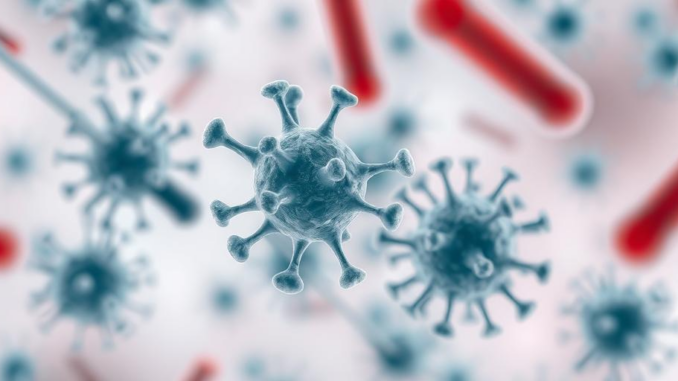
Abstract
Bacteria, ubiquitous and diverse, are fundamental to life on Earth. Their roles span biogeochemical cycling, symbiotic relationships, and pathogenesis. This report provides a comprehensive overview of bacterial biology, encompassing their structural intricacies, metabolic versatility, and remarkable genetic plasticity. We delve into the mechanisms driving antibiotic resistance, including mutational evolution, horizontal gene transfer, and the influence of the microbiome. We explore the clinical manifestations of diverse bacterial infections and highlight emerging bacterial threats, specifically focusing on the convergence of bacterial evolution and artificial intelligence (AI) in predicting and combating antibiotic resistance. Furthermore, we critically assess current strategies for preventing the spread of resistant bacteria and propose future directions for research and intervention, emphasizing the urgent need for a holistic, interdisciplinary approach to mitigate the growing threat of antimicrobial resistance.
Many thanks to our sponsor Esdebe who helped us prepare this research report.
1. Introduction
Bacteria represent one of the three domains of life, exhibiting a remarkable diversity in morphology, metabolism, and ecological niche. Their ancient origins and rapid evolutionary rate have allowed them to adapt to virtually every environment on Earth, from the deepest ocean trenches to the human gut. Beyond their ecological importance, bacteria play critical roles in human health, both as beneficial symbionts and as causative agents of disease. The discovery of antibiotics in the 20th century revolutionized medicine, providing effective treatments for bacterial infections. However, the widespread use and misuse of antibiotics have driven the evolution of antibiotic-resistant bacteria, posing a significant threat to global public health. This report aims to provide a comprehensive overview of bacterial biology, focusing on the mechanisms driving antibiotic resistance and exploring the interplay between bacterial evolution and AI-driven predictive modeling in combating this growing crisis. This report will not only cover the basics of bacterial biology, mechanisms of resistance development, types of bacterial infections and the role of the microbiome in resistance, but also look at emerging bacterial threats, strategies for preventing the spread of resistant bacteria and the impact of AI in helping to prevent the spread of resistant bacteria.
Many thanks to our sponsor Esdebe who helped us prepare this research report.
2. Bacterial Cell Structure and Function
2.1. Basic Cellular Architecture
Bacterial cells are generally characterized by their prokaryotic structure, lacking a membrane-bound nucleus and other complex organelles found in eukaryotes. The bacterial cell is typically enclosed by a plasma membrane, composed of a phospholipid bilayer, which regulates the transport of nutrients and waste products. Most bacteria possess a cell wall external to the plasma membrane, providing structural support and protection against osmotic stress. The composition of the cell wall varies between Gram-positive and Gram-negative bacteria, a key distinction used in bacterial identification. Gram-positive bacteria have a thick layer of peptidoglycan, a polymer of sugars and amino acids, while Gram-negative bacteria have a thinner peptidoglycan layer surrounded by an outer membrane containing lipopolysaccharide (LPS), a potent endotoxin.
