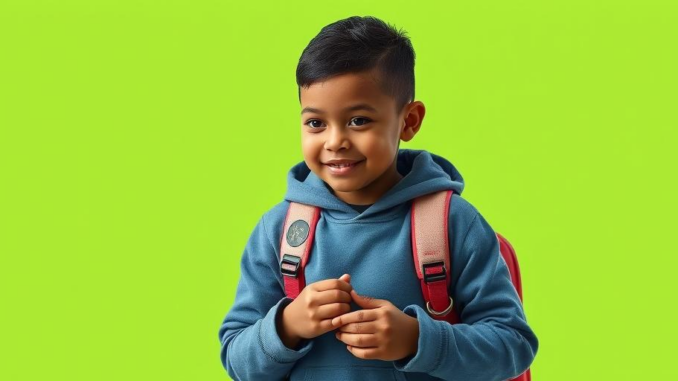
Wearable Technology in Pediatric Healthcare: A Landscape Overview, Challenges, and Future Directions
Many thanks to our sponsor Esdebe who helped us prepare this research report.
Abstract
Wearable technology has rapidly evolved from consumer electronics to a significant player in healthcare, offering continuous, non-invasive physiological monitoring and real-time data collection. This research report provides a comprehensive overview of wearable technology within the context of pediatric care, examining the diverse types of wearable sensors, their specific applications in addressing pediatric health challenges, and the associated ethical, regulatory, and security concerns. While exemplifying innovative applications like the MAIJU jumpsuit, we focus on a broader view, exploring the future potential of integrating wearables with artificial intelligence (AI) and telehealth platforms to revolutionize pediatric healthcare delivery and improve patient outcomes. The report further discusses the key challenges in the adoption of wearable technology, including data privacy, usability, and the need for robust validation studies, and offers recommendations for future research and development.
Many thanks to our sponsor Esdebe who helped us prepare this research report.
1. Introduction
The proliferation of wearable technology has brought about a paradigm shift in healthcare, enabling continuous monitoring of physiological parameters, facilitating early detection of anomalies, and empowering patients with greater control over their health management. Unlike traditional clinical assessments, wearables offer the advantage of real-time, longitudinal data capture in real-world settings, providing a more holistic view of a patient’s health status. While the adult population has been the primary focus of wearable technology development and application, the pediatric population presents a unique set of opportunities and challenges. Children have distinct physiological characteristics and developmental trajectories, requiring tailored wearable designs and analytical methods. The MAIJU jumpsuit, for instance, demonstrates the potential of wearable technology to address specific pediatric needs by providing continuous monitoring in a comfortable and non-intrusive manner. However, a systematic overview of the broader landscape of wearable technology in pediatric care is crucial to identify key areas of innovation, address potential limitations, and guide future research and development. This report aims to provide such an overview, encompassing the types of wearable sensors, their applications, associated challenges, and future directions in the field.
Many thanks to our sponsor Esdebe who helped us prepare this research report.
2. Types of Wearable Sensors and Technologies
Wearable technology encompasses a diverse range of devices and sensors designed to be worn on the body, enabling continuous data collection and analysis. In the context of pediatric care, the choice of wearable technology depends on the specific application and the needs of the patient. The following are some key types of wearable sensors and technologies used in pediatric healthcare:
2.1. Physiological Sensors: These sensors measure vital signs and physiological parameters, providing insights into a child’s health status. Common types include:
- Electrocardiography (ECG) sensors: Measure electrical activity of the heart to monitor heart rate, rhythm, and detect arrhythmias. Wearable ECG monitors are used for children with congenital heart defects, arrhythmias, or those undergoing cardiac rehabilitation. Patch-based ECG sensors are generally better accepted by younger children.
- Photoplethysmography (PPG) sensors: Use light to measure blood volume changes in peripheral tissues, providing information on heart rate, oxygen saturation (SpO2), and blood pressure. PPG sensors are commonly integrated into wrist-worn devices and are used to monitor vital signs during sleep or physical activity.
