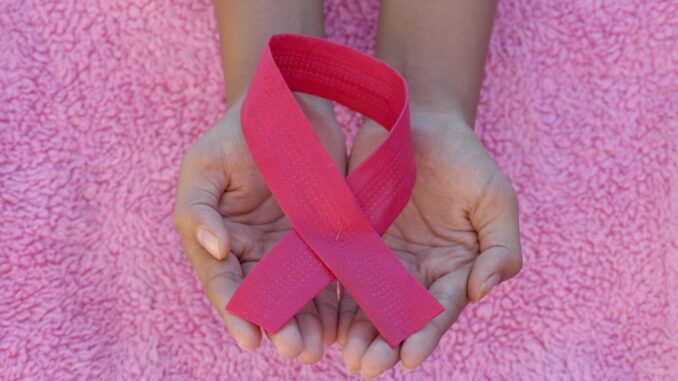
Abstract
Tumor biology is a complex and rapidly evolving field. Despite significant advancements in understanding cancer development and progression, it remains a leading cause of mortality worldwide. This research report provides an in-depth overview of recent advances in tumor biology, focusing on key aspects such as tumor microenvironment (TME) interactions, cancer metabolism, immune evasion mechanisms, and metastasis. Furthermore, it explores novel therapeutic strategies, including targeted therapies, immunotherapies, oncolytic viruses, and emerging approaches like CRISPR-based gene editing and nanotechnology-based drug delivery. The report critically evaluates the current limitations in cancer treatment and highlights promising future directions aimed at improving patient outcomes and achieving long-term remission or cure.
Many thanks to our sponsor Esdebe who helped us prepare this research report.
1. Introduction
Cancer, characterized by uncontrolled cell growth and the potential to invade other parts of the body, poses a significant global health challenge. Our understanding of the fundamental principles driving tumorigenesis has expanded dramatically over the past few decades. The classical view of cancer as a disease solely driven by genetic mutations within tumor cells has evolved to encompass the crucial role of the tumor microenvironment (TME), metabolic reprogramming, and immune evasion. Furthermore, the heterogeneity of tumors, both between patients and within individual tumors, presents a significant obstacle to developing universally effective therapies.
This report aims to provide a comprehensive overview of recent advancements in tumor biology, critically examining key mechanisms that govern cancer development and progression. It will delve into the intricate interplay between tumor cells and the TME, explore the complexities of cancer metabolism and its role in tumor survival, analyze the mechanisms by which tumors evade the immune system, and discuss the process of metastasis. Furthermore, the report will evaluate the current landscape of therapeutic strategies, encompassing both established and emerging approaches, and will highlight promising future directions in cancer research and treatment.
Many thanks to our sponsor Esdebe who helped us prepare this research report.
2. The Tumor Microenvironment: A Critical Determinant of Cancer Progression
The tumor microenvironment (TME) is a complex ecosystem surrounding tumor cells, composed of various cellular and non-cellular components that significantly influence tumor development, growth, and metastasis. This intricate network includes fibroblasts, immune cells, endothelial cells, extracellular matrix (ECM), signaling molecules, and the vasculature. A better understanding of the TME is proving to be vital for the development of effective cancer therapies.
2.1 Cellular Components of the TME
- Cancer-Associated Fibroblasts (CAFs): CAFs are one of the most abundant cell types in the TME. They are activated fibroblasts that promote tumor growth, angiogenesis, and ECM remodeling. CAFs secrete growth factors, cytokines, and ECM components that support tumor cell survival and proliferation. Recent research suggests that CAFs can also contribute to drug resistance by creating a physical barrier that limits drug penetration or by secreting factors that protect tumor cells from chemotherapeutic agents [1].
- Immune Cells: The TME contains a variety of immune cells, including T cells, B cells, natural killer (NK) cells, macrophages, and dendritic cells (DCs). While some immune cells, such as cytotoxic T lymphocytes (CTLs) and NK cells, can recognize and kill tumor cells, others, such as tumor-associated macrophages (TAMs) and myeloid-derived suppressor cells (MDSCs), can promote tumor growth and suppress anti-tumor immunity. The balance between these pro- and anti-tumor immune responses within the TME is a critical determinant of cancer progression [2].
