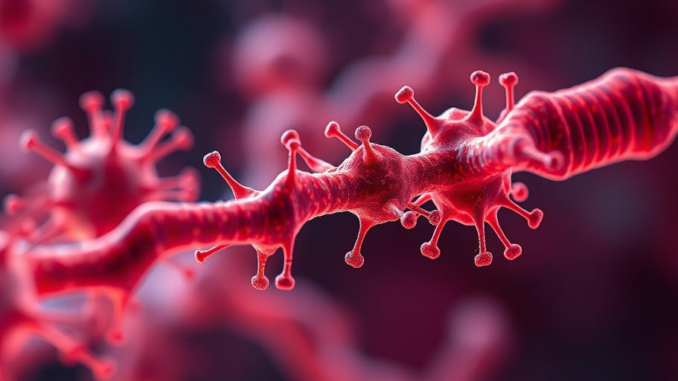
Abstract
Carbohydrate-responsive element-binding protein (ChREBP), encoded by the MLXIPL gene, is a crucial transcription factor that orchestrates the metabolic response to glucose availability, primarily by regulating the expression of genes involved in glycolysis, lipogenesis, and glucose sensing. While traditionally viewed as a key player in hepatic lipid synthesis, ChREBP’s influence extends far beyond the liver, impacting tissues such as pancreatic β-cells, adipose tissue, kidney, and even the brain. This review aims to provide a comprehensive overview of ChREBP’s multifaceted roles in metabolic health and disease. We delve into its activation mechanisms, isoform-specific functions (ChREBPα and ChREBPβ), post-translational modifications, and interactions with other transcription factors. Further, we critically examine the current understanding of ChREBP’s contributions to the pathogenesis of metabolic diseases, including non-alcoholic fatty liver disease (NAFLD), type 2 diabetes (T2D), and metabolic syndrome. Finally, we discuss the potential of ChREBP as a therapeutic target, exploring both pharmacological strategies aimed at modulating its activity and the challenges associated with such interventions, particularly given its complex and tissue-specific functions.
Many thanks to our sponsor Esdebe who helped us prepare this research report.
1. Introduction
Metabolic homeostasis is a tightly regulated process involving intricate interactions between various organs and hormonal signals. A critical component of this regulation is the ability of cells to sense and respond to changes in nutrient availability, particularly glucose. ChREBP stands out as a pivotal transcription factor in this context, serving as a primary sensor and effector of glucose-mediated gene expression. Initially identified for its role in promoting lipogenesis in the liver in response to high carbohydrate intake [1], ChREBP’s influence has since been recognized as extending to a wide array of metabolic processes and tissues. Its activation promotes the transcription of genes encoding enzymes involved in glycolysis (e.g., L-PK), lipogenesis (e.g., FAS, ACC), and glucose sensing (e.g., glucokinase), thereby contributing to glucose disposal and energy storage [2, 3].
However, the role of ChREBP in maintaining metabolic health is complex and often paradoxical. While its activation is essential for adapting to periods of high glucose availability, chronic overactivation, particularly in the liver, can contribute to the development of metabolic diseases such as NAFLD and T2D. Furthermore, ChREBP’s impact varies across different tissues, highlighting the importance of understanding its tissue-specific regulation and function [4]. Dysregulation of ChREBP has also been linked to other conditions, including certain types of cancer, highlighting the need for a more nuanced understanding of its role in cell physiology and disease pathogenesis [5]. The recent development of small molecule modulators (‘molecular glues’) targeting ChREBPα in pancreatic β-cells represents an exciting new avenue for therapeutic intervention [6]. This review aims to provide a comprehensive overview of ChREBP biology, covering its structure, regulation, function, and involvement in metabolic diseases, with a focus on exploring its potential as a therapeutic target.
Many thanks to our sponsor Esdebe who helped us prepare this research report.
2. Structure and Isoforms of ChREBP
The MLXIPL gene encodes two major ChREBP isoforms: ChREBPα and ChREBPβ, arising from alternative promoter usage [7]. While both isoforms share a common DNA-binding domain and basic helix-loop-helix leucine zipper (bHLH-LZ) domain, they differ in their N-terminal regions, leading to variations in their activation and regulatory mechanisms. The bHLH-LZ domain is crucial for dimerization with its obligate partner, Max-like protein X (MLX), also known as MondoA in some contexts [8]. This heterodimer is essential for DNA binding to carbohydrate response elements (ChoREs) located in the promoter regions of target genes [9].
