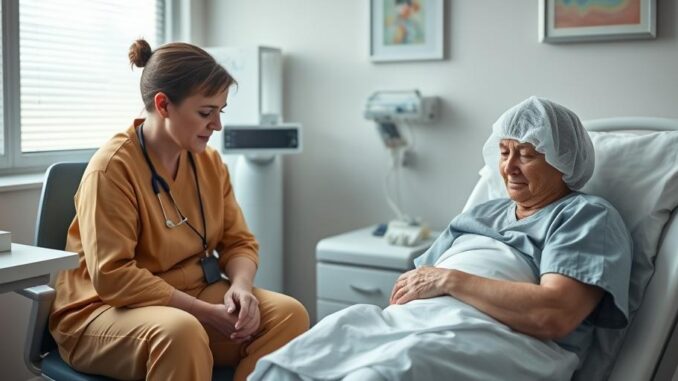
Abstract
Carbamoyl phosphate synthetase 1 (CPS1) deficiency, a rare autosomal recessive disorder, disrupts the urea cycle and leads to hyperammonemia. This report provides a comprehensive overview of CPS1 deficiency, encompassing its genetic underpinnings, the critical role of CPS1 in ammonia detoxification, the intricate pathophysiology of hyperammonemia, current diagnostic and treatment modalities, and promising emerging therapies. We delve into the molecular mechanisms driving CPS1 deficiency, exploring the impact of various mutations on enzyme structure and function. Furthermore, we scrutinize the challenges associated with long-term management, including the prevention of acute hyperammonemic crises and the mitigation of chronic neurological sequelae. Finally, we critically evaluate the potential of gene therapy and mRNA-based treatments to revolutionize the therapeutic landscape of CPS1 deficiency, offering a glimpse into the future of personalized medicine for this debilitating condition.
Many thanks to our sponsor Esdebe who helped us prepare this research report.
1. Introduction
Disorders of the urea cycle (UCDs) represent a group of inherited metabolic diseases characterized by a deficiency in one of the six enzymes or two transporters that constitute the urea cycle. This cycle, predominantly active in the liver, is essential for the detoxification of ammonia, a neurotoxic byproduct of protein catabolism. Among these disorders, carbamoyl phosphate synthetase 1 (CPS1) deficiency, caused by mutations in the CPS1 gene, stands out as one of the most severe. Ammonia accumulation in the blood (hyperammonemia) leads to a cascade of detrimental effects, primarily affecting the central nervous system. Clinically, CPS1 deficiency can manifest with varying degrees of severity, ranging from neonatal onset with life-threatening hyperammonemic coma to late-onset presentations with subtle neurological symptoms. The rarity of CPS1 deficiency poses significant challenges for diagnosis, treatment, and long-term management. Furthermore, the phenotypic heterogeneity and the complexity of ammonia metabolism necessitate a multidisciplinary approach to patient care. This report aims to provide an in-depth analysis of CPS1 deficiency, highlighting the critical role of CPS1 in ammonia detoxification, the molecular basis of the disease, the available diagnostic and therapeutic strategies, and the potential of emerging therapies to improve patient outcomes.
Many thanks to our sponsor Esdebe who helped us prepare this research report.
2. Genetic Basis of CPS1 Deficiency
The CPS1 gene, located on chromosome 2q35, encodes the mitochondrial enzyme carbamoyl phosphate synthetase 1 (CPS1). This enzyme catalyzes the first committed step of the urea cycle, converting ammonia, bicarbonate, and two molecules of ATP into carbamoyl phosphate [1]. CPS1 is a large, complex protein with multiple domains, including an N-terminal domain involved in substrate binding, a central domain responsible for ATP hydrolysis, and a C-terminal domain that interacts with N-acetylglutamate (NAG), an essential allosteric activator [2].