- Endothelial Cells: Endothelial cells line the blood vessels that supply tumors with nutrients and oxygen. Tumor angiogenesis, the formation of new blood vessels, is essential for tumor growth and metastasis. Endothelial cells in the TME are often abnormal, exhibiting increased permeability and structural irregularities. Anti-angiogenic therapies, which target endothelial cells and disrupt tumor blood supply, have shown some success in treating certain cancers, but resistance often develops [3].
2.2 Non-Cellular Components of the TME
- Extracellular Matrix (ECM): The ECM is a complex network of proteins and polysaccharides that provides structural support to tissues and regulates cell adhesion, migration, and differentiation. In the TME, the ECM is often remodeled and altered, becoming more rigid and cross-linked. This altered ECM can promote tumor cell invasion and metastasis by providing a scaffold for tumor cells to migrate along and by activating signaling pathways that promote cell motility [4].
- Signaling Molecules: The TME is rich in signaling molecules, including growth factors, cytokines, chemokines, and metabolites. These molecules mediate communication between tumor cells and other cells in the TME, influencing tumor growth, angiogenesis, immune responses, and metastasis. Targeting these signaling pathways has become a major focus of cancer therapy [5].
2.3 Targeting the TME for Cancer Therapy
Given the crucial role of the TME in cancer progression, targeting the TME has emerged as a promising therapeutic strategy. Several approaches are being investigated, including:
- CAF depletion or inactivation: Strategies to deplete or inactivate CAFs are being developed to disrupt the supportive role of these cells in tumor growth and metastasis.
- Immunomodulation of the TME: Efforts are underway to reprogram the immune response within the TME to favor anti-tumor immunity. This includes strategies to activate CTLs and NK cells, inhibit TAMs and MDSCs, and enhance the presentation of tumor antigens to immune cells.
- Normalization of tumor vasculature: Approaches to normalize the abnormal tumor vasculature are being explored to improve drug delivery and reduce hypoxia, which can promote tumor resistance to therapy.
- ECM modulation: Strategies to remodel the ECM and disrupt its supportive role in tumor invasion and metastasis are being investigated.
Many thanks to our sponsor Esdebe who helped us prepare this research report.
3. Cancer Metabolism: Fueling Tumor Growth and Survival
Cancer cells exhibit distinct metabolic profiles compared to normal cells. This metabolic reprogramming allows them to efficiently acquire nutrients and energy to support their rapid proliferation and survival in the often nutrient-deprived TME. Understanding these metabolic alterations provides opportunities for developing novel therapeutic strategies targeting cancer-specific metabolic pathways.
3.1 The Warburg Effect
One of the most well-known metabolic features of cancer cells is the Warburg effect, which refers to the preference for glycolysis over oxidative phosphorylation (OXPHOS) for energy production, even in the presence of oxygen. While glycolysis is less efficient at producing ATP than OXPHOS, it allows cancer cells to rapidly generate ATP and produce biosynthetic precursors needed for cell growth and division. This metabolic shift also generates a more acidic microenvironment, which can promote tumor invasion and metastasis [6].
3.2 Glutamine Metabolism
In addition to glucose, cancer cells often rely heavily on glutamine as a source of carbon and nitrogen. Glutamine is converted to glutamate, which can then be used to synthesize other amino acids, nucleotides, and lipids. Glutamine metabolism also contributes to the maintenance of redox balance and the production of ATP [7].
3.3 Lipid Metabolism
Cancer cells often exhibit increased lipid synthesis and uptake. Lipids are essential for building cell membranes, storing energy, and producing signaling molecules. Aberrant lipid metabolism can contribute to tumor growth, survival, and metastasis [8].
3.4 Targeting Cancer Metabolism for Therapy
The unique metabolic features of cancer cells provide opportunities for developing targeted therapies. Several approaches are being investigated, including:
- Inhibiting glycolysis: Targeting enzymes involved in glycolysis, such as hexokinase and pyruvate kinase, can inhibit ATP production and suppress tumor growth.
- Disrupting glutamine metabolism: Inhibiting glutaminase, the enzyme that converts glutamine to glutamate, can deprive cancer cells of essential nutrients and energy.
- Targeting lipid metabolism: Inhibiting enzymes involved in lipid synthesis or uptake can disrupt cell membrane formation and energy storage.
