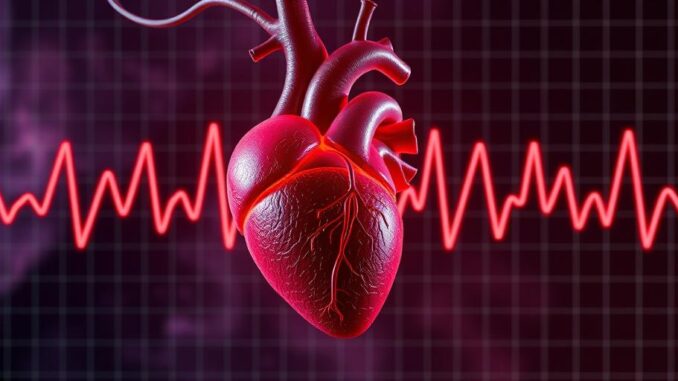
Abstract
Ventricular tachycardia (VT) represents a significant clinical challenge characterized by rapid, life-threatening heart rhythms originating in the ventricles. This condition can lead to hemodynamic instability, sudden cardiac death, and a diminished quality of life. This research report provides a comprehensive overview of VT, encompassing its underlying mechanisms, diagnostic modalities, and contemporary treatment strategies. We delve into the etiological diversity of VT, spanning from structural heart disease to inherited channelopathies, and explore the intricacies of electrophysiological mapping for arrhythmia localization. Current treatment options, including antiarrhythmic drugs and implantable cardioverter-defibrillators (ICDs), are critically evaluated, highlighting their limitations and associated risks. Furthermore, we examine the emerging role of pulsed field ablation (PFA) as a novel ablation modality, assessing its potential benefits and challenges in the context of VT management. The long-term prognosis of VT patients is discussed, considering factors such as recurrence rates, mortality risk, and the impact of comorbidities. Finally, we explore the economic burden associated with VT management and consider the potential cost-effectiveness of new therapies such as PFA, acknowledging the need for further research to define its place in the VT treatment algorithm. This report aims to provide a comprehensive resource for experts in the field, facilitating a deeper understanding of VT and informing future research and clinical practice.
Many thanks to our sponsor Esdebe who helped us prepare this research report.
1. Introduction
Ventricular tachycardia (VT) is a cardiac arrhythmia characterized by a rapid, organized series of ventricular depolarizations at a rate typically exceeding 100 beats per minute. This rapid ventricular activation can compromise cardiac output, leading to symptoms ranging from palpitations and dizziness to syncope and sudden cardiac death (SCD). The clinical presentation and underlying mechanisms of VT are highly variable, reflecting the diverse etiologies and electrophysiological substrates involved. Understanding the complex interplay between these factors is crucial for effective diagnosis, risk stratification, and management.
VT can be broadly classified as monomorphic or polymorphic, based on the QRS morphology on the electrocardiogram (ECG). Monomorphic VT exhibits a uniform QRS morphology, indicating a single site of origin within the ventricles. Polymorphic VT, on the other hand, displays a variable QRS morphology, suggesting multiple foci of ventricular activation or a shifting re-entrant circuit. Torsades de pointes, a specific type of polymorphic VT associated with QT prolongation, is particularly dangerous and often degenerates into ventricular fibrillation (VF). The distinction between these VT subtypes is clinically relevant, as it can guide diagnostic evaluation and treatment strategies.
The incidence of VT varies depending on the population studied and the presence of underlying heart disease. In patients with structural heart disease, such as ischemic cardiomyopathy, dilated cardiomyopathy, or hypertrophic cardiomyopathy, VT is a common occurrence and a significant contributor to SCD. In individuals without apparent structural heart disease, VT may be associated with inherited channelopathies, such as long QT syndrome, Brugada syndrome, or catecholaminergic polymorphic ventricular tachycardia (CPVT). Idiopathic VT, which occurs in the absence of identifiable structural or electrical abnormalities, accounts for a smaller proportion of cases.
This report aims to provide a comprehensive overview of VT, covering its underlying mechanisms, diagnostic approaches, current treatment options, and emerging therapies. We will critically evaluate the limitations of existing treatments and explore the potential role of pulsed field ablation (PFA) as a novel ablation modality. Furthermore, we will discuss the long-term prognosis of VT patients and the economic burden associated with VT management.