CPS1 deficiency is inherited in an autosomal recessive manner, meaning that affected individuals must inherit two copies of a mutated CPS1 gene, one from each parent. Hundreds of different mutations in the CPS1 gene have been identified in patients with CPS1 deficiency, reflecting the genetic heterogeneity of this disorder [3]. These mutations are scattered throughout the gene and include missense mutations, nonsense mutations, frameshift mutations, and splice-site mutations. The type and location of the mutation can significantly impact the severity of the enzyme deficiency and the clinical phenotype. For example, mutations that disrupt critical domains or active sites of the enzyme are more likely to result in severe enzyme deficiency and early-onset disease. Conversely, some missense mutations may lead to milder enzyme deficiencies and late-onset presentations.
Genotype-phenotype correlations in CPS1 deficiency are often complex and not always straightforward. While some mutations are consistently associated with severe or mild phenotypes, others exhibit considerable variability. This variability may be attributed to several factors, including the presence of modifier genes, epigenetic modifications, and environmental influences. Furthermore, the functional consequences of some CPS1 mutations remain unclear, making it difficult to predict the clinical outcome. Detailed in vitro studies of mutant CPS1 enzymes are crucial to elucidate the impact of specific mutations on enzyme activity, stability, and allosteric regulation. Next-generation sequencing technologies, such as whole-exome sequencing and whole-genome sequencing, have greatly facilitated the identification of novel CPS1 mutations and the characterization of genotype-phenotype relationships. However, further research is needed to fully understand the complex interplay between genotype and phenotype in CPS1 deficiency.
Many thanks to our sponsor Esdebe who helped us prepare this research report.
3. Role of Carbamoyl Phosphate Synthetase 1 in Ammonia Detoxification
CPS1 plays a pivotal role in the urea cycle, serving as the rate-limiting enzyme in the pathway. This enzyme catalyzes the ATP-dependent synthesis of carbamoyl phosphate from ammonia, bicarbonate, and two molecules of ATP. N-acetylglutamate (NAG), synthesized by N-acetylglutamate synthase (NAGS), is an essential allosteric activator of CPS1. NAG binds to CPS1 and induces a conformational change that enhances the enzyme’s affinity for its substrates [4].
Carbamoyl phosphate then enters the urea cycle, where it reacts with ornithine to form citrulline, catalyzed by ornithine transcarbamoylase (OTC). The subsequent steps of the urea cycle involve the sequential addition of aspartate, cleavage of argininosuccinate into arginine and fumarate, and hydrolysis of arginine to regenerate ornithine and produce urea, which is ultimately excreted in the urine. In individuals with CPS1 deficiency, the impairment of carbamoyl phosphate synthesis leads to a buildup of ammonia in the blood. Ammonia is a potent neurotoxin that can cross the blood-brain barrier and disrupt brain function. The mechanisms by which ammonia exerts its neurotoxic effects are complex and multifactorial. Ammonia can interfere with neurotransmitter metabolism, disrupt energy production in the brain, and promote oxidative stress and inflammation [5]. Elevated ammonia levels can also alter astrocyte function, leading to cerebral edema and increased intracranial pressure. Furthermore, chronic hyperammonemia can lead to irreversible brain damage and neurological deficits, including intellectual disability, seizures, and motor dysfunction.
The liver is the primary site of ammonia detoxification via the urea cycle. However, other tissues, such as the brain and kidneys, also contribute to ammonia metabolism, albeit to a lesser extent. In the brain, ammonia can be detoxified by glutamine synthetase, which converts glutamate and ammonia into glutamine. However, the capacity of glutamine synthetase to detoxify ammonia is limited, and in the setting of hyperammonemia, glutamine accumulates in the brain, contributing to cerebral edema. The kidneys can also excrete ammonia in the urine, but this process is insufficient to compensate for the impaired urea cycle in CPS1 deficiency. Therefore, the primary focus of treatment for CPS1 deficiency is to reduce ammonia production and enhance ammonia excretion through alternative pathways.
Many thanks to our sponsor Esdebe who helped us prepare this research report.
4. Pathophysiology of Hyperammonemia in CPS1 Deficiency
The pathophysiology of hyperammonemia in CPS1 deficiency is complex and involves multiple organ systems. The primary defect is the impaired synthesis of carbamoyl phosphate, which leads to a buildup of ammonia in the blood. However, the severity of hyperammonemia and the clinical manifestations of CPS1 deficiency are influenced by several factors, including the degree of enzyme deficiency, dietary protein intake, catabolic stress, and the presence of intercurrent illnesses.