- Metformin: Metformin, a commonly used drug for treating type 2 diabetes, has been shown to inhibit tumor growth in several types of cancer. Metformin’s anti-cancer effects are thought to be mediated by its ability to activate AMPK, a key regulator of energy metabolism [9].
Many thanks to our sponsor Esdebe who helped us prepare this research report.
4. Immune Evasion: How Tumors Escape Immune Surveillance
The immune system plays a critical role in recognizing and eliminating cancer cells. However, tumors have evolved various mechanisms to evade immune surveillance and destruction. Understanding these immune evasion mechanisms is crucial for developing effective immunotherapies that can unleash the power of the immune system to fight cancer.
4.1 Downregulation of MHC Class I Expression
Tumor cells can evade immune recognition by downregulating the expression of major histocompatibility complex (MHC) class I molecules, which are required for presenting tumor-associated antigens to cytotoxic T lymphocytes (CTLs). This downregulation prevents CTLs from recognizing and killing tumor cells [10].
4.2 Expression of Immune Checkpoint Molecules
Tumor cells can express immune checkpoint molecules, such as PD-L1 and CTLA-4, which bind to their respective receptors on T cells and inhibit T cell activation. By expressing these checkpoint molecules, tumor cells can effectively suppress anti-tumor immune responses [11].
4.3 Secretion of Immunosuppressive Factors
Tumor cells can secrete immunosuppressive factors, such as TGF-β and IL-10, which inhibit the activity of immune cells and promote the recruitment of immunosuppressive cells, such as regulatory T cells (Tregs) and myeloid-derived suppressor cells (MDSCs). These factors create an immunosuppressive microenvironment that protects tumor cells from immune attack [12].
4.4 Recruitment of Immunosuppressive Cells
Tumors can secrete chemokines that recruit immunosuppressive cells, such as Tregs and MDSCs, to the TME. These cells suppress anti-tumor immune responses and promote tumor growth and metastasis [13].
4.5 Antigen Masking
Tumor cells can shed antigens or express molecules that mask tumor-associated antigens, preventing immune cells from recognizing and targeting them [14].
4.6 Targeting Immune Evasion for Immunotherapy
Overcoming immune evasion is a major goal of cancer immunotherapy. Several approaches are being investigated, including:
- Immune checkpoint inhibitors: These drugs block the interaction between immune checkpoint molecules and their receptors, allowing T cells to become activated and kill tumor cells. Examples include anti-PD-1, anti-PD-L1, and anti-CTLA-4 antibodies.
- Adoptive cell therapy: This involves collecting immune cells from a patient, modifying them in the laboratory to enhance their anti-tumor activity, and then infusing them back into the patient. Examples include CAR-T cell therapy and TIL therapy.
- Cancer vaccines: These vaccines are designed to stimulate the immune system to recognize and attack tumor cells. They can be made from tumor-associated antigens, whole tumor cells, or dendritic cells pulsed with tumor antigens.
- Oncolytic viruses: These viruses selectively infect and kill cancer cells while also stimulating an anti-tumor immune response [15].
Many thanks to our sponsor Esdebe who helped us prepare this research report.
5. Metastasis: The Spread of Cancer to Distant Sites
Metastasis, the spread of cancer cells from the primary tumor to distant sites, is the leading cause of cancer-related deaths. The metastatic process is complex and involves a series of steps, including detachment of tumor cells from the primary tumor, invasion of the surrounding tissue, intravasation into blood vessels, survival in the circulation, extravasation from blood vessels at a distant site, and colonization of the distant site [16].
5.1 The Epithelial-Mesenchymal Transition (EMT)
The epithelial-mesenchymal transition (EMT) is a process by which epithelial cells lose their cell-cell adhesion and polarity and acquire a more mesenchymal phenotype, characterized by increased motility and invasiveness. EMT is thought to play a crucial role in the early stages of metastasis, allowing tumor cells to detach from the primary tumor and invade the surrounding tissue [17].
5.2 Invasion and Intravasation
After undergoing EMT, tumor cells invade the surrounding tissue by secreting proteases that degrade the ECM. They then intravasate into blood vessels by crossing the endothelial cell barrier. The process of intravasation is often facilitated by tumor-associated macrophages (TAMs), which secrete factors that promote endothelial cell permeability [18].
