet Infect Dis. 2015;15(10):1193-202.
[11] Andries K, Verhasselt P, Guillemont J, et al. A diarylquinoline drug active on the ATP synthase of Mycobacterium tuberculosis. Science. 2005;307(5707):223-7.
[12] Gler MT, Skripconoka V, Sanchez-Garavito E, et al. Delamanid for multidrug-resistant pulmonary tuberculosis. N Engl J Med. 2012;366(23):2151-60.
[13] Stenerløw B, Agrenius V, Lebina J, et al. A novel nitroimidazo-oxazole, PA-824, is effective in a murine model of tuberculosis. Antimicrob Agents Chemother. 2006;50(6):2293-6.
[14] Conradie F, Diacon AH, Ngubane N, et al. Bedaquiline, Pretomanid, and Linezolid Regimen for Drug-Resistant Tuberculosis. N Engl J Med. 2020;382(10):893-902.
Wow, that’s a lot to unpack! I didn’t realize TB had so many sponsors listed; maybe they should offer a frequent flyer program? You know, “Cough up a lung, earn a free lung!” I am kidding of course – very important work but needs funding.
Thanks for your comment! It’s definitely a complex topic, and funding is crucial for TB research and control. A frequent flyer program is an interesting thought! Perhaps sponsors could consider innovative ways to incentivize research participation or support programs that address the socio-economic factors contributing to TB.
Editor: MedTechNews.Uk
Thank you to our Sponsor Esdebe
The discussion on socio-economic factors is critical. Addressing issues like poverty and malnutrition alongside medical interventions could significantly improve TB control and prevention, especially in high-burden regions.