Many thanks to our sponsor Esdebe who helped us prepare this research report.
2. Etiology and Mechanisms of Ventricular Tachycardia
The pathogenesis of VT is multifactorial, involving complex interactions between electrical and structural factors. The underlying mechanisms can be broadly categorized into re-entry, abnormal automaticity, and triggered activity.
2.1 Re-entry
Re-entry is the most common mechanism underlying VT, particularly in the context of structural heart disease. It involves the circulation of an electrical impulse around an anatomical or functional obstacle, creating a self-sustaining circuit that perpetuates the arrhythmia. For re-entry to occur, several conditions must be met: (1) a region of unidirectional block, preventing the impulse from propagating in one direction; (2) a circuit with a pathway for slow conduction, allowing the impulse to traverse the blocked region and complete the circuit; and (3) a recovery time of the tissue within the circuit that is shorter than the cycle length of the circulating impulse.
In patients with ischemic cardiomyopathy, scar tissue resulting from myocardial infarction can create anatomical obstacles that facilitate re-entry. The border zone surrounding the scar tissue often exhibits slow conduction and prolonged refractoriness, providing a suitable substrate for re-entrant VT. Similarly, in patients with dilated cardiomyopathy, fibrosis and structural remodeling can disrupt normal electrical propagation and promote re-entry. In these cases, VT is often characterized by monomorphic QRS complexes, reflecting a relatively stable re-entrant circuit within the scar tissue.
2.2 Abnormal Automaticity
Abnormal automaticity refers to the spontaneous generation of action potentials by ventricular myocytes. This can occur in the setting of ischemia, electrolyte imbalances, or exposure to certain medications. Abnormal automaticity can initiate VT by triggering a rapid sequence of ventricular depolarizations. Unlike re-entry, abnormal automaticity does not require a pre-existing circuit and can occur in the absence of structural heart disease. VT due to abnormal automaticity often exhibits a gradual onset and termination, and the QRS morphology may be variable.
2.3 Triggered Activity
Triggered activity arises from abnormal depolarizations that occur during or after the repolarization phase of the action potential. These abnormal depolarizations, known as early afterdepolarizations (EADs) and delayed afterdepolarizations (DADs), can trigger action potentials if they reach a threshold level. EADs are associated with prolonged action potential duration and are commonly observed in patients with long QT syndrome. DADs, on the other hand, are associated with intracellular calcium overload and are often seen in patients with digitalis toxicity or catecholamine excess. Triggered activity can initiate VT by inducing premature ventricular complexes (PVCs) that lead to a rapid sequence of ventricular depolarizations. The QRS morphology of VT due to triggered activity can be variable, depending on the location of the triggering focus.
2.4 Genetic and Molecular Mechanisms
Inherited channelopathies, such as long QT syndrome, Brugada syndrome, and CPVT, are caused by mutations in genes encoding ion channels or associated proteins. These mutations can disrupt normal cardiac electrophysiology and increase the risk of VT and SCD. For example, mutations in the KCNQ1 or KCNH2 genes, which encode potassium channels, can prolong the QT interval and predispose individuals to torsades de pointes. Mutations in the SCN5A gene, which encodes the sodium channel, can cause Brugada syndrome, characterized by ST-segment elevation in the right precordial leads and an increased risk of VT and SCD. CPVT is caused by mutations in genes encoding the ryanodine receptor (RyR2) or calsequestrin 2 (CASQ2), which regulate intracellular calcium handling. These mutations can lead to DADs and triggered activity, particularly during exercise or emotional stress.
The genetic and molecular mechanisms underlying VT are complex and involve a variety of ion channels, signaling pathways, and structural proteins. Further research is needed to fully elucidate these mechanisms and develop targeted therapies for inherited VT syndromes.
Many thanks to our sponsor Esdebe who helped us prepare this research report.
3. Diagnostic Methods
The diagnosis of VT relies on a combination of clinical history, physical examination, and electrocardiographic findings. A comprehensive diagnostic evaluation is essential to identify the underlying cause of VT, assess the risk of SCD, and guide treatment decisions.