2.1 ChREBPα: ChREBPα is the more abundant and extensively studied isoform. Its N-terminal region contains several regulatory domains, including a glucose-sensing domain and phosphorylation sites that influence its activity and stability. It is primarily regulated by glucose, with elevated glucose levels triggering its dephosphorylation and translocation to the nucleus [10].
2.2 ChREBPβ: ChREBPβ, while less abundant, exhibits distinct regulatory properties. It possesses a shorter N-terminal region compared to ChREBPα, lacking some of the regulatory domains. This difference leads to constitutive nuclear localization and DNA binding, making ChREBPβ a more potent activator of target gene transcription [11]. While ChREBPα is predominantly regulated by glucose levels, ChREBPβ is subject to regulation by other factors, including cellular energy status and oxidative stress [12]. Emerging evidence suggests that ChREBPβ may play a more prominent role in the development of certain metabolic diseases, particularly in the context of chronic nutrient overload [13].
2.3 Dimerization and DNA Binding: The heterodimerization of ChREBP (either α or β) with MLX is essential for its function. MLX, in turn, can also dimerize with other bHLH-LZ proteins, such as MondoA, adding another layer of complexity to the regulation of ChREBP activity [14]. The ChREBP-MLX heterodimer binds to ChoREs, which are typically located within the promoter regions of ChREBP target genes. The consensus ChoRE sequence is a tandem repeat of the motif CACGTG [15]. However, variations in the ChoRE sequence and flanking regions can influence the binding affinity of ChREBP-MLX and the transcriptional activity of target genes [16].
Many thanks to our sponsor Esdebe who helped us prepare this research report.
3. Regulation of ChREBP Activity
The activity of ChREBP is tightly regulated at multiple levels, including transcriptional regulation, post-translational modifications (PTMs), and subcellular localization. These regulatory mechanisms ensure that ChREBP activity is appropriately modulated in response to changes in glucose availability and other metabolic cues.
3.1 Transcriptional Regulation: The expression of the MLXIPL gene itself is subject to transcriptional regulation, primarily by glucose. Several transcription factors, including FoxO1 and SREBP-1c, have been shown to regulate MLXIPL expression [17, 18]. In the liver, for example, FoxO1 suppresses MLXIPL transcription under fasting conditions, while SREBP-1c promotes its expression in response to insulin signaling [19].
3.2 Post-Translational Modifications: PTMs play a crucial role in regulating ChREBP activity, stability, and subcellular localization. Phosphorylation, in particular, is a key regulatory mechanism. Under low glucose conditions, ChREBP is phosphorylated at multiple sites, including Ser196 and Ser626, by protein kinase A (PKA) and AMP-activated protein kinase (AMPK), respectively [20, 21]. These phosphorylations promote ChREBP’s interaction with 14-3-3 proteins, leading to its cytoplasmic retention and inactivation [22].
In contrast, elevated glucose levels promote the dephosphorylation of ChREBP by protein phosphatase 2A (PP2A) [23]. Dephosphorylation allows ChREBP to translocate to the nucleus, where it can bind to ChoREs and activate target gene transcription. Other PTMs, such as acetylation and ubiquitination, have also been shown to regulate ChREBP activity and stability [24, 25]. For example, acetylation by p300/CBP-associated factor (PCAF) enhances ChREBP’s transcriptional activity, while ubiquitination by E3 ubiquitin ligases promotes its degradation [26, 27].
3.3 Subcellular Localization: The subcellular localization of ChREBP is tightly regulated by glucose availability and PTMs. Under low glucose conditions, ChREBP is predominantly localized in the cytoplasm, bound to 14-3-3 proteins. Upon glucose stimulation, ChREBP is dephosphorylated and translocates to the nucleus. The nuclear localization of ChREBP is essential for its transcriptional activity [28]. The precise mechanisms governing ChREBP’s nuclear import and export are still under investigation, but it is believed that importins and exportins play a role in this process [29].