In newborns with severe CPS1 deficiency, hyperammonemia can develop rapidly within the first few days of life. This is due to the immature hepatic function and the high protein intake associated with breastfeeding or formula feeding. The liver’s limited capacity to detoxify ammonia overwhelms the residual urea cycle activity, leading to a rapid rise in ammonia levels. Acute hyperammonemic crises are characterized by lethargy, poor feeding, vomiting, seizures, and coma. If left untreated, these crises can result in irreversible brain damage and death.
In individuals with milder CPS1 deficiency, hyperammonemia may be triggered by catabolic stress, such as infections, surgery, or prolonged fasting. During catabolism, protein breakdown increases, leading to an increased production of ammonia. The impaired urea cycle in CPS1 deficiency is unable to efficiently detoxify the excess ammonia, resulting in hyperammonemia. Chronic hyperammonemia, even at relatively low levels, can have detrimental effects on the brain. Studies have shown that chronic hyperammonemia can impair cognitive function, motor skills, and behavior. It can also lead to the development of neurological complications, such as intellectual disability, seizures, and ataxia.
Furthermore, hyperammonemia can disrupt the balance of neurotransmitters in the brain. Ammonia can inhibit the reuptake of glutamate, leading to an accumulation of glutamate in the synaptic cleft. Excess glutamate can overstimulate glutamate receptors, causing excitotoxicity and neuronal damage [6]. Hyperammonemia can also interfere with the synthesis and degradation of GABA, an inhibitory neurotransmitter. Impaired GABAergic neurotransmission can contribute to seizures and other neurological symptoms.
In addition to its direct effects on the brain, hyperammonemia can also affect other organ systems. Elevated ammonia levels can cause gastrointestinal distress, leading to nausea, vomiting, and abdominal pain. Hyperammonemia can also impair renal function and contribute to electrolyte imbalances. In severe cases, hyperammonemia can lead to multi-organ failure and death.
Many thanks to our sponsor Esdebe who helped us prepare this research report.
5. Diagnostic Methods
The diagnosis of CPS1 deficiency typically involves a combination of clinical evaluation, biochemical testing, and genetic analysis. A high index of suspicion is crucial, particularly in newborns presenting with unexplained hyperammonemia. Clinical features that should raise suspicion for CPS1 deficiency include lethargy, poor feeding, vomiting, seizures, and coma, especially in the context of a family history of UCDs or unexplained infant deaths.
Biochemical testing plays a critical role in the diagnosis of CPS1 deficiency. The most important biochemical test is the measurement of plasma ammonia levels. Elevated plasma ammonia levels, particularly in the absence of other obvious causes, such as liver disease or renal failure, should prompt further investigation. In addition to ammonia levels, other biochemical tests that can be helpful include plasma amino acid analysis and urine organic acid analysis. In CPS1 deficiency, plasma glutamine levels are typically elevated, while plasma citrulline levels are low or undetectable. Urine organic acid analysis may reveal elevated levels of orotic acid, although this finding is not specific to CPS1 deficiency.
Genetic testing is essential for confirming the diagnosis of CPS1 deficiency and for identifying the specific mutations in the CPS1 gene. Genetic testing can be performed on DNA extracted from blood, saliva, or other tissues. Sanger sequencing of the CPS1 gene was previously the gold standard for genetic diagnosis. However, next-generation sequencing technologies, such as whole-exome sequencing and whole-genome sequencing, have become increasingly common and offer several advantages over Sanger sequencing, including the ability to screen for mutations in multiple genes simultaneously and to detect rare or novel mutations.
In some cases, liver biopsy may be considered to measure CPS1 enzyme activity directly. However, liver biopsy is an invasive procedure and is typically reserved for cases in which genetic testing is inconclusive or when other liver disorders need to be ruled out. Fibroblast studies can also be used to measure CPS1 enzyme activity, but these studies are less reliable than liver biopsy.
