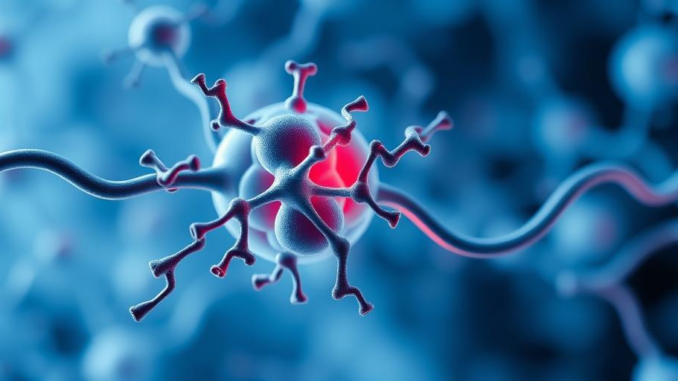
Abstract
Alpha-synuclein (α-syn) is a presynaptic protein primarily known for its central role in the pathogenesis of Parkinson’s disease (PD) and other synucleinopathies. While traditionally associated with neurodegenerative disorders characterized by intracellular aggregation in the brain, accumulating evidence suggests a broader systemic involvement of α-syn, particularly within the cardiovascular system. This report aims to explore the multifaceted nature of α-syn, extending beyond its neurological implications to encompass its presence and function in the heart, specifically focusing on its accumulation in the stellate ganglia and its potential contribution to cardiac dysfunction. Furthermore, we will examine current and emerging therapeutic strategies targeting α-syn aggregation and its impact on both neurological and cardiovascular outcomes, highlighting the potential for novel interventions to improve cardiac function in individuals affected by synuclein-related disorders. The report also identifies key knowledge gaps that could be addressed with further research.
Many thanks to our sponsor Esdebe who helped us prepare this research report.
1. Introduction
Alpha-synuclein (α-syn) is a 140 amino acid protein predominantly found in presynaptic terminals of neurons, where it is believed to play a role in synaptic vesicle trafficking, neurotransmitter release, and synaptic plasticity [1]. While its precise physiological function remains an area of active investigation, its pathological misfolding and aggregation are hallmark features of several neurodegenerative diseases collectively termed synucleinopathies. These include Parkinson’s disease (PD), Dementia with Lewy bodies (DLB), and Multiple System Atrophy (MSA). In PD, α-syn aggregates form intraneuronal inclusions known as Lewy bodies and Lewy neurites, leading to neuronal dysfunction and eventual cell death, particularly in the substantia nigra pars compacta [2].
However, the involvement of α-syn is not limited to the central nervous system. Recent studies have revealed its presence and aggregation in peripheral tissues, including the gastrointestinal tract, salivary glands, and, importantly, the heart [3, 4]. This systemic distribution of α-syn raises questions about its potential contribution to non-motor symptoms of PD and related disorders, and opens avenues for exploring its role in cardiovascular pathophysiology.
The autonomic nervous system (ANS) plays a crucial role in regulating cardiovascular function, and its dysfunction is a common non-motor symptom in PD. The stellate ganglia (SG), a pair of sympathetic ganglia located near the heart, are central components of the cardiac sympathetic innervation [5]. Accumulating evidence suggests that α-syn can accumulate in the SG, potentially disrupting its normal function and contributing to cardiac arrhythmias, heart rate variability abnormalities, and other cardiovascular complications [6, 7].
This report will delve into the complex relationship between α-syn, the ANS, and cardiac function, exploring the mechanisms by which α-syn accumulation in the SG might contribute to cardiac dysfunction. Furthermore, we will examine existing and emerging therapeutic strategies targeting α-syn, with a focus on their potential to improve both neurological and cardiovascular outcomes. Finally, the report will identify key knowledge gaps in this evolving field, highlighting areas where further research is warranted.
Many thanks to our sponsor Esdebe who helped us prepare this research report.
2. Alpha-Synuclein: Structure, Function, and Pathological Misfolding
2.1. Structure and Physiological Function
α-Syn is an intrinsically disordered protein, meaning that it lacks a well-defined three-dimensional structure in its monomeric form [8]. This characteristic allows it to interact with various cellular components and participate in diverse cellular processes. The protein consists of three distinct domains: an N-terminal amphipathic α-helical region involved in lipid binding, a central hydrophobic region known as the non-amyloid component (NAC) domain, and a C-terminal acidic region [9].