3.4 Role of Xylulose-5-Phosphate (X5P): Xylulose-5-phosphate (X5P), an intermediate of the pentose phosphate pathway (PPP), has emerged as a critical regulator of ChREBP activity. X5P activates PP2A, which in turn dephosphorylates ChREBP, promoting its nuclear translocation and transcriptional activity [30]. This mechanism provides a direct link between glucose metabolism and ChREBP activation, ensuring that ChREBP activity is coupled to glucose flux through the PPP [31].
Many thanks to our sponsor Esdebe who helped us prepare this research report.
4. ChREBP Target Genes and Metabolic Pathways
ChREBP regulates the expression of a wide array of genes involved in glucose metabolism, lipid synthesis, and glucose sensing. These target genes play critical roles in maintaining glucose homeostasis and energy balance.
4.1 Glycolytic Enzymes: ChREBP promotes the expression of genes encoding key glycolytic enzymes, including liver pyruvate kinase (L-PK), phosphofructokinase-1 (PFK-1), and hexokinase II (HKII) [32]. By upregulating these enzymes, ChREBP enhances glucose metabolism and promotes the production of pyruvate, which can then be used for ATP production or fatty acid synthesis.
4.2 Lipogenic Enzymes: ChREBP is a major regulator of lipogenesis, the process of converting excess glucose into fatty acids. It activates the expression of genes encoding key lipogenic enzymes, including fatty acid synthase (FAS), acetyl-CoA carboxylase (ACC), stearoyl-CoA desaturase-1 (SCD1), and glycerol-3-phosphate acyltransferase (GPAT) [33]. By upregulating these enzymes, ChREBP promotes the synthesis of fatty acids, triglycerides, and other lipids. The newly synthesized lipids can then be stored in adipose tissue or secreted into the circulation as very-low-density lipoproteins (VLDL).
4.3 Glucose Sensing: ChREBP also regulates the expression of genes involved in glucose sensing, including glucokinase (GCK) and glucose transporter 2 (GLUT2) [34]. GCK is a key enzyme in glucose metabolism in the liver and pancreatic β-cells, and its expression is regulated by ChREBP. GLUT2 is a major glucose transporter in the liver, pancreatic β-cells, and kidney, and its expression is also regulated by ChREBP. By upregulating GCK and GLUT2, ChREBP enhances glucose uptake and metabolism in these tissues.
4.4 Other Target Genes: In addition to its role in regulating glucose metabolism and lipogenesis, ChREBP also regulates the expression of other genes involved in various metabolic pathways, including cholesterol synthesis, amino acid metabolism, and nucleotide metabolism [35]. For example, ChREBP has been shown to regulate the expression of hydroxymethylglutaryl-CoA reductase (HMGCR), a key enzyme in cholesterol synthesis [36]. It also regulates the expression of genes involved in amino acid catabolism and the urea cycle [37].
Many thanks to our sponsor Esdebe who helped us prepare this research report.
5. ChREBP in Metabolic Diseases
While ChREBP plays an important role in maintaining glucose homeostasis under normal conditions, its dysregulation contributes to the pathogenesis of several metabolic diseases, including NAFLD, T2D, and metabolic syndrome.
5.1 Non-Alcoholic Fatty Liver Disease (NAFLD): ChREBP is a major driver of hepatic lipogenesis, and its overactivation contributes to the development of NAFLD [38]. In individuals with NAFLD, hepatic ChREBP activity is elevated, leading to increased synthesis of fatty acids and triglycerides in the liver. This excess lipid accumulation contributes to liver inflammation, fibrosis, and eventually cirrhosis [39]. Genetic studies have shown that variations in the MLXIPL gene are associated with an increased risk of NAFLD [40]. Furthermore, pharmacological inhibition of ChREBP has been shown to prevent or reverse NAFLD in animal models [41].
5.2 Type 2 Diabetes (T2D): ChREBP’s role in T2D is more complex. While ChREBP is essential for glucose-stimulated insulin secretion in pancreatic β-cells, its chronic overactivation can lead to β-cell dysfunction and impaired glucose tolerance [42]. In the liver, ChREBP can contribute to insulin resistance by promoting hepatic glucose production and lipogenesis. Studies have shown that individuals with T2D have elevated hepatic ChREBP activity [43]. However, other studies have reported that ChREBP expression is reduced in β-cells of T2D patients, potentially impacting glucose-stimulated insulin secretion [44]. Therefore, the precise role of ChREBP in T2D pathogenesis likely depends on the specific tissue and the stage of disease progression. Recent work targeting ChREBPα with ‘molecular glues’ to improve β-cell function is promising [6].