2.2. Genetic Material and Protein Synthesis
The bacterial genome typically consists of a single, circular chromosome located in the nucleoid region. Unlike eukaryotes, bacteria lack histones, and their DNA is supercoiled to fit within the cell. In addition to the chromosome, bacteria may contain plasmids, small circular DNA molecules that carry non-essential genes, such as those conferring antibiotic resistance. Bacterial protein synthesis occurs in ribosomes, which are structurally distinct from eukaryotic ribosomes. Transcription and translation are coupled in bacteria, meaning that translation begins before transcription is complete, a feature that allows for rapid protein production in response to environmental changes.
2.3. Motility and Adhesion
Many bacteria are motile, using flagella to propel themselves through liquid environments. Flagella are helical filaments composed of the protein flagellin, which are rotated by a motor complex embedded in the cell membrane. Bacteria can sense chemical gradients and move towards attractants or away from repellents, a process called chemotaxis. Adhesion to surfaces is crucial for bacterial colonization and biofilm formation. Bacteria use a variety of surface structures, such as pili and fimbriae, to attach to host cells or inanimate surfaces. Biofilms are complex communities of bacteria encased in a self-produced matrix of extracellular polymeric substances (EPS), which provide protection against antibiotics and host immune defenses.
Many thanks to our sponsor Esdebe who helped us prepare this research report.
3. Bacterial Metabolism and Genetic Diversity
3.1. Metabolic Versatility
Bacteria exhibit a remarkable diversity in metabolic pathways, enabling them to utilize a wide range of energy sources and thrive in diverse environments. Some bacteria are autotrophs, capable of synthesizing their own organic compounds from inorganic sources, such as carbon dioxide and sunlight (photoautotrophs) or inorganic chemicals (chemoautotrophs). Other bacteria are heterotrophs, obtaining energy and carbon from organic compounds. Bacteria can also utilize a variety of electron acceptors in respiration, including oxygen (aerobic respiration), nitrate (denitrification), sulfate (sulfate reduction), and carbon dioxide (methanogenesis). This metabolic flexibility allows bacteria to play essential roles in biogeochemical cycles, such as carbon and nitrogen cycling.
3.2. Mechanisms of Genetic Diversity
Bacteria are highly adaptable organisms due to their rapid reproduction rate and efficient mechanisms for generating genetic diversity. Mutations, arising from errors in DNA replication or repair, are a primary source of genetic variation. Horizontal gene transfer (HGT), the transfer of genetic material between unrelated bacteria, is another major driver of bacterial evolution. HGT can occur through three main mechanisms: transformation, transduction, and conjugation.
- Transformation: The uptake of naked DNA from the environment.
- Transduction: The transfer of DNA via bacteriophages (viruses that infect bacteria).
- Conjugation: The transfer of DNA through direct cell-to-cell contact via a pilus.
These mechanisms allow bacteria to acquire new genes, including those conferring antibiotic resistance, from other bacteria, even those of different species. The spread of antibiotic resistance genes through HGT is a major concern in the fight against antibiotic resistance.
3.3. The CRISPR-Cas System and Bacterial Immunity
Bacteria have also evolved defense mechanisms against invading DNA, such as the CRISPR-Cas system. CRISPR-Cas (Clustered Regularly Interspaced Short Palindromic Repeats and CRISPR-associated proteins) is an adaptive immune system that allows bacteria to recognize and destroy foreign DNA, such as that from bacteriophages or plasmids. The CRISPR-Cas system consists of a CRISPR array, a region of DNA containing short repeats separated by unique spacer sequences, and Cas proteins, which are enzymes that cleave DNA. When a bacterium is infected by a virus or plasmid, it can incorporate a fragment of the foreign DNA into its CRISPR array as a new spacer. If the bacterium is subsequently infected by the same virus or plasmid, the CRISPR-Cas system will recognize the foreign DNA and cleave it, preventing replication and protecting the bacterium. The CRISPR-Cas system is a powerful example of the ongoing evolutionary arms race between bacteria and their viruses.
Many thanks to our sponsor Esdebe who helped us prepare this research report.