3.1 Electrocardiography (ECG)
The ECG is the cornerstone of VT diagnosis. During VT, the ECG typically shows a wide QRS complex tachycardia, with a rate exceeding 100 beats per minute. The QRS morphology can be monomorphic or polymorphic, depending on the underlying mechanism and the presence of structural heart disease. Certain ECG features, such as the presence of atrioventricular dissociation, fusion beats, or capture beats, can help differentiate VT from supraventricular tachycardia (SVT) with aberrant conduction. However, these features are not always present, and the diagnosis of VT may require further evaluation.
The 12-lead ECG is also essential for determining the QRS axis and morphology, which can provide clues about the origin of the VT. For example, a left bundle branch block morphology with a superior axis is often indicative of VT originating from the right ventricle, while a right bundle branch block morphology with an inferior axis may suggest VT originating from the left ventricle.
3.2 Holter Monitoring and Event Recorders
Holter monitoring involves continuous ECG recording for 24-48 hours, allowing for the detection of infrequent or paroxysmal VT episodes. Event recorders, which are patient-activated devices, can be used to record ECGs during symptomatic episodes. These devices are particularly useful for capturing infrequent VT episodes that may not be detected during Holter monitoring.
3.3 Exercise Stress Testing
Exercise stress testing can be used to evaluate the relationship between exercise and VT. In some patients, VT may be induced or exacerbated by exercise, while in others, it may be suppressed. Exercise stress testing can also help identify patients with CPVT, in whom VT is typically triggered by exercise or emotional stress.
3.4 Signal-Averaged ECG (SAECG)
SAECG is a non-invasive technique used to detect late potentials, which are low-amplitude signals that occur at the end of the QRS complex. Late potentials are thought to reflect slow conduction in areas of scar tissue and are associated with an increased risk of VT in patients with structural heart disease. However, the sensitivity and specificity of SAECG for predicting VT are limited.
3.5 Echocardiography
Echocardiography is used to assess cardiac structure and function, including left ventricular ejection fraction (LVEF), wall motion abnormalities, and valvular disease. Echocardiography can help identify patients with structural heart disease who are at increased risk of VT. In addition, echocardiography can be used to assess the severity of heart failure, which is a common comorbidity in patients with VT.
3.6 Cardiac Magnetic Resonance Imaging (MRI)
Cardiac MRI provides detailed anatomical and functional information about the heart, including scar tissue, fibrosis, and edema. Late gadolinium enhancement (LGE) imaging, a specific type of cardiac MRI, can be used to identify scar tissue in patients with ischemic cardiomyopathy or dilated cardiomyopathy. The presence and extent of scar tissue are strongly associated with an increased risk of VT. Cardiac MRI can also be used to assess the presence of arrhythmogenic right ventricular cardiomyopathy (ARVC), a genetic disorder characterized by fibrofatty replacement of the right ventricle.
3.7 Electrophysiological Study (EPS)
EPS is an invasive procedure that involves inserting catheters into the heart to record electrical activity and induce arrhythmias. EPS is used to identify the mechanism of VT, localize the site of origin, and assess the efficacy of antiarrhythmic drugs or ablation. During EPS, programmed electrical stimulation is used to induce VT. The characteristics of the induced VT, such as the cycle length, QRS morphology, and response to pacing maneuvers, can help determine the mechanism and location of the arrhythmia. EPS is particularly useful for patients with recurrent VT or those at high risk of SCD.
Many thanks to our sponsor Esdebe who helped us prepare this research report.
4. Current Treatment Options
The management of VT aims to prevent SCD, reduce symptom burden, and improve quality of life. Treatment options include antiarrhythmic drugs, implantable cardioverter-defibrillators (ICDs), and catheter ablation.
4.1 Antiarrhythmic Drugs
Antiarrhythmic drugs are used to suppress VT by altering cardiac electrophysiology. These drugs are classified according to the Vaughan Williams classification system, which categorizes them based on their primary mechanism of action. Class I drugs block sodium channels, Class II drugs block beta-adrenergic receptors, Class III drugs prolong repolarization, and Class IV drugs block calcium channels. However, this classification system is imperfect, as many antiarrhythmic drugs have multiple mechanisms of action.