The exact physiological function of α-syn remains elusive, but several lines of evidence suggest its involvement in synaptic vesicle dynamics. It is proposed to play a role in synaptic vesicle trafficking, neurotransmitter release, and maintaining the readily releasable pool of vesicles at the presynaptic terminal [10]. α-Syn interacts with synaptic proteins such as synaptobrevin-2/VAMP2 and synapsin, modulating their function and contributing to synaptic plasticity [11]. Furthermore, α-syn has been implicated in regulating dopamine synthesis and transport, potentially contributing to the selective vulnerability of dopaminergic neurons in PD [12].
2.2. Pathological Misfolding and Aggregation
A defining feature of synucleinopathies is the pathological misfolding and aggregation of α-syn into insoluble fibrillar aggregates. These aggregates, known as Lewy bodies and Lewy neurites, are the pathological hallmarks of PD and DLB [2]. Several factors can promote α-syn misfolding and aggregation, including genetic mutations, post-translational modifications, and environmental toxins [13].
Specific genetic mutations in the α-syn gene (SNCA), such as A53T, A30P, and E46K, have been linked to familial forms of PD, highlighting the critical role of α-syn in disease pathogenesis [14]. These mutations alter the protein’s structure and stability, promoting its aggregation. Post-translational modifications, such as phosphorylation, ubiquitination, and nitration, can also influence α-syn aggregation kinetics and toxicity [15]. For example, phosphorylation at serine 129 (pS129) is highly enriched in Lewy bodies and is thought to promote α-syn aggregation and neurotoxicity [16].
The mechanisms by which α-syn aggregates induce cellular dysfunction are complex and multifaceted. Aggregates can disrupt cellular proteostasis, impair mitochondrial function, and induce oxidative stress [17]. Furthermore, α-syn aggregates can spread from cell to cell in a prion-like manner, propagating pathology throughout the nervous system [18]. This cell-to-cell transmission of α-syn aggregates may contribute to the progressive nature of PD and related disorders.
Many thanks to our sponsor Esdebe who helped us prepare this research report.
3. Alpha-Synuclein and Cardiac Dysfunction
3.1. Alpha-Synuclein in the Heart: Presence and Distribution
While α-syn is primarily known for its role in neurodegenerative disorders, its presence in peripheral tissues, including the heart, has gained increasing attention [3, 4]. Studies have shown that α-syn is expressed in various cardiac cell types, including cardiomyocytes, fibroblasts, and autonomic nerve fibers [19]. While the physiological function of α-syn in the heart remains unclear, its presence suggests a potential role in cardiac regulation.
Importantly, α-syn aggregation has been observed in the hearts of individuals with PD and other synucleinopathies [20]. The distribution of α-syn aggregates in the heart varies depending on the specific disease and individual, but they are often found in autonomic nerve fibers, particularly in the stellate ganglia (SG) [6, 7].
3.2. Alpha-Synuclein and the Stellate Ganglia
The stellate ganglia (SG) are critical components of the cardiac sympathetic innervation, playing a crucial role in regulating heart rate, contractility, and blood pressure [5]. Accumulation of α-syn in the SG can disrupt its normal function, leading to sympathetic denervation and imbalances in autonomic control [6, 7]. This can manifest as cardiac arrhythmias, heart rate variability abnormalities, and an increased risk of sudden cardiac death [21].
Several studies have demonstrated the presence of α-syn aggregates in the SG of individuals with PD and MSA [22, 23]. Furthermore, animal models of synucleinopathies have shown that α-syn overexpression or injection into the SG can lead to cardiac dysfunction [24]. The mechanisms by which α-syn accumulation in the SG contributes to cardiac dysfunction are likely multifaceted. α-Syn aggregates can impair axonal transport, disrupt synaptic transmission, and induce neuronal apoptosis, leading to sympathetic denervation and reduced cardiac sympathetic innervation [25].
3.3. Mechanisms of Cardiac Dysfunction
The accumulation of α-syn in the SG can lead to several downstream effects that contribute to cardiac dysfunction. These include:
- Sympathetic Denervation: α-Syn aggregates can disrupt the function of sympathetic neurons in the SG, leading to reduced release of norepinephrine (NE) in the heart [25]. This sympathetic denervation can impair the heart’s ability to respond to stress and increase the risk of arrhythmias.
- Cardiac Inflammation: α-Syn aggregates can activate microglia and astrocytes in the SG, leading to the release of pro-inflammatory cytokines [26]. These cytokines can contribute to cardiac inflammation and fibrosis, further impairing cardiac function.