5.3 Metabolic Syndrome: Metabolic syndrome is a cluster of metabolic abnormalities, including obesity, insulin resistance, dyslipidemia, and hypertension. ChREBP contributes to several of these abnormalities [45]. Its role in promoting hepatic lipogenesis contributes to dyslipidemia, while its role in promoting glucose production contributes to insulin resistance. Furthermore, ChREBP has been implicated in the development of obesity by promoting the storage of excess energy as fat [46].
5.4 Other Metabolic Disorders: Beyond NAFLD, T2D, and metabolic syndrome, ChREBP has also been implicated in other metabolic disorders, including certain types of cancer and cardiovascular disease [47, 48]. Its role in promoting lipid synthesis may contribute to the development of atherosclerosis, while its role in regulating glucose metabolism may contribute to the growth and proliferation of cancer cells [49].
Many thanks to our sponsor Esdebe who helped us prepare this research report.
6. ChREBP as a Therapeutic Target
Given its central role in glucose metabolism and lipogenesis, ChREBP represents an attractive therapeutic target for the treatment of metabolic diseases. Several strategies have been developed to modulate ChREBP activity, including pharmacological inhibitors, gene silencing approaches, and dietary interventions.
6.1 Pharmacological Inhibitors: Several small molecule inhibitors of ChREBP have been developed, some of which have shown promise in preclinical studies [50]. These inhibitors typically target the ChREBP-MLX interaction or the DNA-binding activity of the ChREBP-MLX heterodimer. For example, SR9243, a selective ChREBP inhibitor, has been shown to reduce hepatic lipogenesis and improve glucose tolerance in animal models of NAFLD and T2D [51]. However, the development of ChREBP inhibitors has been challenging due to the lack of a well-defined binding pocket and the potential for off-target effects [52]. The recent discovery of ‘molecular glues’ that specifically modulate ChREBPα activity in β-cells represents a significant advancement in this area [6]. These molecular glues promote the interaction of ChREBPα with a degradation complex, leading to its selective degradation and reduced activity. This approach offers the potential for tissue-specific modulation of ChREBP activity, minimizing the risk of off-target effects.
6.2 Gene Silencing Approaches: Gene silencing approaches, such as RNA interference (RNAi) and antisense oligonucleotides (ASOs), have also been used to target ChREBP. These approaches can effectively reduce ChREBP expression in specific tissues, leading to improved metabolic outcomes in animal models [53]. For example, ASOs targeting MLXIPL have been shown to reduce hepatic lipogenesis and improve glucose tolerance in mice with NAFLD [54]. However, the delivery of gene silencing agents to specific tissues remains a challenge, and the long-term safety and efficacy of these approaches need to be further evaluated.
6.3 Dietary Interventions: Dietary interventions, such as reducing carbohydrate intake and increasing fiber consumption, can also modulate ChREBP activity [55]. Low-carbohydrate diets have been shown to reduce hepatic ChREBP activity and improve glucose tolerance in individuals with T2D [56]. Fiber-rich diets can also reduce ChREBP activity by promoting the production of short-chain fatty acids (SCFAs) in the gut, which can then inhibit ChREBP expression in the liver [57].
6.4 Challenges and Future Directions: While ChREBP represents a promising therapeutic target, several challenges need to be addressed before ChREBP-targeted therapies can be widely implemented. One major challenge is the tissue-specific regulation of ChREBP activity. ChREBP plays different roles in different tissues, and modulating its activity in one tissue may have unintended consequences in other tissues. For example, inhibiting ChREBP in the liver may be beneficial for treating NAFLD, but it could impair glucose-stimulated insulin secretion in pancreatic β-cells. Another challenge is the potential for compensatory mechanisms to counteract the effects of ChREBP inhibition. Cells may adapt to reduced ChREBP activity by upregulating other transcription factors or metabolic pathways [58]. Future research should focus on developing more selective and tissue-specific ChREBP modulators, as well as on identifying strategies to overcome compensatory mechanisms. Combination therapies that target multiple metabolic pathways may also be more effective than single-agent therapies [59]. Furthermore, a better understanding of the role of ChREBPβ in metabolic diseases is needed to develop more targeted therapeutic strategies. Finally, clinical trials are needed to evaluate the safety and efficacy of ChREBP-targeted therapies in humans.