4. Antibiotic Resistance Mechanisms
Antibiotic resistance is the ability of bacteria to survive and multiply in the presence of antibiotics that would normally kill or inhibit their growth. Antibiotic resistance can arise through a variety of mechanisms, including:
4.1. Target Modification
Bacteria can alter the target of an antibiotic, preventing it from binding and exerting its effect. For example, mutations in the genes encoding ribosomal proteins can prevent antibiotics like tetracycline and aminoglycosides from binding to the ribosome and inhibiting protein synthesis.
4.2. Antibiotic Inactivation
Bacteria can produce enzymes that inactivate antibiotics, rendering them ineffective. For example, beta-lactamases are enzymes that cleave the beta-lactam ring of penicillin and other beta-lactam antibiotics, preventing them from binding to their target, penicillin-binding proteins (PBPs), which are involved in cell wall synthesis.
4.3. Efflux Pumps
Bacteria can express efflux pumps, which are membrane proteins that actively pump antibiotics out of the cell, reducing their intracellular concentration and preventing them from reaching their target. Efflux pumps can confer resistance to a wide range of antibiotics.
4.4. Reduced Permeability
Bacteria can reduce the permeability of their outer membrane, preventing antibiotics from entering the cell. This is particularly important for Gram-negative bacteria, which have an outer membrane that acts as a barrier to many antibiotics. Mutations in porin proteins, which are channels in the outer membrane that allow antibiotics to enter the cell, can reduce antibiotic uptake.
4.5. Target Overproduction
Some bacteria can overcome the effects of antibiotics by overproducing the target of the antibiotic. For example, some bacteria can overproduce dihydrofolate reductase (DHFR), the target of trimethoprim, making the antibiotic less effective.
4.6. Bypass Pathways
Bacteria can evolve bypass pathways that circumvent the inhibited target of the antibiotic. This is a relatively rare mechanism, but it can confer high-level resistance.
4.7. The Role of Plasmids and Integrons
Many antibiotic resistance genes are located on plasmids, which can be readily transferred between bacteria through HGT. Integrons are genetic elements that can capture and express gene cassettes, often containing antibiotic resistance genes. Integrons play a significant role in the spread of antibiotic resistance genes in bacteria.
Many thanks to our sponsor Esdebe who helped us prepare this research report.
5. Bacterial Infections: A Spectrum of Disease
Bacterial infections can manifest in a wide range of clinical syndromes, affecting virtually every organ system. The severity of an infection depends on several factors, including the virulence of the pathogen, the site of infection, and the host’s immune status. Common bacterial infections include:
5.1. Respiratory Tract Infections
Pneumonia, bronchitis, and sinusitis are common respiratory tract infections caused by bacteria such as Streptococcus pneumoniae, Haemophilus influenzae, and Moraxella catarrhalis. Antibiotic resistance is a growing concern in the treatment of respiratory tract infections, particularly pneumonia caused by penicillin-resistant S. pneumoniae.
5.2. Urinary Tract Infections (UTIs)
UTIs are among the most common bacterial infections, particularly in women. Escherichia coli is the most common cause of UTIs, but other bacteria, such as Klebsiella pneumoniae and Staphylococcus saprophyticus, can also cause UTIs. Antibiotic resistance is a major challenge in the treatment of UTIs, with increasing rates of resistance to commonly used antibiotics such as trimethoprim-sulfamethoxazole and fluoroquinolones.
5.3. Skin and Soft Tissue Infections
Skin and soft tissue infections (SSTIs) can range from minor superficial infections to severe, life-threatening infections. Staphylococcus aureus and Streptococcus pyogenes are common causes of SSTIs. Methicillin-resistant S. aureus (MRSA) is a major concern in the treatment of SSTIs, particularly in hospital settings. Other bacteria, such as Pseudomonas aeruginosa and Clostridium perfringens, can also cause SSTIs, particularly in immunocompromised individuals.