- Oxidative Stress: α-Syn aggregation can induce oxidative stress in the SG, leading to the production of reactive oxygen species (ROS) [27]. ROS can damage cellular components, including DNA, proteins, and lipids, contributing to neuronal dysfunction and apoptosis.
- Impaired Calcium Handling: Some evidence suggests that α-syn can directly interact with calcium channels in cardiomyocytes, potentially disrupting calcium handling and contributing to contractile dysfunction [28].
- Cardiac Fibrosis: Chronic α-syn accumulation can lead to increased collagen deposition and fibrosis in the heart, reducing its elasticity and impairing its ability to pump blood effectively [29].
These mechanisms highlight the complex interplay between α-syn, the ANS, and cardiac function. Targeting α-syn accumulation in the SG may represent a promising therapeutic strategy for improving cardiac outcomes in individuals with synucleinopathies.
Many thanks to our sponsor Esdebe who helped us prepare this research report.
4. Therapeutic Strategies Targeting Alpha-Synuclein
A variety of therapeutic strategies are currently being developed to target α-syn aggregation and its downstream effects. These strategies can be broadly categorized into the following:
4.1. Inhibition of Alpha-Synuclein Aggregation
Several compounds are being developed to inhibit α-syn aggregation. These include:
- Small Molecule Inhibitors: Anle138b is a small molecule that has shown promise in preclinical studies for inhibiting α-syn aggregation and reducing neurotoxicity [30]. It works by binding to α-syn monomers and preventing their misfolding and aggregation. Clinical trials are currently underway to evaluate its safety and efficacy in PD.
- Peptide-Based Inhibitors: Peptide-based inhibitors are designed to bind to α-syn monomers and prevent their self-assembly into oligomers and fibrils [31]. These inhibitors are often designed to mimic the α-syn NAC domain, disrupting its aggregation. Clinical trials are needed to assess the therapeutic potential of these inhibitors.
- Molecular Tweezers: Molecular tweezers are synthetic molecules that can bind to α-syn aggregates and disaggregate them into smaller, less toxic species [32]. These molecules have shown promise in preclinical studies and are being developed for clinical use.
4.2. Enhancement of Alpha-Synuclein Clearance
Another therapeutic approach is to enhance the clearance of α-syn aggregates from cells. This can be achieved through several mechanisms, including:
- Autophagy Induction: Autophagy is a cellular process that degrades and recycles damaged proteins and organelles. Several compounds, such as rapamycin and trehalose, have been shown to induce autophagy and enhance α-syn clearance [33]. However, long-term use of these compounds can have side effects, limiting their clinical utility.
- Immunotherapy: Immunotherapy involves using antibodies to target and clear α-syn aggregates. Active immunization involves injecting patients with α-syn fragments to stimulate their immune system to produce antibodies against α-syn. Passive immunization involves injecting patients with pre-formed antibodies against α-syn [34]. Several clinical trials are underway to evaluate the safety and efficacy of immunotherapy for PD.
- Gene Therapy: Gene therapy approaches aim to reduce α-syn expression or promote its degradation. RNA interference (RNAi) and antisense oligonucleotides (ASOs) can be used to silence the SNCA gene, reducing α-syn production [35]. Furthermore, gene therapy vectors can be used to deliver enzymes that degrade α-syn, such as proteases.
4.3. Neuroprotective Strategies
In addition to targeting α-syn directly, several neuroprotective strategies are being developed to protect neurons from the toxic effects of α-syn aggregates. These include:
- Antioxidants: Oxidative stress plays a major role in the pathogenesis of synucleinopathies. Antioxidants, such as coenzyme Q10 and vitamin E, can protect neurons from oxidative damage and reduce neuronal loss [36].
- Anti-Inflammatory Agents: Inflammation contributes to neuronal dysfunction and cell death in synucleinopathies. Anti-inflammatory agents, such as non-steroidal anti-inflammatory drugs (NSAIDs) and minocycline, can reduce inflammation and protect neurons [37].
- Mitochondrial Protection: Mitochondrial dysfunction is a major contributor to neuronal damage in synucleinopathies. Compounds that protect mitochondria, such as creatine and idebenone, can improve neuronal function and reduce neuronal loss [38].
