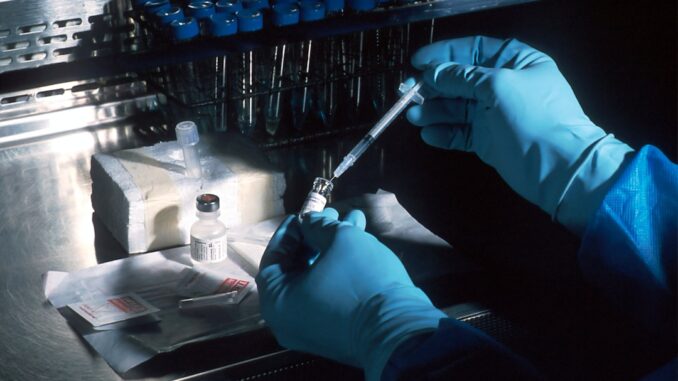
Abstract
Precision medicine, also known as personalized medicine, represents a paradigm shift in healthcare that aims to tailor medical treatment to the individual characteristics of each patient. This approach utilizes genetic information, lifestyle factors, and environmental exposures to optimize treatment efficacy and minimize adverse effects. While the potential benefits of precision medicine are immense, significant challenges remain in its implementation, including data integration, ethical considerations, and cost-effectiveness. This report provides a comprehensive overview of the current state of precision medicine, examining its applications across various disease areas, discussing the challenges associated with its implementation, and exploring potential future directions for this rapidly evolving field. We explore the methodological approaches, including omics technologies and computational modeling, that are driving progress. We then assess the real-world impact through analysis of clinical trials and implementation studies. Furthermore, the ethical, legal, and social implications of precision medicine are addressed, alongside discussions on health equity and data privacy. The report concludes by outlining key research priorities and strategies for accelerating the translation of precision medicine into routine clinical practice.
Many thanks to our sponsor Esdebe who helped us prepare this research report.
1. Introduction
The traditional “one-size-fits-all” approach to medicine often fails to adequately address the heterogeneity of disease presentation and treatment response across individuals. Precision medicine offers a more refined approach, recognizing that individual variability plays a critical role in determining health outcomes. This variability stems from a complex interplay of genetic predisposition, environmental factors, and lifestyle choices. By integrating these factors into clinical decision-making, precision medicine aims to deliver the right treatment, to the right patient, at the right time.
Precision medicine is not a new concept; however, advances in genomics, bioinformatics, and computational technologies have dramatically accelerated its development and implementation. The completion of the Human Genome Project in 2003 provided a foundational understanding of the human genome, paving the way for large-scale genomic sequencing and analysis. These technologies have enabled the identification of genetic variants associated with disease risk, drug response, and other clinically relevant phenotypes. Moreover, the development of sophisticated computational models has facilitated the integration of multi-omic data (genomics, transcriptomics, proteomics, metabolomics) to provide a holistic view of individual disease biology.
The goal of precision medicine is to enhance the accuracy of diagnosis, prognosis, and treatment selection. By identifying individuals at high risk for a particular disease, preventative measures can be implemented to reduce disease incidence. Furthermore, precision medicine can guide the selection of the most effective treatment regimen for a given patient, minimizing the likelihood of treatment failure and adverse drug reactions. Ultimately, precision medicine has the potential to improve patient outcomes, reduce healthcare costs, and advance our understanding of fundamental disease mechanisms.
Many thanks to our sponsor Esdebe who helped us prepare this research report.
2. Methodological Approaches in Precision Medicine
Precision medicine relies on a diverse array of methodological approaches, including omics technologies, bioinformatics, and computational modeling. Each of these approaches plays a crucial role in generating, analyzing, and interpreting the complex data required for personalized treatment strategies.