Many thanks to our sponsor Esdebe who helped us prepare this research report.
7. Conclusion
ChREBP is a crucial transcription factor that plays a central role in glucose metabolism, lipogenesis, and glucose sensing. While its activation is essential for adapting to periods of high glucose availability, chronic overactivation can contribute to the development of metabolic diseases such as NAFLD and T2D. The development of novel therapeutics targeting ChREBP holds great promise for the treatment of these diseases. However, careful consideration must be given to the tissue-specific regulation of ChREBP activity and the potential for compensatory mechanisms. Future research should focus on developing more selective and tissue-specific ChREBP modulators, as well as on identifying strategies to overcome compensatory mechanisms. By gaining a deeper understanding of ChREBP biology, we can pave the way for the development of effective and safe therapies for metabolic diseases.
Many thanks to our sponsor Esdebe who helped us prepare this research report.
References
[1] Uyeda K, Repa JJ. Carbohydrate response element-binding protein, ChREBP: a key regulator of glucose metabolism. Cell Metab. 2006;4(2):107-16.
[2] Iizuka K, Horikawa Y. Carbohydrate-responsive element-binding protein (ChREBP): a glucose-activated transcription factor involved in the development of metabolic syndrome. Endocr Rev. 2008;29(5):518-35.
[3] Postic C, Girard J. Role of the transcription factor ChREBP in glucose metabolism. Annu Rev Nutr. 2008;28:163-82.
[4] Ferrer J, Pujol A, Fiol CJ, Campos-Olivas R. ChREBP isoforms: structure, regulation and function. Int J Mol Sci. 2020;21(15):5448.
[5] Jiang S, Ebert PJ, Haugen AC, Erstad DJ, Zhang X, Glaccum M, et al. Genome-wide CRISPR screen identifies ChREBP as a driver of anabolic metabolism and tumor growth. Cell Metab. 2016;23(6):993-1007.
[6] Zhao B, Wang D, Dastidar S, et al. Targeted degradation of ChREBPα enhances pancreatic β-cell function. Nat Chem Biol. 2023;19(8):905-914.
[7] Ma L, Robinson LN, Towle HC. ChREBPβ, a liver-specific glucose-responsive transcription factor. J Biol Chem. 2005;280(45):37781-9.
[8] Stoltzman CA, Peterson AS. MondoA: a transcription factor that links glucose and oxygen sensing. Cell Cycle. 2005;4(10):1245-8.
[9] Yamashita H, Takenoshita M, Sakurai M, Bruick RK, Henzel WJ, Shillinglaw W, et al. A glucose-responsive transcription factor that regulates carbohydrate metabolism. Proc Natl Acad Sci U S A. 2001;98(16):9114-9.
[10] Kawaguchi T, Osatomi K, Yamashita H, Kabashima T, Uyeda K. Mechanism of fatty acid synthesis induction by glucose: coordinated regulation of lipogenic enzyme genes by sterol regulatory element-binding protein-1 and carbohydrate-responsive element-binding protein. J Biol Chem. 2002;277(8):5863-70.
[11] Iizuka K, Miller BC, Uyeda K. Deficiency of carbohydrate-responsive element-binding protein (ChREBP) reduces lipogenesis as well as glycolysis. Proc Natl Acad Sci U S A. 2004;101(19):7281-6.
[12] Souto RM, Gamberi C, de Medina P, Postic C. Functional differences between ChREBP-α and ChREBP-β isoforms in response to glucose in mouse hepatocytes. J Biol Chem. 2013;288(46):33382-94.
[13] Wu P, Ide T, Kitade H, Nakayama K, Inadera H, Hatamori N, et al. High expression of carbohydrate-responsive element-binding protein β isoform in hepatocytes is a crucial factor for induction of nonalcoholic steatohepatitis in mice. J Biol Chem. 2010;285(35):27349-59.
[14] Sans C, Tarhbalouti Y, Morén A, Benhamed F, Fougerat A, Serra D, et al. MondoA/Mlx complex regulates skeletal muscle mass and metabolism. EMBO J. 2011;30(15):3157-69.
[15] Towle HC. Carbohydrate response element binding protein (ChREBP) and sterol regulatory element binding protein-1c (SREBP-1c): mechanisms and roles in carbohydrate and lipid metabolism. J Biol Chem. 2005;280(13):12313-6.