5.4. Bloodstream Infections (Bacteremia and Sepsis)
Bacteremia is the presence of bacteria in the bloodstream. Sepsis is a life-threatening condition caused by the body’s overwhelming response to an infection, often leading to organ dysfunction. Bacteria such as E. coli, S. aureus, and K. pneumoniae are common causes of bacteremia and sepsis. Antibiotic resistance is a major factor contributing to the morbidity and mortality of sepsis.
5.5. Foodborne Illnesses
Foodborne illnesses, also known as food poisoning, are caused by consuming food contaminated with bacteria, viruses, or parasites. Bacteria such as Salmonella, Campylobacter, and E. coli are common causes of foodborne illnesses. Antibiotic resistance is a concern in the treatment of foodborne illnesses caused by bacteria such as Salmonella, as antibiotic treatment can sometimes prolong the duration of shedding of the bacteria.
5.6. Neurological Infections
Meningitis is an inflammation of the membranes surrounding the brain and spinal cord. Encephalitis is an inflammation of the brain. Bacteria such as Neisseria meningitidis, Streptococcus pneumoniae, and Haemophilus influenzae are common causes of meningitis. Antibiotic resistance is a concern in the treatment of meningitis, particularly in cases caused by penicillin-resistant S. pneumoniae.
Many thanks to our sponsor Esdebe who helped us prepare this research report.
6. The Microbiome and Antibiotic Resistance
The human microbiome, the community of microorganisms that live in and on the human body, plays a critical role in health and disease. The gut microbiome, in particular, is a complex ecosystem containing trillions of bacteria, archaea, fungi, and viruses. The gut microbiome performs many important functions, including:
- Aiding in digestion and nutrient absorption
- Producing vitamins and other essential nutrients
- Training and modulating the immune system
- Protecting against pathogens
Antibiotics can have a profound impact on the gut microbiome, disrupting its composition and function. Antibiotic treatment can reduce the diversity of the gut microbiome, leading to an overgrowth of opportunistic pathogens, such as Clostridium difficile. Antibiotic use can also select for antibiotic-resistant bacteria in the gut microbiome, which can then spread to other parts of the body or to other individuals.
6.1. Reservoirs of Resistance
The microbiome acts as a reservoir of antibiotic resistance genes, which can be transferred to pathogenic bacteria through HGT. Antibiotic resistance genes can persist in the microbiome even in the absence of antibiotic selection pressure, making it a long-term source of resistance. The widespread use of antibiotics in agriculture can also contribute to the spread of antibiotic resistance genes in the environment and the human microbiome.
6.2. Strategies for Microbiome Modulation
Strategies for modulating the gut microbiome, such as fecal microbiota transplantation (FMT) and probiotics, are being explored as potential treatments for antibiotic-resistant infections. FMT involves transferring fecal material from a healthy donor to a recipient, with the goal of restoring a healthy gut microbiome. Probiotics are live microorganisms that are intended to provide health benefits when consumed. While FMT has shown promise in treating C. difficile infections, the use of probiotics for preventing or treating antibiotic-resistant infections is still under investigation.
Many thanks to our sponsor Esdebe who helped us prepare this research report.
7. Emerging Bacterial Threats
Several bacterial pathogens are posing increasing threats to public health due to their high levels of antibiotic resistance and virulence. These include:
7.1. Carbapenem-Resistant Enterobacteriaceae (CRE)
CRE are a group of Gram-negative bacteria that are resistant to carbapenems, a class of broad-spectrum antibiotics that are often used as a last resort for treating infections caused by multidrug-resistant bacteria. CRE infections are associated with high mortality rates, particularly in immunocompromised individuals.
7.2. Acinetobacter baumannii
A. baumannii is a Gram-negative bacterium that is often resistant to multiple antibiotics, including carbapenems. A. baumannii infections are common in hospital settings and can cause pneumonia, bloodstream infections, and wound infections.
7.3. Pseudomonas aeruginosa
P. aeruginosa is a Gram-negative bacterium that is intrinsically resistant to many antibiotics. P. aeruginosa infections are common in patients with cystic fibrosis, burns, and immunocompromised individuals.