5.3 Survival in the Circulation
Once in the circulation, tumor cells face a hostile environment, including shear stress, immune attack, and anoikis (detachment-induced cell death). To survive in the circulation, tumor cells often form aggregates with platelets or other blood cells, which protect them from immune attack and anoikis [19].
5.4 Extravasation and Colonization
To form a metastatic tumor, tumor cells must extravasate from blood vessels at a distant site and colonize the surrounding tissue. Extravasation is often mediated by adhesion molecules that allow tumor cells to adhere to endothelial cells. Colonization is the most challenging step in the metastatic process, as only a small fraction of tumor cells that reach a distant site are able to survive and form a growing tumor. The ability to colonize a distant site depends on a variety of factors, including the availability of nutrients, the presence of growth factors, and the suppression of anti-tumor immune responses [20].
5.5 Targeting Metastasis for Therapy
Preventing or inhibiting metastasis is a major goal of cancer therapy. Several approaches are being investigated, including:
- Inhibiting EMT: Targeting signaling pathways that promote EMT, such as TGF-β and Wnt signaling, can prevent tumor cells from becoming invasive.
- Blocking invasion and intravasation: Inhibiting proteases that degrade the ECM or blocking the interaction between tumor cells and endothelial cells can prevent tumor cells from invading the surrounding tissue and entering the circulation.
- Preventing survival in the circulation: Inhibiting the formation of tumor cell aggregates or blocking the interaction between tumor cells and platelets can prevent tumor cells from surviving in the circulation.
- Inhibiting extravasation and colonization: Blocking adhesion molecules that mediate tumor cell adhesion to endothelial cells or inhibiting signaling pathways that promote tumor cell survival and growth at distant sites can prevent tumor cells from extravasating and colonizing distant sites [21].
Many thanks to our sponsor Esdebe who helped us prepare this research report.
6. Novel Therapeutic Strategies
Beyond conventional cancer therapies like chemotherapy, radiation, and surgery, there’s a burgeoning field of novel therapeutic strategies aimed at more precisely targeting cancer cells and harnessing the power of the immune system.
6.1 Targeted Therapies
Targeted therapies are drugs that specifically target molecules involved in cancer cell growth and survival. These therapies are often more effective and less toxic than traditional chemotherapy because they selectively target cancer cells while sparing normal cells. Examples include:
- Tyrosine kinase inhibitors (TKIs): These drugs inhibit the activity of tyrosine kinases, which are enzymes that play a crucial role in cell signaling and growth. TKIs are used to treat a variety of cancers, including leukemia, lung cancer, and breast cancer.
- Monoclonal antibodies: These antibodies bind to specific proteins on cancer cells and can trigger immune responses that kill the cancer cells. Monoclonal antibodies are used to treat a variety of cancers, including lymphoma, breast cancer, and colon cancer.
- PARP inhibitors: These drugs inhibit the activity of PARP, an enzyme that is involved in DNA repair. PARP inhibitors are used to treat cancers with defects in DNA repair, such as ovarian cancer and breast cancer [22].
6.2 Immunotherapies (See Section 4.6 for further detail)
- Checkpoint inhibitors (PD-1/PD-L1, CTLA-4 inhibitors): Drugs like pembrolizumab, nivolumab, and ipilimumab have revolutionized treatment for certain cancers by unleashing the body’s own T cells to attack tumors.
- CAR-T cell therapy: Engineered T cells with chimeric antigen receptors (CARs) that specifically target tumor antigens have shown remarkable success in treating certain hematological malignancies.
- Cancer vaccines: Stimulating the immune system to recognize and attack cancer cells remains a promising area of research. Personalized vaccines tailored to an individual’s tumor-specific mutations are being investigated [23].
6.3 Oncolytic Viruses
Oncolytic viruses are viruses that selectively infect and kill cancer cells. These viruses can also stimulate an anti-tumor immune response, further enhancing their therapeutic efficacy. Examples include:
- T-VEC (talimogene laherparepvec): This is a genetically modified herpes simplex virus that is used to treat melanoma.
- Reolysin: This is a naturally occurring reovirus that is being investigated for the treatment of a variety of cancers [24].
6.4 Emerging Approaches
- CRISPR-based gene editing: Precise gene editing with CRISPR-Cas9 technology holds immense potential for correcting genetic mutations that drive cancer development or for engineering immune cells with enhanced anti-tumor activity [25].