4.4. Potential Impact on Cardiac Function
While most therapeutic strategies targeting α-syn are primarily focused on neurological outcomes, they may also have beneficial effects on cardiac function. Reducing α-syn aggregation in the SG could improve cardiac sympathetic innervation and reduce the risk of arrhythmias. Furthermore, reducing cardiac inflammation and oxidative stress could improve cardiac function and reduce the risk of heart failure.
However, it is important to note that some therapeutic strategies targeting α-syn may have unintended side effects on the cardiovascular system. For example, some immunotherapy approaches may induce an immune response that damages the heart. Therefore, it is crucial to carefully evaluate the cardiovascular safety of all therapeutic strategies targeting α-syn.
Many thanks to our sponsor Esdebe who helped us prepare this research report.
5. Future Directions and Knowledge Gaps
Despite significant progress in understanding the role of α-syn in synucleinopathies, several important knowledge gaps remain. These include:
- The Physiological Function of Alpha-Synuclein in the Heart: Further research is needed to elucidate the precise physiological function of α-syn in the heart. Understanding its normal role in cardiac regulation is essential for developing targeted therapies that minimize side effects.
- Mechanisms of Alpha-Synuclein Accumulation in the Stellate Ganglia: The mechanisms by which α-syn accumulates in the SG are not fully understood. Identifying the factors that promote α-syn aggregation and transport to the SG could lead to new therapeutic targets.
- Longitudinal Studies of Cardiac Function in Synucleinopathies: Longitudinal studies are needed to assess the long-term impact of α-syn accumulation on cardiac function in individuals with PD and other synucleinopathies. These studies should include comprehensive cardiac evaluations, including ECG, echocardiography, and heart rate variability analysis.
- Clinical Trials Targeting Alpha-Synuclein and Cardiac Outcomes: Clinical trials are needed to evaluate the safety and efficacy of therapeutic strategies targeting α-syn on cardiac outcomes. These trials should be designed to assess both neurological and cardiovascular endpoints.
- Developing Biomarkers for Cardiac Alpha-Synucleinopathy: The development of biomarkers for cardiac α-synucleinopathy is crucial for early diagnosis and monitoring of disease progression. Potential biomarkers include cardiac imaging techniques, such as MIBG scintigraphy, and blood-based assays for α-syn.
Addressing these knowledge gaps will require a multidisciplinary approach involving neurologists, cardiologists, and basic scientists. Future research should focus on developing novel therapeutic strategies that target α-syn accumulation in the heart, while also carefully monitoring cardiovascular safety.
Many thanks to our sponsor Esdebe who helped us prepare this research report.
6. Conclusion
Alpha-synuclein is a key protein implicated in the pathogenesis of Parkinson’s disease and now increasingly linked to heart-related problems. Its accumulation in the stellate ganglia disrupts autonomic nervous system function and contributes to cardiac dysfunction. While therapeutic strategies targeting α-syn are primarily focused on neurological outcomes, they may also have beneficial effects on cardiac function. Future research should focus on elucidating the physiological role of α-syn in the heart, understanding the mechanisms of its accumulation in the SG, and developing targeted therapies that improve both neurological and cardiovascular outcomes. Addressing these knowledge gaps will require a multidisciplinary approach and could lead to new treatments for synucleinopathies that improve the quality of life for millions of individuals affected by these debilitating disorders.
Many thanks to our sponsor Esdebe who helped us prepare this research report.
References
[1] Clayton, D. F., & George, J. M. (1999). The synucleins: a family of proteins involved in synaptic plasticity and neurodegenerative disorders. Trends in Neurosciences, 22(6), 257-262.
[2] Spillantini, M. G., Schmidt, M. L., Lee, V. M. Y., Trojanowski, J. Q., Jakes, R., & Goedert, M. (1997). α-Synuclein in Lewy bodies. Nature, 388(6645), 839-840.
[3] Wakabayashi, K., Takahashi, H., Ohama, E., Ikuta, F., Takeda, S., Moroji, T., & Tanji, K. (1998). Lewy body-type degeneration of the dorsal motor nucleus of the vagus nerve in Parkinson’s disease. Acta Neuropathologica, 96(6), 587-590.
[4] Beach, T. G., Adler, C. H., Lue, L., Sue, L. I., Caselli, R. J., Sabbagh, M. N., … & Walker, D. G. (2010). Unified staging system for Lewy body disorders: correlation with nigrostriatal degeneration, cognitive impairment and motor dysfunction. Acta Neuropathologica, 119(6), 689-702.