- Accelerometers and Gyroscopes: Detect movement and orientation, providing information on physical activity levels, sleep patterns, and posture. These sensors are widely used in pedometers and fitness trackers and can be helpful in monitoring children with motor impairments or movement disorders.
- Temperature sensors: Measure body temperature, providing an indication of fever or other thermal abnormalities. Wearable temperature sensors can be particularly useful for monitoring infants and young children who may not be able to communicate their symptoms effectively.
- Electrodermal Activity (EDA) sensors: Measure changes in skin conductance, which are related to sympathetic nervous system activity. EDA sensors can be used to monitor stress levels, anxiety, and emotional responses in children.
- Respiratory sensors: Measure respiratory rate and patterns, detecting signs of respiratory distress or sleep apnea. These sensors can be integrated into chest straps or nasal cannulas and are used to monitor children with asthma, cystic fibrosis, or other respiratory conditions. Novel approaches involving impedance pneumography and inductive plethysmography are gaining traction for accurate respiratory monitoring.
2.2. Environmental Sensors: These sensors monitor environmental factors that can impact a child’s health, such as:
- Air quality sensors: Measure levels of pollutants such as particulate matter, ozone, and nitrogen dioxide. Wearable air quality monitors can be used to assess a child’s exposure to environmental hazards and inform preventive measures.
- Ultraviolet (UV) radiation sensors: Measure the intensity of UV radiation, providing information on sun exposure and the risk of sunburn. These sensors can be integrated into wristbands or clothing and are particularly useful for children with photosensitivity.
- Ambient temperature and humidity sensors: Monitor environmental conditions that can affect a child’s comfort and health, such as overheating or dehydration.
2.3. Smart Textiles: These are fabrics integrated with electronic components, such as sensors, actuators, and communication modules. Smart textiles offer the advantage of being comfortable, discreet, and washable, making them well-suited for pediatric applications. Examples include:
- ECG-integrated shirts: Monitor heart activity without the need for adhesive electrodes.
- Respiration-sensing vests: Measure respiratory rate and patterns.
- Activity-tracking socks: Monitor foot movements and activity levels in infants and young children.
2.4. Biometric Identification: Using methods such as Fingerprint scanning, facial recognition, and voice recognition. These methods are being used to track patient identity and provide security.
2.5. Data Transmission and Storage: Wearable sensors typically transmit data wirelessly to a smartphone, tablet, or computer for analysis and storage. Common communication protocols include Bluetooth, Wi-Fi, and cellular networks. Data security and privacy are paramount, requiring robust encryption and access control mechanisms.
Many thanks to our sponsor Esdebe who helped us prepare this research report.
3. Applications of Wearable Technology in Pediatric Care
Wearable technology has the potential to transform pediatric healthcare across a wide range of applications. The following are some key areas where wearables are making a significant impact:
3.1. Chronic Disease Management: Wearables can play a crucial role in managing chronic conditions such as asthma, diabetes, and epilepsy. Continuous monitoring of physiological parameters can provide early warning signs of exacerbations, allowing for timely intervention and prevention of hospitalizations.
- Asthma: Wearable sensors can monitor respiratory rate, lung sounds, and environmental triggers to help children with asthma manage their condition effectively. For instance, devices can detect wheezing or coughing episodes and provide alerts to administer medication.
- Diabetes: Continuous glucose monitors (CGMs) are a type of wearable sensor that measures blood glucose levels in real-time, eliminating the need for finger pricks. CGMs can be integrated with insulin pumps to automatically deliver insulin based on glucose levels, improving glycemic control and reducing the risk of complications.
- Epilepsy: Wearable EEG monitors can detect seizure activity and provide alerts to caregivers, allowing for timely intervention and preventing injuries. These devices can also be used to monitor the effectiveness of anti-epileptic medications and personalize treatment plans.