Commonly used antiarrhythmic drugs for VT include amiodarone, sotalol, and lidocaine. Amiodarone is a Class III drug that prolongs repolarization and also has sodium channel-blocking and beta-adrenergic-blocking effects. Amiodarone is highly effective in suppressing VT, but it has a high incidence of side effects, including thyroid dysfunction, pulmonary toxicity, and liver toxicity. Sotalol is a Class III drug that also has beta-adrenergic-blocking effects. Sotalol is less effective than amiodarone in suppressing VT, but it has fewer side effects. Lidocaine is a Class Ib drug that blocks sodium channels. Lidocaine is primarily used for the acute termination of VT, but it is not effective for long-term prevention.
The use of antiarrhythmic drugs for VT is limited by their potential side effects and proarrhythmic effects. Antiarrhythmic drugs can prolong the QT interval and increase the risk of torsades de pointes. In addition, some antiarrhythmic drugs can depress myocardial contractility and worsen heart failure. The decision to use antiarrhythmic drugs for VT should be made carefully, considering the potential benefits and risks.
4.2 Implantable Cardioverter-Defibrillators (ICDs)
ICDs are electronic devices that are implanted in the chest to monitor heart rhythm and deliver electrical shocks or pacing to terminate VT or VF. ICDs are highly effective in preventing SCD in patients at high risk of VT or VF. ICDs are typically implanted in patients with a history of sustained VT or VF, or those with structural heart disease and a high risk of SCD. The primary indication for ICD implantation is secondary prevention of SCD in patients who have already experienced a life-threatening arrhythmia. ICDs are also indicated for primary prevention of SCD in patients with structural heart disease and a high risk of SCD, such as those with LVEF ≤35% after myocardial infarction.
While ICDs are highly effective in preventing SCD, they do not prevent VT or VF from occurring. ICD shocks can be painful and can lead to anxiety and psychological distress. In addition, ICDs can malfunction or require replacement. Strategies to reduce the frequency of ICD shocks include optimizing medical therapy, catheter ablation, and anti-tachycardia pacing (ATP), which involves delivering rapid pacing to terminate VT without delivering a shock.
4.3 Catheter Ablation
Catheter ablation is a minimally invasive procedure that involves using radiofrequency energy or cryoenergy to create lesions in the heart tissue that cause VT. Catheter ablation is increasingly being used to treat VT, particularly in patients with recurrent VT or those who are intolerant of antiarrhythmic drugs. Catheter ablation can be performed using a variety of techniques, including mapping-guided ablation, substrate-based ablation, and epicardial ablation. Mapping-guided ablation involves using electrophysiological mapping to identify the critical sites within the re-entrant circuit that are necessary for VT to occur. Substrate-based ablation involves targeting the underlying structural abnormalities that promote VT, such as scar tissue or fibrosis. Epicardial ablation involves accessing the epicardial surface of the heart to ablate VT originating from the outer layer of the ventricle. This is often necessary for VT associated with non-ischemic cardiomyopathies.
Catheter ablation can be effective in reducing the frequency of VT episodes and improving quality of life. However, catheter ablation is not always successful, and VT can recur in some patients. The success rate of catheter ablation depends on the underlying mechanism of VT, the extent of scar tissue, and the ablation technique used. Catheter ablation is associated with a small risk of complications, including bleeding, infection, cardiac perforation, and stroke.
Many thanks to our sponsor Esdebe who helped us prepare this research report.
5. Pulsed Field Ablation (PFA): A Novel Approach
Pulsed field ablation (PFA) is an emerging ablation modality that utilizes short, high-voltage electrical pulses to create lesions in the heart tissue. Unlike radiofrequency ablation (RFA) and cryoablation, which rely on thermal energy to induce cell death, PFA selectively targets cardiomyocytes while sparing surrounding structures such as the esophagus and phrenic nerve. This tissue-selective mechanism of action is thought to reduce the risk of complications associated with thermal ablation, such as esophageal injury and phrenic nerve palsy.
5.1 Mechanism of Action
PFA works by creating nanopores in the cell membranes of cardiomyocytes, leading to irreversible electroporation and cell death. The electrical pulses are delivered in a rapid sequence, creating a strong electric field that disrupts the cell membrane. The selectivity of PFA for cardiomyocytes is thought to be due to the larger size and lower impedance of cardiomyocytes compared to other tissue types. This allows PFA to target cardiomyocytes while sparing surrounding structures such as blood vessels and nerves.