Newborn screening for UCDs, including CPS1 deficiency, has been implemented in many countries. Newborn screening typically involves measuring plasma glutamine levels or citrulline levels in dried blood spots. If the initial screening test is positive, confirmatory biochemical and genetic testing is performed. Newborn screening can lead to earlier diagnosis and treatment of CPS1 deficiency, which can improve patient outcomes.
Many thanks to our sponsor Esdebe who helped us prepare this research report.
6. Current Treatment Strategies
The treatment of CPS1 deficiency is aimed at reducing ammonia production, enhancing ammonia excretion, and preventing or managing hyperammonemic crises. Current treatment strategies include dietary management, ammonia-scavenging medications, and liver transplantation.
Dietary Management: Dietary management is a cornerstone of treatment for CPS1 deficiency. The goal of dietary management is to restrict protein intake to the minimum level necessary to support growth and development while minimizing ammonia production. The specific protein intake recommendations vary depending on the age and severity of the enzyme deficiency. Infants with severe CPS1 deficiency typically require a protein-restricted diet supplemented with essential amino acids to ensure adequate growth. Older children and adults may also benefit from a protein-restricted diet, but the level of restriction may be less stringent. In addition to protein restriction, dietary management also includes frequent feedings to prevent catabolism and the provision of adequate calories to support growth. Specialized formulas and medical foods are available that are low in protein and enriched with essential amino acids and calories.
Ammonia-Scavenging Medications: Ammonia-scavenging medications are used to enhance ammonia excretion through alternative pathways. The two most commonly used ammonia-scavenging medications are sodium benzoate and sodium phenylbutyrate. Sodium benzoate conjugates with glycine to form hippurate, which is excreted in the urine, thereby removing ammonia. Sodium phenylbutyrate is converted to phenylacetate, which conjugates with glutamine to form phenylacetylglutamine, which is also excreted in the urine. These medications can effectively lower ammonia levels in patients with CPS1 deficiency, but they do not correct the underlying enzyme deficiency.
Liver Transplantation: Liver transplantation is a curative therapy for CPS1 deficiency. Liver transplantation provides a source of functional CPS1 enzyme, allowing for normal ammonia detoxification. Liver transplantation is typically considered for patients with severe CPS1 deficiency who are not responding adequately to dietary management and ammonia-scavenging medications. Liver transplantation can significantly improve patient outcomes and quality of life. However, it is associated with significant risks, including rejection, infection, and complications from immunosuppression. The decision to pursue liver transplantation should be made on a case-by-case basis, taking into account the severity of the disease, the patient’s overall health, and the availability of a suitable donor.
Emergency Management of Hyperammonemic Crises: Prompt and aggressive management of hyperammonemic crises is essential to prevent irreversible brain damage. The management of hyperammonemic crises includes the following measures: stopping protein intake, providing intravenous fluids with dextrose to prevent catabolism, administering ammonia-scavenging medications (sodium benzoate and sodium phenylbutyrate), and considering hemodialysis or continuous venovenous hemofiltration (CVVH) to rapidly remove ammonia from the blood. Hemodialysis or CVVH is typically reserved for patients with severe hyperammonemia who are not responding to other treatments.
Many thanks to our sponsor Esdebe who helped us prepare this research report.
7. Emerging Therapies
Despite the advances in treatment for CPS1 deficiency, there is still a need for more effective and less burdensome therapies. Emerging therapies, such as gene therapy and mRNA-based treatments, hold promise for revolutionizing the treatment of CPS1 deficiency.
Gene Therapy: Gene therapy involves the introduction of a functional copy of the CPS1 gene into the patient’s liver cells. This can be achieved using viral vectors, such as adeno-associated viruses (AAVs), to deliver the CPS1 gene to the liver. AAV vectors have several advantages for gene therapy, including their ability to transduce liver cells efficiently, their low immunogenicity, and their long-term expression of the transgene. Several preclinical studies have demonstrated the efficacy of AAV-mediated gene therapy in animal models of CPS1 deficiency [7]. These studies have shown that gene therapy can restore CPS1 enzyme activity, lower ammonia levels, and improve survival. Clinical trials of gene therapy for CPS1 deficiency are currently underway. While showing promise, gene therapy still faces challenges such as achieving sustained expression of the transgene and preventing immune responses against the viral vector.