3.2. Neonatal Care: Wearable technology is particularly valuable in neonatal care, where continuous monitoring of vital signs is essential for detecting early signs of distress. Wearable sensors can be used to monitor heart rate, respiratory rate, oxygen saturation, and temperature in premature infants and newborns. These devices can also be used to detect apnea episodes and provide alerts to nurses.
3.3. Behavioral Health: Wearable sensors can be used to monitor activity levels, sleep patterns, and physiological responses in children with behavioral health conditions such as autism spectrum disorder (ASD), attention-deficit/hyperactivity disorder (ADHD), and anxiety disorders. This data can provide valuable insights into a child’s behavior and emotional state, helping clinicians to develop personalized treatment plans. EDA sensors, in particular, have shown promise in monitoring stress levels and anxiety in children with ASD.
3.4. Sports Medicine: Wearable sensors are widely used in sports medicine to monitor athletes’ performance, prevent injuries, and track rehabilitation progress. Accelerometers and gyroscopes can measure movement patterns and identify risk factors for injuries. Heart rate monitors can track exertion levels and provide feedback on training intensity. GPS trackers can monitor distance traveled and speed during training sessions.
3.5. Sleep Monitoring: Wearable sensors can be used to monitor sleep patterns and detect sleep disorders such as insomnia, sleep apnea, and restless legs syndrome. Actigraphy, using accelerometers, is a common method for assessing sleep duration and quality. More advanced wearable sleep monitors can also measure heart rate, respiratory rate, and oxygen saturation.
3.6. Remote Patient Monitoring: Wearable technology enables remote patient monitoring, allowing healthcare providers to track patients’ health status from a distance. This is particularly valuable for children with chronic conditions who require frequent monitoring but may not be able to visit the clinic regularly. Remote patient monitoring can improve access to care, reduce healthcare costs, and empower patients to manage their own health.
Many thanks to our sponsor Esdebe who helped us prepare this research report.
4. Data Privacy, Security, and Ethical Considerations
The widespread adoption of wearable technology in pediatric care raises significant data privacy, security, and ethical concerns. These concerns must be addressed to ensure that wearable technology is used responsibly and ethically.
4.1. Data Privacy: Wearable sensors collect sensitive personal information, including physiological data, location data, and behavioral data. This data must be protected from unauthorized access, use, or disclosure. Strong data encryption and access control mechanisms are essential to ensure data privacy. It is also important to obtain informed consent from parents or guardians before collecting and using data from wearable sensors.
4.2. Data Security: Wearable devices and data transmission channels are vulnerable to security breaches. Hackers could potentially gain access to sensitive patient data, which could be used for malicious purposes. Robust security measures, such as firewalls, intrusion detection systems, and regular security audits, are necessary to protect wearable devices and data from cyberattacks.
4.3. Data Ownership and Control: It is important to clarify who owns the data collected by wearable sensors and who has the right to access and use the data. In the context of pediatric care, parents or guardians typically have the right to access and control their child’s data. However, it is important to involve children in decisions about data sharing and usage, especially as they mature.
4.4. Algorithmic Bias: The algorithms used to analyze data from wearable sensors may be biased, leading to inaccurate or unfair predictions. It is important to carefully evaluate algorithms for bias and ensure that they are fair and equitable across different populations.
4.5. Data Accuracy and Validation: Wearable sensors are not always accurate, and the data they collect may be affected by various factors, such as sensor placement, skin type, and environmental conditions. It is important to validate the accuracy of wearable sensors and to interpret data with caution. Clinical validation studies are essential to determine the reliability and validity of wearable data.
4.6. Ethical Implications of AI Integration: The integration of wearable technology with AI raises additional ethical concerns, such as the potential for automated decision-making and the lack of transparency in AI algorithms. It is important to ensure that AI algorithms are used ethically and responsibly and that humans remain in control of critical decisions.