2.1. Omics Technologies
Omics technologies encompass a suite of high-throughput techniques that allow for the comprehensive analysis of biological molecules within a given sample. These technologies include:
-
Genomics: Genomics focuses on the analysis of the entire genome, including DNA sequence variations, structural variants, and epigenetic modifications. Genome-wide association studies (GWAS) are a powerful tool for identifying genetic variants associated with disease risk and treatment response [1]. Whole-exome sequencing (WES) and whole-genome sequencing (WGS) provide comprehensive information on all coding and non-coding regions of the genome, respectively. These technologies are particularly useful for identifying rare genetic variants that may contribute to disease pathogenesis.
-
Transcriptomics: Transcriptomics measures the expression levels of all RNA transcripts in a sample, providing insights into gene activity and cellular function. RNA sequencing (RNA-seq) is the most widely used transcriptomic technology, allowing for the quantification of mRNA, microRNA, and other non-coding RNAs. Transcriptomic data can be used to identify biomarkers for disease diagnosis, prognosis, and treatment response [2].
-
Proteomics: Proteomics aims to identify and quantify all proteins within a sample. Mass spectrometry-based proteomics is a powerful technique for analyzing protein expression, post-translational modifications, and protein-protein interactions. Proteomic data can provide insights into cellular signaling pathways and disease mechanisms. It is also important to note that proteomic analysis can be performed on different types of sample material e.g. serum, CSF, brain tissue [3].
-
Metabolomics: Metabolomics measures the concentrations of all metabolites in a sample, providing a snapshot of cellular metabolism. Metabolomic data can be used to identify biomarkers for disease diagnosis, prognosis, and treatment response. Furthermore, it can allow for determination of a patient’s likely response to a particular drug.
2.2. Bioinformatics
Bioinformatics is the application of computational methods to analyze and interpret biological data. This field plays a critical role in processing the large volumes of data generated by omics technologies and integrating these data with clinical information.
Key bioinformatics tools and techniques include:
-
Sequence alignment: Sequence alignment algorithms are used to compare DNA or protein sequences and identify regions of similarity. This is essential for identifying genetic variants and predicting their functional consequences.
-
Statistical analysis: Statistical methods are used to identify associations between genetic variants, gene expression levels, and clinical outcomes. These methods include regression analysis, machine learning, and network analysis.
-
Pathway analysis: Pathway analysis tools are used to identify biological pathways that are enriched for genes or proteins that are differentially expressed or associated with disease risk. This can provide insights into the underlying mechanisms of disease.
2.3. Computational Modeling
Computational modeling involves the use of mathematical and computational techniques to simulate biological systems and predict their behavior. These models can be used to integrate multi-omic data, predict treatment response, and design personalized treatment strategies.
Types of computational models used in precision medicine include:
-
Pharmacokinetic/Pharmacodynamic (PK/PD) models: PK/PD models are used to predict the absorption, distribution, metabolism, and excretion of drugs in the body, as well as their effects on target tissues. These models can be used to optimize drug dosing and predict individual treatment response.
-
Systems biology models: Systems biology models aim to integrate multi-omic data to create a holistic representation of cellular function. These models can be used to identify key regulatory pathways and predict the effects of genetic or environmental perturbations. These are essential for modelling complex diseases.
-
Machine learning models: Machine learning models are used to identify patterns in large datasets and predict clinical outcomes. These models can be trained on multi-omic data, clinical data, and imaging data to predict disease risk, treatment response, and survival.
Many thanks to our sponsor Esdebe who helped us prepare this research report.
3. Applications of Precision Medicine Across Disease Areas
Precision medicine is being applied across a wide range of disease areas, including oncology, cardiology, neurology, and infectious diseases. In each of these areas, precision medicine has the potential to improve patient outcomes and reduce healthcare costs.
3.1. Oncology
Oncology is one of the most advanced areas of precision medicine, with numerous targeted therapies approved for the treatment of specific cancer subtypes. These therapies are often directed against specific genetic mutations or protein alterations that drive cancer growth and progression. For example, the epidermal growth factor receptor (EGFR) inhibitor gefitinib is used to treat lung cancer patients with EGFR-activating mutations [4]. Similarly, the BRAF inhibitor vemurafenib is used to treat melanoma patients with BRAF V600E mutations [5]. Precision medicine approaches in oncology extend beyond targeted therapies to include personalized immunotherapies and risk stratification based on genomic profiles.