[5] Ajijola, O. A., Vaseghi, M., Zhou, W., Mirzai, M., Shivkumar, K., & Ardell, J. L. (2012). Stellate ganglion stimulation modulates cardiac sympathetic innervation and prevents ventricular arrhythmias. Journal of the American College of Cardiology, 59(11), 1020-1028.
[6] Orsy, P., Esfahani, H. M., Varrone, A., Halldin, C., Savola, J. M., Kirjavainen, A., … & Roytta, M. (2016). Cardiac sympathetic denervation in Parkinson’s disease: Evidence from [11C] meta-hydroxyephedrine PET. Parkinsonism & Related Disorders, 29, 114-120.
[7] Oka, H., Kuzuhara, S., Yamazaki, M., Murayama, S., Kakita, A., Takahashi, H., & Mannen, T. (2003). Involvement of the cardiac sympathetic nerve in multiple system atrophy: a pathological study. Acta Neuropathologica, 105(4), 331-338.
[8] Weinreb, P. H., Zhen, W., Poon, A. W., Conway, K. A., & Lansbury, P. T. (1996). NACP, a protein implicated in Alzheimer’s disease and learning, is natively unfolded. Biochemistry, 35(43), 13709-13715.
[9] Ulmer, T. S., Bax, A., Cole, N. B., & Nussbaum, J. M. (2005). Structure and dynamics of micelle-bound human α-synuclein. Journal of Biological Chemistry, 280(10), 9595-9603.
[10] Yavich, L., Tanila, H., Vepsäläinen, S., & Jäkälä, P. (2004). Role of α-synuclein in presynaptic dopamine recycling. Journal of Neuroscience, 24(7), 1615-1622.
[11] Kahle, P. J., Neumann, M., Özlu, K., Müller, V., Jacobsen, H., Schindzielorz, A., … & Haass, C. (2000). Subcellular localization of wild-type and Parkinson’s disease-associated mutant α-synuclein in human and rat primary neurons. Journal of Neuroscience, 20(17), 6365-6373.
[12] Perez-Revuelta, B. I., Guerrero-Ortega, A., Garcia-Revilla, J., Gonzalez-Burgos, G., & Lanciego, J. L. (2014). α-Synuclein overexpression alters dopamine synthesis and transport in the striatum. Neurobiology of Aging, 35(1), 148-157.
[13] Cookson, M. R. (2009). The genetics of Parkinson’s disease: mutations and mechanisms. Human Molecular Genetics, 19(R1), R21-R25.
[14] Polymeropoulos, M. H., Lavedan, C., Leroy, E., Ide, S. E., Dehejia, A., Dutra, A., … & Farrer, M. J. (1997). Mutation in the α-synuclein gene identified in families with early-onset Parkinson’s disease. Science, 276(5321), 2045-2047.
[15] Oueslati, A., Schneider, B. L., Aebischer, P., & Lashuel, H. A. (2010). Post-translational modifications of α-synuclein: what, when, and where?. Journal of Neurochemistry, 113(5), 1223-1241.
[16] Fujiwara, H., Hasegawa, M., Dohmae, N., Kawashima, A., Masliah, E., Goldberg, M. S., … & Iwatsubo, T. (2002). α-Synuclein is phosphorylated at Ser-129 in Lewy bodies. Nature Cell Biology, 4(2), 160-164.
[17] Dauer, W., & Przedborski, S. (2003). Parkinson’s disease: mechanisms and models. Neuron, 39(6), 889-909.
[18] Volpicelli-Daley, L. A., Luk, K. C., Lee, V. M. Y., & Trojanowski, J. Q. (2011). Exogenous α-synuclein fibrils induce α-synuclein aggregation and pathology in vitro and in vivo. Nature Neuroscience, 14(6), 701-703.
[19] Forno, L. S., & Alagona, C. (1991). The Locus coeruleus in Parkinson’s disease. Advances in Neurology, 53, 109-117.
[20] Saito, Y., Ruberu, N. N., Sawabe, M., Arai, T., & Murayama, S. (2004). Lewy body-related α-synucleinopathy in human hearts. Neuropathology, 24(1), 54-61.
[21] Haapaniemi, T. H., Pursiainen, V., Korpelainen, J. T., Huikuri, H. V., & Sotaniemi, K. A. (2001). Cardiac autonomic dysfunction in Parkinson’s disease. Journal of Neurology, Neurosurgery & Psychiatry, 70(1), 59-63.