7.4. Neisseria gonorrhoeae
N. gonorrhoeae is the bacterium that causes gonorrhea, a sexually transmitted infection. N. gonorrhoeae has developed resistance to many antibiotics, including penicillin, tetracycline, and ciprofloxacin. Ceftriaxone is currently the recommended treatment for gonorrhea, but resistance to ceftriaxone is emerging, raising concerns about the future treatment of gonorrhea.
7.5. Clostridioides difficile
C. difficile is a bacterium that can cause diarrhea and colitis, particularly in individuals who have recently taken antibiotics. Antibiotic resistance is not a major problem in C. difficile infections, but the use of antibiotics can disrupt the gut microbiome and allow C. difficile to proliferate.
Many thanks to our sponsor Esdebe who helped us prepare this research report.
8. Strategies for Preventing the Spread of Resistant Bacteria
Preventing the spread of antibiotic-resistant bacteria requires a multifaceted approach, including:
8.1. Antibiotic Stewardship
Antibiotic stewardship programs aim to optimize antibiotic use, ensuring that antibiotics are used only when necessary and that the appropriate antibiotic is selected for the infection being treated. Antibiotic stewardship programs can reduce antibiotic use and the development of antibiotic resistance.
8.2. Infection Prevention and Control
Infection prevention and control measures, such as hand hygiene, isolation of patients with antibiotic-resistant infections, and environmental cleaning, can prevent the spread of antibiotic-resistant bacteria in healthcare settings.
8.3. Surveillance of Antibiotic Resistance
Surveillance of antibiotic resistance patterns is essential for tracking the emergence and spread of antibiotic-resistant bacteria. Surveillance data can be used to inform antibiotic stewardship programs and infection prevention and control measures.
8.4. Development of New Antibiotics
The development of new antibiotics is crucial for combating antibiotic resistance. However, the pipeline of new antibiotics is limited, and there is a need for new strategies for discovering and developing antibiotics.
8.5. Diagnostics
Rapid and accurate diagnostics are needed to identify bacterial infections and determine antibiotic susceptibility. This can help to ensure that patients receive the appropriate antibiotic treatment and prevent the unnecessary use of antibiotics.
8.6. Public Awareness
Raising public awareness about antibiotic resistance is important for promoting responsible antibiotic use and preventing the spread of antibiotic-resistant bacteria. Public awareness campaigns can educate people about the importance of hand hygiene, vaccination, and other measures to prevent infections.
Many thanks to our sponsor Esdebe who helped us prepare this research report.
9. The Role of AI in Predicting Antibiotic Resistance
Artificial intelligence (AI) is rapidly emerging as a powerful tool for predicting and combating antibiotic resistance. AI algorithms can analyze large datasets of genomic, clinical, and environmental data to identify patterns and predict the emergence and spread of antibiotic resistance.
9.1. Predicting Resistance Genes
AI can be used to predict the presence of antibiotic resistance genes in bacterial genomes based on their sequence. Machine learning algorithms can be trained on datasets of known resistance genes and their corresponding sequences to identify new resistance genes.
9.2. Predicting Resistance Phenotypes
AI can be used to predict antibiotic resistance phenotypes (the susceptibility of bacteria to antibiotics) based on their genotype (the genetic makeup of the bacteria). Machine learning algorithms can be trained on datasets of bacterial genomes and their corresponding antibiotic susceptibility profiles to predict the resistance phenotypes of new isolates.
9.3. Optimizing Antibiotic Treatment
AI can be used to optimize antibiotic treatment by predicting the most effective antibiotic for a given infection based on the patient’s clinical data and the pathogen’s antibiotic susceptibility profile. AI can also be used to predict the likelihood of treatment failure and to identify patients who are at high risk of developing antibiotic-resistant infections.
9.4. Monitoring Resistance Spread
AI can be used to monitor the spread of antibiotic resistance by analyzing data from various sources, such as electronic health records, laboratory databases, and social media. AI can identify outbreaks of antibiotic-resistant infections and track the movement of resistance genes between bacteria.