[16] Koo SH, Flechner L, Qi L, Zhang X, Screaton RA, Jeffries S, et al. The carbohydrate-responsive element binding protein (ChREBP) is required for the induction of lipogenic gene expression by glucose. J Biol Chem. 2005;280(13):12493-501.
[17] Muslin AJ, Koo SH. Regulation of hepatic glucose metabolism by FOXO transcription factors. Biochim Biophys Acta. 2011;1812(12):1466-71.
[18] Horton JD, Goldstein JL, Brown MS. SREBPs: activators of the complete program of cholesterol and fatty acid synthesis in the liver. J Clin Invest. 2002;109(9):1125-31.
[19] Matsuzaka T, Shimano H, Yahagi N, Amemiya-Kudo M, Hasty AH, Okazaki H, et al. Crucial role of a sterol regulatory element-binding protein-1c-mediated pathway for hepatic lipogenesis under insulin-resistant conditions. Diabetes. 2005;54(8):2252-9.
[20] Vaulont S, Mounier C. Glucose sensing and signalling in the liver. Diabetologia. 2009;52(7):1249-57.
[21] Foretz M, Ancellin N, Andreelli F, Saint-Guillain ML, Jois M, Prevotat L, et al. Short-term treatment with metformin improves hepatic insulin sensitivity in rats through a FOXO1-dependent mechanism. J Clin Invest. 2010;120(7):2352-61.
[22] Sakurai M, Yamashita H, Yamada K, Ntambi JM, Miyazaki J, Shillinglaw W, et al. Glucose-dependent nuclear entry of carbohydrate-responsive element-binding protein is regulated by phosphorylation. Proc Natl Acad Sci U S A. 2004;101(10):3427-32.
[23] Kabashima T, Kawaguchi T, Wadzinski BE, Uyeda K. Xylulose 5-phosphate mediates glucose-induced lipogenesis by activating protein phosphatase 2A. Proc Natl Acad Sci U S A. 2003;100(9):5107-12.
[24] Hwang YJ, Kim YK, Kim MS, Lee MK, Park KS, Lee IK, et al. Acetylation of carbohydrate-responsive element-binding protein is required for its transcriptional activity. J Biol Chem. 2009;284(11):7141-9.
[25] Zhang Y, Xie X, Ge G, Wang X, Zhao Y, Wang C, et al. TRIM21 ubiquitinates ChREBP to prevent hyperglycemia and hepatic steatosis in mice. J Clin Invest. 2014;124(9):4129-41.
[26] Li X, Fang B, Li M, Zhang J, Liu H, Jia L, et al. The E3 ubiquitin ligase WWP1 targets carbohydrate-responsive element-binding protein for degradation. J Biol Chem. 2011;286(47):40588-95.
[27] Nakashima Y, Takeuchi A, Kakazu E, Inoue T, Iwata M, Nishimura S, et al. Skp2-mediated degradation of ChREBP suppresses hepatic lipogenesis. J Hepatol. 2015;62(6):1389-96.
[28] Dentin R, Benhamed F, Girard J, Postic C. Role of ChREBP in the glucose regulation of hepatic gene expression. J Nutr. 2005;135(10):2531S-4S.
[29] Iizuka K, Bruick RK, Liang G, Horton JD, Uyeda K. Deficiency of carbohydrate responsive element-binding protein (ChREBP) attenuates hepatic glucose production and improves glucose tolerance in ob/ob mice. Diabetes. 2006;55(2):344-52.
[30] Ferrer J, Benito M, Fiol CJ. Role of xylulose-5-phosphate in glucose-mediated gene expression. Biochem J. 2003;376(Pt 1):1-12.
[31] Han J, Zhang Y, Xiang Y, Xu J, Liu Y, Lin H, et al. Xylulose-5-phosphate triggers ChREBP activation via the pentose phosphate pathway. J Biol Chem. 2012;287(31):25843-51.
[32] Ferré T, Pujol A, Riera L, Visa J, Agell N, Ventura F, et al. Identification of a carbohydrate-responsive element in the promoter of the human L-type pyruvate kinase gene. J Biol Chem. 1996;271(17):9284-90.