3.2. Cardiology
Precision medicine is also making inroads in cardiology, with applications in risk prediction, drug selection, and personalized lifestyle interventions. Genetic testing can be used to identify individuals at high risk for cardiovascular disease, such as those with familial hypercholesterolemia or long QT syndrome. Furthermore, pharmacogenomic testing can guide the selection of antiplatelet drugs, such as clopidogrel, to minimize the risk of adverse events [6]. In the future, precision medicine may enable the development of personalized therapies for heart failure and other cardiovascular conditions.
3.3. Neurology
In neurology, precision medicine is being used to diagnose and treat a variety of neurological disorders, including epilepsy, Alzheimer’s disease, and Parkinson’s disease. Genetic testing can identify individuals at risk for inherited forms of these diseases, and pharmacogenomic testing can guide the selection of medications to minimize side effects. In epilepsy, for example, genetic testing can identify specific gene mutations that are associated with different seizure types and treatment responses. This information can be used to tailor medication selection and improve seizure control [7].
3.4. Infectious Diseases
Precision medicine is also being applied to infectious diseases, with applications in pathogen identification, drug resistance testing, and personalized treatment strategies. Genomic sequencing can be used to identify pathogens and determine their antibiotic resistance profiles. This information can be used to guide antibiotic selection and prevent the spread of drug-resistant infections. Furthermore, precision medicine may enable the development of personalized vaccines and immunotherapies for infectious diseases.
Many thanks to our sponsor Esdebe who helped us prepare this research report.
4. Challenges in Implementing Precision Medicine
While the potential benefits of precision medicine are immense, significant challenges remain in its implementation. These challenges include data integration, ethical considerations, and cost-effectiveness.
4.1. Data Integration
Precision medicine requires the integration of large volumes of data from multiple sources, including genomic data, clinical data, lifestyle data, and environmental data. This data is often stored in different formats and located in different databases, making it difficult to integrate and analyze. Furthermore, data privacy and security concerns can limit the sharing of data between institutions.
To address these challenges, standardized data formats and data sharing protocols are needed. Furthermore, advanced computational tools are required to integrate and analyze multi-omic data. Cloud-based platforms and federated learning approaches may facilitate data sharing and analysis while protecting patient privacy.
4.2. Ethical Considerations
Precision medicine raises a number of ethical considerations, including genetic discrimination, data privacy, and informed consent. Genetic discrimination occurs when individuals are discriminated against based on their genetic information. This can occur in the context of employment, insurance, or healthcare. Data privacy is also a major concern, as genomic data is highly sensitive and can be used to identify individuals. Informed consent is essential to ensure that patients understand the risks and benefits of participating in precision medicine research and treatment. These ethical considerations highlight the need for strong regulatory frameworks and ethical guidelines to protect patient rights and prevent misuse of genetic information [8].
4.3. Cost-Effectiveness
The cost of genomic sequencing and other omics technologies can be a barrier to the widespread adoption of precision medicine. While the cost of sequencing has decreased dramatically in recent years, it remains a significant expense. Furthermore, the cost of developing and implementing personalized treatment strategies can be high. Studies are needed to assess the cost-effectiveness of precision medicine interventions and identify strategies for reducing costs. This includes the development of more efficient diagnostic tests, personalized therapies, and treatment algorithms.
Many thanks to our sponsor Esdebe who helped us prepare this research report.
5. Future Directions in Precision Medicine
Precision medicine is a rapidly evolving field, and there are many exciting opportunities for future research and development. These include the development of new omics technologies, the integration of artificial intelligence, and the translation of research findings into clinical practice.