5.2 Potential Benefits of PFA in VT Management
PFA offers several potential advantages over traditional ablation modalities in the management of VT:
- Tissue Selectivity: PFA’s tissue-selective mechanism of action may reduce the risk of complications such as esophageal injury and phrenic nerve palsy. This is particularly important for VT ablation, where the ablation targets are often located near critical structures.
- Faster Ablation Times: PFA can create lesions more rapidly than RFA or cryoablation, potentially reducing procedure times and improving patient comfort.
- Reduced Pain: PFA is thought to be less painful than RFA or cryoablation, potentially reducing the need for anesthesia or sedation.
- Improved Lesion Durability: Early data suggests that PFA lesions may be more durable than RFA or cryoablation lesions, potentially reducing the risk of VT recurrence.
5.3 Current Evidence and Clinical Trials
The clinical experience with PFA for VT is still limited, but early studies have shown promising results. Several ongoing clinical trials are evaluating the safety and efficacy of PFA for VT ablation. These trials are comparing PFA to RFA or cryoablation in patients with various types of VT. The results of these trials will provide valuable information about the role of PFA in VT management.
5.4 Challenges and Limitations
Despite its potential advantages, PFA also faces several challenges and limitations in the context of VT management:
- Energy Delivery: Ensuring consistent and effective energy delivery with PFA can be challenging, particularly in areas with complex anatomy or scar tissue. Optimization of PFA catheters and ablation protocols is ongoing.
- Long-Term Outcomes: Long-term data on the efficacy and safety of PFA for VT are still lacking. Further research is needed to determine the durability of PFA lesions and the long-term risk of VT recurrence.
- Cost-Effectiveness: The cost of PFA systems and catheters may be higher than traditional ablation modalities. Cost-effectiveness analyses are needed to determine the value of PFA in VT management.
Many thanks to our sponsor Esdebe who helped us prepare this research report.
6. Long-Term Prognosis and Management Strategies
The long-term prognosis of VT patients varies depending on the underlying cause, the severity of heart disease, and the effectiveness of treatment. Patients with structural heart disease and recurrent VT have a higher risk of SCD and heart failure progression. Effective management strategies are essential to improve the long-term outcomes of VT patients.
6.1 Risk Stratification
Risk stratification is a crucial step in the management of VT patients. Risk stratification involves assessing the risk of SCD and heart failure progression based on clinical, electrocardiographic, and imaging findings. Factors that are associated with an increased risk of SCD include a history of sustained VT or VF, LVEF ≤35%, the presence of scar tissue on cardiac MRI, and the presence of inducible VT during EPS. Patients at high risk of SCD should be considered for ICD implantation.
6.2 Medical Management
Medical management of VT patients includes optimizing medical therapy for underlying heart disease, such as heart failure, coronary artery disease, and hypertension. Antiarrhythmic drugs may be used to suppress VT and reduce the frequency of ICD shocks. However, the use of antiarrhythmic drugs is limited by their potential side effects and proarrhythmic effects. In some cases, medical management may involve addressing underlying electrolyte imbalances or medication side effects that can contribute to VT.
6.3 Device Therapy
Device therapy for VT patients includes ICDs and cardiac resynchronization therapy (CRT). ICDs are used to prevent SCD in patients at high risk of VT or VF. CRT is used to improve cardiac function in patients with heart failure and ventricular dyssynchrony. CRT can also reduce the frequency of VT episodes by improving ventricular hemodynamics and reducing myocardial stress. Some ICDs also offer anti-tachycardia pacing (ATP) which can terminate VT without delivering a painful shock.
6.4 Lifestyle Modifications
Lifestyle modifications are an important part of the management of VT patients. These modifications include avoiding triggers that can provoke VT, such as excessive alcohol consumption, caffeine intake, and strenuous exercise. Patients should also be educated about the signs and symptoms of VT and how to respond to VT episodes. Regular follow-up with a cardiologist is essential to monitor heart rhythm and adjust treatment as needed.
Many thanks to our sponsor Esdebe who helped us prepare this research report.
7. Economic Burden and Cost-Effectiveness of New Treatments
Ventricular tachycardia imposes a significant economic burden on healthcare systems due to the costs associated with diagnosis, treatment, and long-term management. These costs include hospitalizations, procedures (such as EPS and ablation), device implantation and maintenance, medications, and outpatient follow-up. The economic burden is particularly high for patients with recurrent VT or those requiring multiple interventions.