9.5. Drug Discovery
AI can be used to accelerate the discovery of new antibiotics by identifying potential drug targets and predicting the activity of novel compounds. AI can also be used to optimize the design of new antibiotics to improve their efficacy and reduce the likelihood of resistance development.
While AI holds great promise for combating antibiotic resistance, there are also challenges that need to be addressed. These include the need for large, high-quality datasets, the development of robust and reliable AI algorithms, and the integration of AI into clinical practice.
Many thanks to our sponsor Esdebe who helped us prepare this research report.
10. Conclusion
Bacteria are essential components of life on Earth, playing critical roles in biogeochemical cycles, symbiotic relationships, and pathogenesis. The emergence and spread of antibiotic-resistant bacteria pose a significant threat to global public health. Addressing this threat requires a multifaceted approach, including antibiotic stewardship, infection prevention and control, surveillance of antibiotic resistance, development of new antibiotics, and public awareness campaigns. Artificial intelligence (AI) is rapidly emerging as a powerful tool for predicting and combating antibiotic resistance, offering the potential to optimize antibiotic treatment, monitor resistance spread, and accelerate the discovery of new antibiotics. A combination of prudent antibiotic usage, innovative therapeutic strategies, and AI-driven predictive modelling offer a path towards mitigating the antibiotic resistance crisis and safeguarding public health.
Many thanks to our sponsor Esdebe who helped us prepare this research report.
References
- Alekshun, M. N., & Levy, S. B. (2007). Molecular mechanisms of antibacterial multidrug resistance. Cell, 128(6), 1037-1050.
- Blair, J. M. A., Webber, M. A., Baylay, A. J., Ogbolu, D. O., Piddock, L. J. V. (2015). Molecular mechanisms of antibiotic resistance. Nature Reviews Microbiology, 13(1), 42-51.
- Bush, K. (2010). Antibiotic resistance mechanisms in gram-negative bacteria. American Journal of Infection Control, 38(4), S1-S4.
- Carlet, J., Collignon, P., Goldmann, D., Gyssens, I. C., Janssens, J. P., Pittet, D., … & Van Der Meer, J. W. (2011). Society’s failure to protect a precious resource: antibiotics. The Lancet, 378(9788), 369-371.
- Fair, R. J., & Tor, Y. (2014). Antibiotics and bacterial resistance in the 21st century. Perspectives in medicinal chemistry, 6, 25-64.
- Founou, R. C., Founou, L. L., & Essack, S. Y. (2017). Antibiotic resistance in the food chain: a developing country perspective. Frontiers in Microbiology, 7, 2105.
- Gupta, V., Patel, M., Adalja, A. A., & Haass, K. A. (2019). Prioritizing antimicrobial stewardship interventions: an operational framework for healthcare facilities. Infection Control & Hospital Epidemiology, 40(1), 1-7.
- Holmes, A. H., Moore, C. E., Sundsfjord, A., Steinbakk, M., Regmi, S., Karkey, A., … & Dougan, G. (2016). Antimicrobial resistance in lower-income countries. The Lancet, 387(10019), 690-701.
- Lewis, K. (2013). Platforms for antibiotic discovery. Nature Reviews Drug Discovery, 12(5), 371-387.
- O’Neill, J. (2016). Tackling drug-resistant infections globally: final report and recommendations. Review on Antimicrobial Resistance. https://amr-review.org/sites/default/files/160525_Final%20paper_with%20cover.pdf
- Ventola, C. L. (2015). The antibiotic resistance crisis: part 1: causes and threats. Pharmacy and Therapeutics, 40(4), 277.
- Zaman, S., Hussain, M. A., Nye, R., Mehta, V., Mamun, Y., & Hossain, N. (2017). A review on antibiotic resistance: alarm bells are ringing. Cureus, 9(6).
So, bacteria are evolving defenses faster than we can invent new antibiotics? Are we sure we’re not accidentally training superbugs with all that hand sanitizer? Maybe we should all start hugging trees again – for science, of course!