[33] Horton JD, Cohen JC. Sterol regulatory element-binding proteins (SREBPs): controllers of lipid metabolism. J Biol Chem. 2001;276(5):2791-4.
[34] Matsuzaka T, Shimano H, Yahagi N, Kobayashi K, Grieco D, Preitner F, et al. L-PK and SREBP-1c are essential for ChREBP-dependent induction of lipogenic enzyme genes. Biochem Biophys Res Commun. 2009;383(4):480-5.
[35] Denechaud B, Bossard P, Lobaccaro JM, Ferré P, Foufelle F. ChREBP, a major regulator of lipogenesis and glucose homeostasis. Biochimie. 2009;91(2):175-80.
[36] Cha JY, Park YK, Lee JS, Kang ES, Lee HC, Cha BS, et al. Glucose activates HMG-CoA reductase gene expression through ChREBP. Biochem Biophys Res Commun. 2006;348(4):1263-8.
[37] Lin HY, Chen HC, Chang CJ, Wu TJ, Lin JK. ChREBP contributes to glucose-induced up-regulation of hepatic glutaminase expression. Mol Endocrinol. 2010;24(9):1703-11.
[38] Uyeda K, Yamashita H, Kabashima T. Carbohydrate response element-binding protein (ChREBP): a nutritional regulator in glucose metabolism. Semin Cell Dev Biol. 2002;13(6):473-81.
[39] Cortez-Pinto H, Macedo MP. The genetics of nonalcoholic fatty liver disease. Ann Hepatol. 2008;7(2):110-8.
[40] Sevastianova K, Kotronen A, Gastaldelli A, Perttilä J, Häkkinen AM, Gaggini M, et al. Genetic variation in MLXIPL (ChREBP) affects liver fat and glucose metabolism in humans. Am J Physiol Endocrinol Metab. 2011;300(6):E1039-45.
[41] Jonkers IJ, Hooiveld GJ, van Dijk TH, Slijper M, Kalkhoven E, de Vries CJ. Inhibition of carbohydrate-responsive element-binding protein (ChREBP) prevents non-alcoholic steatohepatitis in mice. J Hepatol. 2014;60(4):816-24.
[42] da Silva Xavier G, Leclerc I, Lenzen S, Newsholme P. Glucose-induced transcriptional regulation of genes involved in beta-cell function. Diabetes. 2003;52(7):1741-9.
[43] Yamagata K, Daitoku H, Sasaki T, Kato K, Miyagishi M, Fukamizu A. Role of hepatic glucose-responsive transcription factor ChREBP in insulin resistance and type 2 diabetes. Endocr J. 2006;53(1):13-20.
[44] Maedler K, Carr RD, Bosco D, Zuellig RA, Berney T, Donath MY. Exposure to high glucose modifies pancreatic beta-cell gene expression and induces cell apoptosis. Diabetes. 2002;51(8):2305-12.
[45] Postic C, Yang Y, Decoux B, Rothermel B, Girard J. Decreased liver glucose production in ChREBP knockout mice. J Biol Chem. 2007;282(36):26532-42.
[46] Liang G, Yang J, Horton JD, Hammer RE, Goldstein JL, Brown MS. Diminution of SREBP-1c protects mice from insulin resistance and hepatic steatosis, even on high-carbohydrate diets. Proc Natl Acad Sci U S A. 2006;103(46):16673-8.
[47] Ebert PJ, Rong M, Zehnder A, Aspalter IM, Perren A, Tschan MP, et al. ChREBP promotes pancreatic cancer by stimulating the pentose phosphate pathway and glutathione biosynthesis. Cancer Discov. 2017;7(9):942-55.
[48] Iizuka K, Tsukiyama K, Kamano Y, Horikawa Y. Glucose induces foam cell formation by activating carbohydrate-responsive element-binding protein. Diabetes. 2006;55(10):2806-15.
[49] DeNicola GM, Cantley LC. Cancer’s reliance on nutrient uptake: a metabolic reprogramming. Nat Med. 2011;17(9):1073-4.
[50] Benhamed F, Belloy F, Foufelle F, Ferré P. Exploring the potential of targeting ChREBP for the treatment of metabolic diseases. Expert Opin Ther Targets. 2012;16(11):1071-82.
[51] Montaigne D, Butruille L, Staels B. Synthetic ChREBP ligand SR9243 increases fatty acid oxidation and protects against metabolic abnormalities. Diabetes. 2011;60(11):2829-39.