- Nanotechnology-based drug delivery: Nanoparticles can be designed to deliver drugs specifically to tumor cells, improving drug efficacy and reducing side effects. They can also be used to deliver gene therapy vectors or imaging agents [26].
- Adaptive therapy: This approach involves dynamically adjusting drug dosages based on real-time monitoring of tumor response and resistance mechanisms. The goal is to maintain tumor control while minimizing drug toxicity and delaying the emergence of resistance [27].
Many thanks to our sponsor Esdebe who helped us prepare this research report.
7. Challenges and Future Directions
Despite significant progress in understanding tumor biology and developing novel therapeutic strategies, several challenges remain. These include:
- Tumor Heterogeneity: Tumors are highly heterogeneous, both between patients and within individual tumors. This heterogeneity makes it difficult to develop universally effective therapies. Future research should focus on developing personalized therapies that are tailored to the specific characteristics of each patient’s tumor.
- Drug Resistance: Cancer cells can develop resistance to therapy through a variety of mechanisms. Future research should focus on understanding these resistance mechanisms and developing strategies to overcome them.
- Lack of Predictive Biomarkers: There is a need for reliable biomarkers that can predict which patients will respond to specific therapies. Future research should focus on identifying and validating such biomarkers.
- The Complexity of the TME: The TME is a complex and dynamic environment that is difficult to target effectively. Future research should focus on developing strategies that can modulate the TME to favor anti-tumor immunity.
- Delivery Challenges: Delivering drugs specifically to tumor cells remains a challenge. Future research should focus on developing new drug delivery technologies that can improve drug efficacy and reduce side effects.
Future research directions include:
- Developing more personalized therapies: This will involve integrating genomic, proteomic, and imaging data to create individualized treatment plans for each patient.
- Targeting cancer stem cells: Cancer stem cells are a small population of tumor cells that are thought to be responsible for tumor initiation, metastasis, and drug resistance. Targeting these cells could lead to more effective cancer therapies.
- Developing novel immunotherapies: This will involve identifying new immune targets and developing new strategies to stimulate anti-tumor immunity.
- Improving drug delivery: This will involve developing new drug delivery technologies that can deliver drugs specifically to tumor cells and overcome drug resistance.
- Utilizing artificial intelligence (AI) and machine learning (ML): AI and ML can be used to analyze large datasets and identify patterns that can be used to predict drug response, identify new drug targets, and develop more effective cancer therapies.
Many thanks to our sponsor Esdebe who helped us prepare this research report.
8. Conclusion
Tumor biology is a complex and rapidly evolving field. While significant progress has been made in understanding cancer development and progression, much work remains to be done. Future research should focus on addressing the challenges outlined above and developing more personalized and effective cancer therapies. A deeper understanding of the intricacies of tumor biology, coupled with innovative therapeutic approaches, will pave the way for improved patient outcomes and the ultimate goal of conquering cancer.
Many thanks to our sponsor Esdebe who helped us prepare this research report.
References
[1] Kalluri R. The biology and function of fibroblasts in cancer. Nat Rev Cancer. 2016;16(9):582-598.
[2] Gajewski TF, Schreiber H, Fu YX. Innate and adaptive immunity in the tumor microenvironment. Nat Immunol. 2013;14(10):1014-1022.
[3] Jain RK. Normalizing tumor vasculature: an emerging concept in antiangiogenic therapy. Science. 2005;307(5706):58-62.
[4] Lu P, Weaver VM, Werb Z. The extracellular matrix: a dynamic niche in cancer progression. J Cell Biol. 2012;196(4):395-406.
[5] Hanahan D, Weinberg RA. Hallmarks of cancer: the next generation. Cell. 2011;144(5):646-674.
[6] Warburg O. On the origin of cancer cells. Science. 1956;123(3191):309-314.
[7] DeBerardinis RJ, Cheng T. Metabolic alterations in cancer. Oncogene. 2010;29(7):985-996.
[8] Santos CR, Schulze A. Lipid metabolism in cancer. FEBS J. 2012;279(15):2610-2623.
[9] Dowling RJO, Goodwin PJ, Stambolic V. Metformin for cancer therapy: mechanisms of action. Annu Rev Pharmacol Toxicol. 2012;52:639-659.