mRNA-Based Treatments: mRNA-based treatments involve the administration of messenger RNA (mRNA) encoding the CPS1 enzyme. The mRNA is taken up by the liver cells, where it is translated into functional CPS1 enzyme. mRNA-based treatments offer several advantages over gene therapy, including their ease of manufacturing, their transient expression, and their lack of integration into the host genome. Preclinical studies have shown that mRNA-based treatments can effectively restore CPS1 enzyme activity and lower ammonia levels in animal models of CPS1 deficiency [8]. Clinical trials of mRNA-based treatments for CPS1 deficiency are also in development. However, challenges remain such as optimizing mRNA delivery to the liver and ensuring that sufficient protein expression is achieved.
Chaperone Therapy: Another potential therapeutic approach is chaperone therapy, which involves the use of small molecules that can stabilize mutant CPS1 enzymes and enhance their activity. Some CPS1 mutations result in misfolded or unstable enzymes that are rapidly degraded. Chaperone molecules can bind to these mutant enzymes and help them to fold correctly, thereby increasing their stability and activity. This approach is currently theoretical in the context of CPS1 deficiency, however, it may be possible to identify chaperone molecules that can specifically target mutant CPS1 enzymes and improve their function.
CRISPR-Cas9 Gene Editing: CRISPR-Cas9 gene editing is a revolutionary technology that allows for precise modification of the genome. In the context of CPS1 deficiency, CRISPR-Cas9 could be used to correct the mutations in the CPS1 gene directly. While still in the early stages of development, CRISPR-Cas9 holds tremendous potential for treating genetic disorders like CPS1 deficiency, offering the possibility of a permanent cure. However, significant challenges remain, including ensuring the safety and efficacy of CRISPR-Cas9 gene editing and delivering the gene-editing machinery to the liver cells with high precision.
Many thanks to our sponsor Esdebe who helped us prepare this research report.
8. Long-Term Management and Potential Complications
Long-term management of patients with CPS1 deficiency is essential to prevent hyperammonemic crises and minimize neurological sequelae. This requires a multidisciplinary approach involving physicians, dietitians, and other healthcare professionals. Regular monitoring of plasma ammonia levels, plasma amino acid levels, and urine organic acid levels is crucial to assess the effectiveness of treatment and to adjust dietary and medication regimens as needed.
Patients with CPS1 deficiency are at risk for several potential complications, including neurological damage, intellectual disability, seizures, motor dysfunction, and growth retardation. Early diagnosis and treatment are essential to minimize the risk of these complications. In addition to medical management, patients with CPS1 deficiency may benefit from developmental therapies, such as physical therapy, occupational therapy, and speech therapy, to improve their cognitive and motor skills.
Adherence to dietary restrictions and medication regimens can be challenging for patients with CPS1 deficiency, particularly during adolescence. Patient education and support are essential to promote adherence and to empower patients to manage their condition effectively. Family support is also crucial, as parents and siblings play a vital role in providing care and support.
The long-term prognosis for patients with CPS1 deficiency varies depending on the severity of the enzyme deficiency, the age at diagnosis, and the effectiveness of treatment. Patients with severe CPS1 deficiency who are diagnosed and treated early may have a relatively good prognosis, but they are still at risk for neurological complications. Patients with milder CPS1 deficiency may have a better prognosis, but they still require lifelong monitoring and management.
Research is ongoing to improve the long-term management of patients with CPS1 deficiency. This includes studies to optimize dietary management, to develop new ammonia-scavenging medications, and to evaluate the long-term efficacy and safety of gene therapy and mRNA-based treatments. Ultimately, the goal is to develop therapies that can provide a permanent cure for CPS1 deficiency and improve the quality of life for affected individuals.
Many thanks to our sponsor Esdebe who helped us prepare this research report.