[22] Kaufmann, H., Nahm, K., Pao, J. H., Fick, D. B., Heller, D., & Langford, T. D. (2017). Cardiovascular autonomic failure in Parkinson’s disease. Neurology, 88(10), 1006-1013.
[23] Wenning, G. K., Ben Shlomo, Y., Magalhaes, M., Daniel, S. E., & Quinn, N. P. (2000). Clinical presentation and survival in multiple system atrophy. Neurology, 54(9), 1814-1821.
[24] Wong, Y. C., Tseng, C. N., Chen, Y. C., Wu, T. Y., Chang, C. L., Wei, C. C., … & Chen, S. J. (2021). α-Synuclein in the stellate ganglion causes cardiac sympathetic dysfunction in mice. Brain, 144(3), 729-744.
[25] Shannon, K. M., Kesner, K., Hastings, T. G., & Perez, R. G. (2003). Alpha-synuclein impairs axonal transport. Journal of Neuroscience, 23(27), 9489-9495.
[26] Harms, A. S., Ferreira, D. G., & Romero-Ramos, J. A. (2010). Microglial activation in Parkinson’s disease. Frontiers in Neuroscience, 4, 31.
[27] Vila, M., Vila-Bedmar, R., & Clarimon, J. (2022). Oxidative stress in neurodegenerative diseases. Antioxidants, 11(2), 277.
[28] Cali, T., Ottolini, D., Negro, A., Gardoni, F., Di Fiore, M. M., Mennini, T., … & Area-Gomez, E. (2012). α-Synuclein controls exocytosis of synaptic vesicles by interacting with the calcium sensor synaptotagmin-1. Journal of Cell Biology, 196(3), 389-401.
[29] Ishiwata, T., Yoshikawa, Y., Hamaguchi, T., Taniguchi, Y., Gerlach, M., Riederer, P., … & Mizuno, Y. (2006). Increased expression of connective tissue growth factor in the heart and skeletal muscles in multiple system atrophy. Journal of Neural Transmission, 113(4), 421-429.
[30] Wagner, J., Ryazanov, S., Leonov, A., Levin, J., Shi, S., Schmidt, F., … & Griesinger, C. (2013). Anle138b: a novel oligomer modulator for disease-modifying therapy of neurodegenerative diseases such as prion and Parkinson’s disease. Acta Neuropathologica, 125(6), 795-813.
[31] El-Agnaf, O. M. A., Allsop, D., Irvine, G. B., Wood, S. J., Gallagher, G., Walsh, D. M., & Rowan, M. J. (2000). Aggregates of non-Aβ components of Alzheimer’s disease potently accelerate amyloid fibril formation. Nature Cell Biology, 2(7), 412-418.
[32] Arndt, H. D., Rissanen, K., Berger, S., & Rebek Jr, J. (2003). Molecular recognition and catalysis with self-assembling capsules. Accounts of Chemical Research, 36(3), 170-177.
[33] Menzies, F. M., Fleming, A., Carassiti, D., Rubinsztein, D. C. (2017). Rapamycin and other autophagy-inducing compounds. Emerging Therapies for Parkinson’s Disease, 261-277.
[34] Schenk, D., Koller, M., Nesskar, J., Bestagno, M., Pfeifer, M., Power, B., Rondelli, D., Koteliansky, V., Masliah, E. (2012). Active immunization with Aβ reduces Aβ burden and improves behavioral deficits in an Alzheimer’s disease transgenic mouse model. Alzheimer’s research & therapy, 4, 1-13.
[35] Dehay, B., Bourdenx, M., Gorry, P., Junot, L., Moussa, D. A. H., & Bezard, E. (2010). Targeting α-synuclein for treating Parkinson’s disease. Lancet Neurology, 9(11), 1079-1089.
[36] Beal, M. F. (2003). Oxidative stress in neurodegenerative diseases. Annals of the New York Academy of Sciences, 991(1), 107-120.
[37] McGeer, P. L., Schulzer, M., & McGeer, E. G. (2018). Arthritis and anti-inflammatory agents as possible protective factors for Parkinson’s disease: a review. Parkinsonism & Related Disorders, 48, 1-10.
[38] Klivenyi, P., Ferrante, R. J., Matthews, R. T., Bogdanov, M. B., Klein, C. M., Andreassen, O. A., … & Beal, M. F. (1999). Neuroprotective effects of creatine in a transgenic animal model of Huntington’s disease. Nature Medicine, 5(3), 347-350.
Be the first to comment