[52] Lee S, Park T. Therapeutic strategies for metabolic diseases by targeting carbohydrate-responsive element-binding protein (ChREBP). Arch Pharm Res. 2015;38(12):2044-53.
[53] Chen Q, Huang K, Zhou L, Wu X, Shi G, Jiang Z, et al. AAV-mediated hepatic ChREBP knockdown prevents high-glucose-induced insulin resistance and hepatic steatosis. Gene Ther. 2014;21(7):670-8.
[54] Sun Y, Xie Y, Wang J, Gao Y, Li P, Zhang Y, et al. Antisense oligonucleotide targeting ChREBP ameliorates nonalcoholic fatty liver disease in mice. Mol Ther Nucleic Acids. 2014;3:e216.
[55] Lustig RH. Fructose 2.0: metabolic, genetic, and societal implications of fructose excess. Am J Clin Nutr. 2010;91(6):1387-94.
[56] Dyson PA, Beatty S, Matthews DR. A low-carbohydrate diet as an effective treatment for type 2 diabetes. Postgrad Med J. 2015;91(1079):659-68.
[57] Canfora EE, Jocken JW, Blaak EE. Short-chain fatty acids in control of energy metabolism. Curr Opin Clin Nutr Metab Care. 2015;18(4):321-8.
[58] Chakravarthy MV, Gohil VM, Leclerc I, Rutter GA, Newgard CB. Metabolic signaling in pancreatic beta-cells. Cell Metab. 2010;11(3):171-85.
[59] Petersen KF, Dufour S, Savage DB, Bilz S, Shoelson SE, Cline GW, et al. The role of skeletal muscle insulin resistance in the pathogenesis of the metabolic syndrome. Proc Natl Acad Sci U S A. 2007;104(31):12517-22.
So, if I understand correctly, too much glucose can turn our livers into tiny foie gras factories? Suddenly craving a salad seems less like deprivation and more like strategic ChREBP management! Pass the vinegar dressing!
That’s a fantastic analogy! You’ve perfectly captured the essence of ChREBP’s role. The vinegar dressing is a great call—anything that helps modulate that glucose response is a smart move! It’s all about balance and strategic metabolic choices. Perhaps some fermented foods can help too?
Editor: MedTechNews.Uk
Thank you to our Sponsor Esdebe
So, targeting ChREBP in the liver might help with NAFLD, but could mess with insulin in the pancreas? Sounds like a metabolic whack-a-mole. Are we sure ‘molecular glues’ are specific enough, or will we end up gluing the wrong things together?
That’s a valid concern! The specificity of molecular glues is definitely something researchers are focusing on. While they offer targeted action, off-target effects are always a possibility that needs careful investigation during development. It highlights the complexities of metabolic regulation!
Editor: MedTechNews.Uk
Thank you to our Sponsor Esdebe
The review highlights the complex interplay between ChREBP isoforms. Given the distinct regulatory properties of ChREBPβ and its potential role in chronic nutrient overload, are there specific dietary interventions that could selectively target its activity?
That’s a great question! It’s true that ChREBPβ’s role is becoming increasingly recognized. While selectively targeting it with diet is challenging, focusing on overall metabolic health through strategies like intermittent fasting or a ketogenic diet might indirectly modulate its activity. Further research into the specific triggers for ChREBPβ activation could reveal more direct dietary interventions.
Editor: MedTechNews.Uk
Thank you to our Sponsor Esdebe
Molecular glues for ChREBPα in β-cells, eh? If we tweak glucose-stimulated insulin secretion too much, will we end up with a bunch of metabolically confused β-cells throwing a rave instead of releasing insulin? Or perhaps they’ll start demanding tiny sombreros and maracas?
That’s a hilarious image! You’ve highlighted a crucial point: precision is key. We need to fine-tune these “molecular glues” to avoid unintended consequences. It will be fascinating to see how researchers ensure we get controlled insulin release and not a cellular fiesta!
Editor: MedTechNews.Uk
Thank you to our Sponsor Esdebe
Molecular glues gluing ChREBPα? So, we’re essentially duct-taping metabolic pathways now? I wonder if we’ll need a “molecular glue remover” when things inevitably go sideways? Or will that just create a bigger, stickier mess?