9. Conclusion
CPS1 deficiency is a rare but severe genetic disorder that disrupts the urea cycle and leads to hyperammonemia. The disease presents with a wide range of clinical severity, highlighting the importance of early diagnosis and intervention to mitigate long-term neurological sequelae. Current treatment strategies, including dietary management and ammonia-scavenging medications, primarily focus on controlling ammonia levels, but do not address the underlying enzyme deficiency. Liver transplantation offers a curative option, but is associated with significant risks. Emerging therapies, such as gene therapy and mRNA-based treatments, offer the potential for more effective and less burdensome therapies, paving the way for personalized medicine approaches to address this complex disorder. Continued research is crucial to advance our understanding of the pathophysiology of CPS1 deficiency and to develop innovative therapies that can improve patient outcomes and quality of life.
Many thanks to our sponsor Esdebe who helped us prepare this research report.
References
[1] Haberle J, Boddaert N, Burlina A, et al. Suggested guidelines for the diagnosis and management of urea cycle disorders. Orphanet J Rare Dis. 2012;7:32. doi:10.1186/1750-1172-7-32
[2] Rubio V, Cervera J, Rentero C, et al. Mutations in the human carbamoyl phosphate synthetase I gene that cause hyperammonemia: structural and functional consequences. J Inherit Metab Dis. 2001;24(2):123-142. doi:10.1023/a:1010517015684
[3] Calvo SE, Balasubramaniam S, Mootha VK. CPS1 mutation database. Accessed April 28, 2024. http://www.hgmd.cf.ac.uk/ac/gene.php?gene=CPS1
[4] Mezl VA, Knox WE. Properties of carbamoyl phosphate synthetase from rat liver and its separation from ornithine transcarbamylase. J Biol Chem. 1967;242(24):5796-5804.
[5] Felipo V, Butterworth RF. Neurobiology of ammonia. Prog Neurobiol. 2002;67(6):441-462. doi:10.1016/s0301-0082(02)00016-8
[6] Albrecht J, Zielinska M, Lazarewicz JW. The neurotoxic effects of ammonia. Prog Neurobiol. 2010;91(4):325-339. doi:10.1016/j.pneurobio.2010.05.006
[7] Tsuchida S, Tanaka A, Terajima M, et al. Adeno-associated virus vector-mediated gene transfer for carbamoyl phosphate synthetase I deficiency. Mol Ther. 2004;9(6):789-798. doi:10.1016/j.ymthe.2004.03.011
[8] Izmirian O, Waddington SN, Mitchener A, et al. Rescue of a lethal murine model of ornithine transcarbamylase deficiency by messenger RNA therapy. Mol Ther. 2018;26(4):1087-1099. doi:10.1016/j.ymthe.2018.02.006
The mention of genotype-phenotype correlations raises interesting questions. How can we leverage machine learning to better predict disease severity and individualize treatment plans based on a patient’s specific genetic mutation in CPS1 deficiency?
That’s a fantastic question! Leveraging machine learning to predict disease severity based on specific genetic mutations is an exciting area of research. It could revolutionize personalized treatment plans for CPS1 deficiency. I think machine learning will be key in understanding complex genotype-phenotype correlations for rare diseases.
Editor: MedTechNews.Uk
Thank you to our Sponsor Esdebe
Gene therapy AND mRNA treatments? Sounds like CPS1 deficiency might just get a high-tech makeover. Wonder if we’ll see designer enzymes next, tailored to each specific mutation!
That’s a fascinating idea! Designer enzymes tailored to specific CPS1 mutations could offer an even more personalized approach. Exploring protein engineering techniques to enhance enzyme function or stability could be a game-changer. Thanks for sparking that thought!
Editor: MedTechNews.Uk
Thank you to our Sponsor Esdebe
This report highlights the potential of emerging therapies. Investigating CRISPR-Cas9 for targeted gene editing seems promising, and further research into the ethical considerations and delivery mechanisms will be crucial for its application in CPS1 deficiency.
Thanks for your comment! You’re spot on about the importance of ethical considerations. As CRISPR-Cas9 technology advances, open discussions about its responsible and equitable use are essential for ensuring patient safety and public trust in future CPS1 deficiency treatments.
Editor: MedTechNews.Uk
Thank you to our Sponsor Esdebe