Newer treatments for VT, such as PFA, have the potential to improve outcomes and reduce the economic burden associated with VT management. However, the cost-effectiveness of these treatments needs to be carefully evaluated. Cost-effectiveness analyses compare the costs of a new treatment to its benefits, such as improved survival, reduced hospitalization rates, and improved quality of life. These analyses can help healthcare providers and policymakers make informed decisions about the adoption of new treatments.
The cost-effectiveness of PFA for VT will depend on several factors, including the cost of the PFA system and catheters, the success rate of PFA ablation, the long-term durability of PFA lesions, and the reduction in complications compared to traditional ablation modalities. If PFA can effectively reduce VT recurrence rates and complications, it may be cost-effective compared to traditional ablation modalities, even if the initial cost is higher.
Further research is needed to evaluate the cost-effectiveness of new treatments for VT, such as PFA. These studies should consider the long-term costs and benefits of these treatments and should be conducted in a variety of healthcare settings. The results of these studies will help guide the adoption of new treatments and improve the overall economic value of VT management.
Many thanks to our sponsor Esdebe who helped us prepare this research report.
8. Future Directions and Research Opportunities
The field of VT management is rapidly evolving, with ongoing research focused on improving diagnostic methods, developing new treatment strategies, and enhancing our understanding of the underlying mechanisms of VT. Several promising areas of research hold the potential to significantly impact the future of VT management.
8.1 Advanced Imaging Techniques
Advanced imaging techniques, such as cardiac MRI with late gadolinium enhancement (LGE) and cardiac computed tomography (CT), are increasingly being used to identify scar tissue and other structural abnormalities that contribute to VT. These techniques can help guide ablation procedures by providing detailed anatomical information about the ablation targets. Future research should focus on developing new imaging techniques that can provide even more detailed information about the electrophysiological substrate of VT. This could involve developing techniques that can directly visualize areas of slow conduction or identify the specific ion channel abnormalities that contribute to VT.
8.2 Personalized Medicine Approaches
Personalized medicine approaches, which tailor treatment to the individual patient based on their genetic and clinical characteristics, hold great promise for improving VT management. Genetic testing can be used to identify patients with inherited channelopathies that predispose them to VT. This information can be used to guide treatment decisions and select the most appropriate antiarrhythmic drug or ablation strategy. Future research should focus on developing new biomarkers that can predict the response to treatment and identify patients who are at high risk of VT recurrence.
8.3 Novel Ablation Technologies
Novel ablation technologies, such as PFA and high-intensity focused ultrasound (HIFU), offer the potential to improve the safety and efficacy of VT ablation. These technologies can create lesions more rapidly and with greater precision than traditional ablation modalities. Future research should focus on optimizing these technologies and evaluating their long-term outcomes. In addition, research should focus on developing new ablation techniques that can target the epicardial surface of the heart, which is often the site of origin of VT in patients with non-ischemic cardiomyopathies.
8.4 Computational Modeling and Simulation
Computational modeling and simulation are increasingly being used to study the electrophysiological mechanisms of VT and to develop new treatment strategies. These models can simulate the propagation of electrical impulses in the heart and can be used to predict the effects of antiarrhythmic drugs or ablation on VT. Future research should focus on developing more sophisticated models that can incorporate patient-specific anatomical and electrophysiological data. These models could be used to personalize treatment decisions and optimize ablation strategies.
Many thanks to our sponsor Esdebe who helped us prepare this research report.
9. Conclusion
Ventricular tachycardia remains a significant clinical challenge, requiring a comprehensive and multidisciplinary approach to diagnosis, risk stratification, and management. While current treatment options, including antiarrhythmic drugs and ICDs, have proven effective in reducing mortality and improving quality of life, they are not without limitations. The emergence of novel therapies such as PFA holds promise for improving the safety and efficacy of VT ablation. However, further research is needed to fully understand the potential benefits and risks of PFA and to define its place in the VT treatment algorithm.
The future of VT management lies in the development of personalized approaches that tailor treatment to the individual patient based on their genetic and clinical characteristics. Advanced imaging techniques, genetic testing, and computational modeling will play an increasingly important role in guiding treatment decisions and optimizing outcomes. By continuing to invest in research and innovation, we can improve the lives of patients with VT and reduce the burden of this life-threatening arrhythmia.