4.7. Patient Autonomy and Informed Consent: The use of wearable technology should not compromise patient autonomy. Children and their families should be fully informed about the potential benefits and risks of wearable technology and should have the right to refuse to use it. Obtaining informed consent from parents or guardians is essential, and it is important to involve children in the decision-making process as they mature.
Many thanks to our sponsor Esdebe who helped us prepare this research report.
5. Regulatory Considerations
The regulation of wearable technology in healthcare is an evolving landscape, with different regulatory bodies having jurisdiction over different aspects of wearable devices and data. In the United States, the Food and Drug Administration (FDA) regulates wearable devices that are marketed as medical devices. The FDA requires that medical devices meet certain safety and efficacy standards before they can be sold to the public.
5.1. FDA Regulation: The FDA classifies wearable devices based on their intended use and risk level. Class I devices are considered low-risk and are subject to general controls. Class II devices are considered moderate-risk and are subject to special controls, such as performance standards and labeling requirements. Class III devices are considered high-risk and require premarket approval.
5.2. HIPAA Compliance: The Health Insurance Portability and Accountability Act (HIPAA) regulates the privacy and security of protected health information (PHI). Healthcare providers and their business associates must comply with HIPAA regulations when using wearable technology to collect and transmit PHI.
5.3. Data Protection Regulations: Other data protection regulations, such as the General Data Protection Regulation (GDPR) in Europe, may also apply to wearable technology in healthcare. These regulations require organizations to obtain informed consent from individuals before collecting and processing their personal data.
5.4. Liability Considerations: The use of wearable technology in healthcare raises liability considerations. Healthcare providers may be liable for harm caused by inaccurate data from wearable sensors or by errors in the interpretation of wearable data. Manufacturers of wearable devices may be liable for defects in their products.
5.5. Standardization and Interoperability: The lack of standardization and interoperability in wearable technology is a major challenge. Different wearable devices may use different data formats and communication protocols, making it difficult to integrate data from different sources. Standardization efforts are needed to promote interoperability and facilitate data sharing.
Many thanks to our sponsor Esdebe who helped us prepare this research report.
6. Future Trends and Directions
The field of wearable technology in pediatric care is rapidly evolving, with several exciting trends and directions emerging:
6.1. Miniaturization and Integration: Wearable sensors are becoming smaller, more comfortable, and more integrated into clothing and accessories. This trend will make wearables more appealing to children and improve their adherence to monitoring protocols.
6.2. Advanced Sensor Technologies: New sensor technologies are being developed to measure a wider range of physiological parameters, such as biomarkers in sweat and saliva. These advanced sensors will provide more comprehensive insights into a child’s health status.
6.3. Artificial Intelligence (AI) Integration: AI is being used to analyze data from wearable sensors and to provide personalized recommendations to patients and healthcare providers. AI algorithms can detect patterns in data that would be difficult for humans to identify, and they can adapt to individual patients’ needs.
6.4. Telehealth Integration: Wearable technology is being integrated with telehealth platforms to enable remote patient monitoring and virtual consultations. This integration will improve access to care, reduce healthcare costs, and empower patients to manage their own health.
6.5. Personalized Medicine: Wearable technology is playing a key role in personalized medicine, allowing healthcare providers to tailor treatment plans to individual patients’ needs. Data from wearable sensors can be used to identify patients who are at high risk for certain conditions and to develop targeted interventions.
6.6. Gamification and Engagement: Gamification techniques are being used to make wearable technology more engaging and motivating for children. By turning health monitoring into a game, children are more likely to adhere to monitoring protocols and to adopt healthy behaviors.
6.7. Focus on Mental Health: Future advancements will likely see a greater focus on using wearable technology to address mental health challenges in children and adolescents, including stress management, anxiety reduction, and early detection of mood disorders.
Many thanks to our sponsor Esdebe who helped us prepare this research report.