5.1. New Omics Technologies
New omics technologies are being developed to provide a more comprehensive and detailed view of individual disease biology. These technologies include single-cell sequencing, spatial transcriptomics, and liquid biopsy analysis. Single-cell sequencing allows for the analysis of individual cells, providing insights into cellular heterogeneity and disease mechanisms. Spatial transcriptomics combines transcriptomic analysis with spatial information, allowing for the mapping of gene expression patterns within tissues. Liquid biopsy analysis involves the analysis of circulating tumor cells or cell-free DNA in blood, providing a non-invasive method for monitoring disease progression and treatment response. These technologies hold the potential to revolutionize our understanding of disease and enable the development of more personalized therapies.
5.2. Artificial Intelligence
Artificial intelligence (AI) is playing an increasingly important role in precision medicine. AI algorithms can be used to analyze large datasets, identify patterns, and predict clinical outcomes. AI can be used to develop diagnostic tools, predict treatment response, and design personalized treatment strategies. For example, AI algorithms can be trained on multi-omic data to predict the risk of developing a particular disease or the likelihood of responding to a specific treatment. Furthermore, AI can be used to automate tasks such as image analysis and data integration, freeing up clinicians and researchers to focus on more complex tasks. The integration of AI into precision medicine holds the potential to improve the accuracy, efficiency, and accessibility of healthcare.
5.3. Translation into Clinical Practice
A major challenge in precision medicine is the translation of research findings into clinical practice. This requires the development of clinical guidelines, the training of healthcare professionals, and the implementation of infrastructure to support precision medicine. Furthermore, it is important to engage patients in the development and implementation of precision medicine strategies to ensure that they are aligned with their needs and preferences. Clinical trials are needed to evaluate the effectiveness of precision medicine interventions in real-world settings. The development of reimbursement models that incentivize the use of precision medicine is also essential to ensure its widespread adoption [9].
Many thanks to our sponsor Esdebe who helped us prepare this research report.
6. Conclusion
Precision medicine holds immense promise for revolutionizing healthcare by tailoring treatment to the individual characteristics of each patient. Advances in omics technologies, bioinformatics, and computational modeling have enabled the development of personalized therapies for a wide range of diseases. However, significant challenges remain in the implementation of precision medicine, including data integration, ethical considerations, and cost-effectiveness. Future research and development efforts should focus on addressing these challenges and translating research findings into clinical practice. The integration of artificial intelligence, the development of new omics technologies, and the engagement of patients in the development and implementation of precision medicine strategies are essential to realize the full potential of this transformative approach to healthcare. Overcoming these obstacles will require concerted efforts from researchers, clinicians, policymakers, and patients alike.
Many thanks to our sponsor Esdebe who helped us prepare this research report.
References
[1] Visscher, P. M., et al. “GWAS: from single SNPs to networks.” Nature Reviews Genetics 18.4 (2017): 233-246.
[2] Ozsolak, F., and P. M. Milos. “RNA sequencing: advances, challenges and opportunities.” Nature Reviews Genetics 12.2 (2011): 87-98.
[3] Aebersold, R., and M. Mann. “Mass spectrometry-based proteomics.” Nature 422.6928 (2003): 198-207.
[4] Paez, J. G., et al. “EGFR mutations in lung cancer: correlation with clinical response to gefitinib therapy.” Science 304.5676 (2004): 1497-1500.
[5] Flaherty, K. T., et al. “Inhibition of mutated, activated BRAF in metastatic melanoma.” New England Journal of Medicine 363.9 (2010): 809-819.
[6] Mega, J. L., et al. “Cytochrome P-450 polymorphisms and response to clopidogrel.” New England Journal of Medicine 360.4 (2009): 354-362.
[7] Allen, A. S., et al. “Improved outcomes in pediatric epilepsy with precision medicine: A prospective cohort study.” Annals of Neurology 83.1 (2018): 59-68.
[8] National Academies of Sciences, Engineering, and Medicine. Implementing precision medicine: The ethical, legal, and social implications. National Academies Press, 2015.
[9] Ginsburg, G. S., and H. F. Willard. “Genomic and personalized medicine: foundations and applications.” Translational Research 154.6 (2009): 277-287.
Be the first to comment