Many thanks to our sponsor Esdebe who helped us prepare this research report.
References
- Al-Khatib SM, Stevenson WG, Ackerman MJ, et al. 2017 AHA/ACC/HRS guideline for management of patients with ventricular arrhythmias and the prevention of sudden cardiac death: Executive summary: A report of the American College of Cardiology/American Heart Association Task Force on Clinical Practice Guidelines and the Heart Rhythm Society. Circulation. 2018;138(16):e210-e271.
- Cronin EM, Bogun F, Maury P, et al. 2019 HRS/EHRA expert consensus statement on catheter ablation of ventricular arrhythmias. Heart Rhythm. 2019;16(1):e1-e148.
- Callans DJ. Catheter ablation of ventricular tachycardia. Circulation. 2011;124(20):2234-2242.
- Reddy VY, Dukkipati SR, Neuzil P, et al. Pulsed Field Ablation for Atrial Fibrillation. J Am Coll Cardiol. 2018;72(22):2750-2759.
- Koruth APP, Reddy VY, Natale A. Pulsed Field Ablation in Atrial Fibrillation: Clinical Applications, Limitations, and Future Directions. Arrhythmia Electrophysiol Rev. 2021;10(1):17-24.
- Soejima K, Stevenson WG, Sapp JL, et al. Association of ischemic scar with localized reentry circuit as a mechanism of ventricular tachycardia. J Am Coll Cardiol. 2001;37(2):448-453.
- Priori SG, Blomström-Lundqvist C, Mazzanti A, et al. 2015 ESC Guidelines for management of patients with ventricular arrhythmias and the prevention of sudden cardiac death: The Task Force for the Management of Patients with Ventricular Arrhythmias and the Prevention of Sudden Cardiac Death of the European Society of Cardiology (ESC). Eur Heart J. 2015;36(41):2793-2867.
- Zeppenfeld K, Tfelt-Hansen J, de Riva M, et al. 2022 ESC Guidelines for the management of patients with ventricular arrhythmias and the prevention of sudden cardiac death. Eur Heart J. 2022;43(39):3997-4126.
- Arenal A, Jauregui B, Arbelo E, et al. Use of pulsed field ablation in ventricular tachycardia: A systematic review and meta-analysis. J Interv Card Electrophysiol. 2023;67(1):155-164.
- Verma S, Haïssaguerre M, Sanders P. Pulsed field ablation for ventricular tachycardia: Promise and future directions. Heart Rhythm. 2023;20(4):646-647.
So, PFA selectively zaps cardiomyocytes, huh? Does this mean we can finally ditch the whole “shock and awe” approach and be more like a cardiac ninja? Asking for a friend whose ICD is a little *too* enthusiastic.
That’s a great analogy! The potential for more targeted therapies like PFA is definitely exciting, especially for those whose ICDs are a little *too* eager. Hopefully, we’ll see refinements that make VT treatment more precise and less reliant on shocks in the future. Perhaps less of a shock and awe and more of a calm and collected approach! Thanks for the interesting question.
Editor: MedTechNews.Uk
Thank you to our Sponsor Esdebe
The discussion of genetic and molecular mechanisms opens up interesting avenues. Could inherited channelopathies be better understood through advanced computational modelling, potentially leading to more targeted drug therapies or even gene editing approaches to correct the underlying defects?
That’s a fantastic point! Computational modelling could really accelerate our understanding of channelopathies. By simulating the effects of genetic mutations on cardiac electrophysiology, we might pinpoint specific targets for drug development or even explore the feasibility of gene editing to correct these defects. It’s an exciting prospect!
Editor: MedTechNews.Uk
Thank you to our Sponsor Esdebe
The discussion of personalized medicine approaches, incorporating genetic and clinical data, highlights the potential for more effective VT management. Further research into identifying predictive biomarkers could significantly improve treatment selection and outcomes.
Thanks for highlighting the importance of personalized medicine! Identifying predictive biomarkers will definitely refine our treatment strategies. Imagine tailoring VT therapy based on individual genetic profiles – it’s a future worth striving for! This could vastly improve outcomes and reduce unnecessary interventions. What are your thoughts on the timeline for this progress?
Editor: MedTechNews.Uk
Thank you to our Sponsor Esdebe