7. Conclusion
Wearable technology holds immense promise for transforming pediatric healthcare, offering the potential for continuous monitoring, early detection of anomalies, and personalized treatment plans. However, the successful adoption of wearable technology in pediatric care requires careful consideration of data privacy, security, ethical considerations, and regulatory requirements. Future research and development should focus on addressing these challenges, developing advanced sensor technologies, integrating AI and telehealth platforms, and personalizing wearable solutions to meet the unique needs of children. By embracing a responsible and ethical approach to wearable technology, we can unlock its full potential to improve the health and well-being of children worldwide.
Many thanks to our sponsor Esdebe who helped us prepare this research report.
References
- Chan, M., Estève, D., Fourniols, J. Y., Escriba, C., & Campo, E. (2012). Smart wearable systems: Current status and future challenges. Artificial Intelligence in Medicine, 56(3), 137-156.
- Chiang, M. F., Andes, L. J., & Vanderveen, J. A. (2017). Telemedicine in the era of health care reform. JAMA pediatrics, 171(4), 301-302.
- Dunn, J., Runge, R., & Snyder, M. (2018). Wearable sensors for human health monitoring. IEEE pulse, 9(5), 35-42.
- Gualtieri, A. P., & Rosenbaum, D. (2016). An overview of wearable technology in the medical field. Journal of Biomedical Engineering, 5(3), 1-7.
- Israel, A. C., Johnson, A. M., & Steele, R. G. (2015). Mobile health for pediatric obesity: Current evidence, challenges, and future directions. Current obesity reports, 4(2), 222-230.
- Kumar, S., Nilsen, W., Abernethy, A., Atienza, J., Patrick, K., Pavel, M., … & Spring, B. (2013). Mobile health technology for better health. IEEE pervasive computing, 12(4), 22-29.
- Luprano, J., Puiatti, A., Masiero, M., Mazzini, L., Ferrarin, M., & Signorini, M. G. (2022). Innovative Wearable Technology for Remote and Ecological Assessment in Children with Neurological Disorders: A Review. Sensors, 22(10), 3920.
- Martínez-Pérez, B., De la Torre-Díez, I., López-Coronado, M., Herreros-González, J., & Consuegra-García, L. (2013). Mobile health applications for the most prevalent diseases. JMIR mHealth and uHealth, 1(2), e24.
- Patel, S., Park, H., Bonato, P., Chan, L., & Rodgers, M. (2012). A review of wearable sensors and systems with application in rehabilitation. Journal of neuroengineering and rehabilitation, 9(1), 21.
- Pioggia, G., Guzzetta, A., Baldassari, S., Cecchi, F., Ahluwalia, B. S., & Dario, P. (2017). Wearable systems for monitoring and early detection of abnormal motor patterns in infants at risk for cerebral palsy. Frontiers in neuroscience, 11, 274.
- World Health Organization. (2018). mHealth: Use of mobile technologies for public health. World Health Organization.
The MAIJU jumpsuit sounds like something straight out of a sci-fi film! I wonder, beyond continuous monitoring, could these wearables also deliver targeted therapies or micro-interventions based on the data collected? Imagine a smart jumpsuit that dispenses a calming scent during a tantrum.
That’s a fantastic point! The potential for closed-loop systems, where wearables not only monitor but also proactively respond with micro-interventions, is really exciting. Imagine personalized scent therapy or even gentle haptic feedback during moments of distress. The future of pediatric wearables is full of possibilities!
Editor: MedTechNews.Uk
Thank you to our Sponsor Esdebe
The discussion around data privacy is critical. As these technologies become more integrated, how do we ensure children understand data usage and have a voice in decisions about their own information, especially as they grow older?
That’s such a vital question! Building digital literacy and giving children agency over their data is paramount. Perhaps incorporating age-appropriate educational modules within the wearable tech interfaces themselves could be a proactive step? This would empower them to learn and make informed choices about their data as they mature.
Editor: MedTechNews.Uk
Thank you to our Sponsor Esdebe