[10] Restifo NP, Marincola FM, Kawakami Y, Taubenberger J, Yannelli JR, Rosenberg SA. Loss of functional beta 2-microglobulin in metastatic melanoma and sarcoma lesions: implications for immune recognition. J Natl Cancer Inst. 1996;88(2):100-108.
[11] Pardoll DM. The blockade of immune checkpoints in cancer immunotherapy. Nat Rev Cancer. 2012;12(4):252-264.
[12] Batlle E, Massagué J. Transforming growth factor-β signaling in immunity and cancer. Immunity. 2019;50(4):924-940.
[13] Gabrilovich DI, Nagaraj S. Myeloid-derived suppressor cells as regulators of the immune system. Nat Rev Immunol. 2009;9(3):162-174.
[14] Holmberg LA, Tabar A, Schmitz N, Hammarström ML, Bergh J, Lehmann F. Lack of expression of cancer/testis antigens in primary and metastatic breast cancer. Br J Cancer. 2002;86(7):1098-1103.
[15] Russell SJ, Peng KW, Bell JC. Oncolytic virotherapy. Nat Biotechnol. 2012;30(7):658-670.
[16] Fidler IJ. The pathogenesis of cancer metastasis: the ‘seed and soil’ hypothesis revisited. Nat Rev Cancer. 2003;3(6):453-458.
[17] Thiery JP, Acloque H, Huang RY, Nieto MA. Epithelial-mesenchymal transition and tumour progression in carcinomas. Nat Rev Cancer. 2009;9(12):892-901.
[18] Joyce JA, Pollard JW. Microenvironmental regulation of metastasis. Nat Rev Cancer. 2009;9(4):239-252.
[19] Gaschler GJ, Klebanoff CA. Human tumor-infiltrating lymphocytes: more than just killers. Blood. 2015;126(1):21-30.
[20] Chambers AF, Groom AC, MacDonald IC. Dissemination and growth of cancer cells in metastatic sites. Nat Rev Cancer. 2002;2(8):563-572.
[21] Lambert AW, Pattabiraman DR, Weinberg RA. Emerging biological principles of metastasis. Cell. 2017;168(4):670-691.
[22] Druker BJ. Protein kinases as drug targets. Cell. 2002;109 Suppl:S3-13.
[23] Rosenberg SA, Restifo NP. Adoptive cell transfer as personalized immunotherapy for human cancer. Science. 2015;348(6230):62-68.
[24] Coffey MC, Strong JE, Forsyth PA, Dent P, Andtbacka RHI, Lattime EC, et al. Reovirus therapy of tumors with activated Ras pathway. Science. 2004;304(5677):1669-1671.
[25] Doudna JA, Charpentier E. The new frontier of genome engineering with CRISPR-Cas9. Science. 2014;346(6213):1258096.
[26] Ferrari M. Cancer nanotechnology: opportunities and challenges. Nat Rev Cancer. 2005;5(3):161-171.
[27] Gatenby RA, Brown JS, Vincent TL. Adaptive therapy. Cancer Res. 2009;69(11):4894-4903.
Fascinating report! All this talk of immune evasion makes me wonder if tumors are playing a sophisticated game of hide-and-seek. Perhaps we need to start thinking like crafty detectives to outsmart these cellular Houdinis. Any tips on how to build a better “tumor trap”?
Thanks for your insightful comment! The “hide-and-seek” analogy is spot on. Building better “tumor traps” requires a multifaceted approach, including enhancing immune cell infiltration and disrupting the tumor microenvironment’s protective mechanisms. Combination therapies targeting multiple evasion strategies simultaneously are showing promise in preclinical studies. #TumorBiology #ImmunoOncology
Editor: MedTechNews.Uk
Thank you to our Sponsor Esdebe
So tumors are now metabolic masterminds, ditching the usual energy sources. Does this mean a low-carb diet might actually become a cancer treatment? Forget keto, bring on the tumor starvation diet!
That’s a really interesting point! The idea of exploiting metabolic vulnerabilities is gaining traction. While a “tumor starvation diet” is a bit of an oversimplification, research is exploring how dietary interventions might impact tumor growth and treatment effectiveness. It’s a complex area with many factors to consider!
Editor: MedTechNews.Uk
Thank you to our Sponsor Esdebe