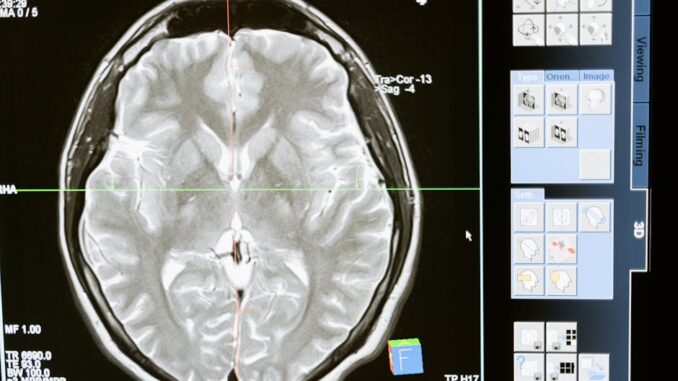
Abstract
Radiation, encompassing a broad spectrum of electromagnetic waves and particulate emissions, plays a pivotal role across diverse scientific and technological domains. This report provides a comprehensive overview of radiation, delving into its fundamental properties, various types, and the mechanisms of interaction with matter. Beyond foundational aspects, the report investigates cutting-edge advancements in radiation-based technologies, spanning medical imaging and therapy, industrial applications, and space exploration. Emphasis is placed on emerging techniques like intraoperative radiation therapy (IORT) and the development of advanced radiation delivery platforms that enhance precision and minimize collateral damage. Furthermore, the report critically assesses the biological effects of radiation, exploring both the short-term and long-term consequences of exposure, including the underlying mechanisms of radiation-induced damage and the evolving landscape of radiation safety protocols. Finally, the report examines the ethical considerations associated with radiation technologies and highlights future research directions aimed at optimizing their benefits while mitigating potential risks.
Many thanks to our sponsor Esdebe who helped us prepare this research report.
1. Introduction
Radiation, in its broadest sense, refers to the emission or transmission of energy in the form of waves or particles through space or a material medium. This phenomenon underpins a vast array of scientific and technological applications, ranging from medical imaging and cancer therapy to industrial non-destructive testing and space exploration. However, the dual nature of radiation – its potential for both immense benefit and significant harm – necessitates a comprehensive understanding of its fundamental properties, interaction mechanisms, and biological effects.
Historically, the discovery of X-rays by Wilhelm Röntgen in 1895 marked a paradigm shift in medical diagnostics and ushered in the era of modern radiation science. Subsequent discoveries of radioactivity by Henri Becquerel and Marie Curie further expanded the understanding of radiation and its potential applications. Over the past century, significant advancements have been made in radiation technology, driven by the need for more precise and effective tools for diagnosis, treatment, and research.
This report aims to provide a comprehensive overview of radiation, encompassing its fundamental properties, diverse applications, biological effects, and the evolving landscape of radiation safety. By examining both the benefits and risks associated with radiation exposure, this report seeks to inform future research and development efforts aimed at optimizing the use of radiation technologies for the betterment of society.
Many thanks to our sponsor Esdebe who helped us prepare this research report.
2. Fundamentals of Radiation
Radiation can be broadly classified into two main categories: electromagnetic radiation and particulate radiation. Electromagnetic radiation encompasses a wide spectrum of energy, ranging from low-frequency radio waves to high-frequency gamma rays. Each type of electromagnetic radiation is characterized by its wavelength and frequency, which are inversely proportional to each other and related to the energy of the radiation through Planck’s equation: E = hf, where E is energy, h is Planck’s constant, and f is frequency. Particulate radiation, on the other hand, consists of subatomic particles such as alpha particles, beta particles, neutrons, and protons.
2.1 Electromagnetic Radiation
The electromagnetic spectrum spans a wide range of frequencies and wavelengths, each with distinct properties and applications. Radio waves, with their long wavelengths and low frequencies, are primarily used for communication purposes, including broadcasting, telecommunications, and radar. Microwaves, with shorter wavelengths and higher frequencies, are used in cooking, radar, and satellite communications. Infrared radiation, located between microwaves and visible light, is associated with heat and is used in thermal imaging and remote sensing.
Visible light, the portion of the electromagnetic spectrum that is visible to the human eye, consists of different colors, each corresponding to a specific wavelength. Ultraviolet (UV) radiation, located beyond visible light, is energetic enough to cause sunburn and can lead to skin cancer with prolonged exposure. X-rays and gamma rays, with their short wavelengths and high frequencies, are highly penetrating and are used in medical imaging, radiation therapy, and industrial radiography.
2.2 Particulate Radiation
Particulate radiation consists of subatomic particles that possess kinetic energy and can interact with matter through various mechanisms. Alpha particles, consisting of two protons and two neutrons, are emitted during the radioactive decay of heavy nuclei. Due to their relatively large mass and charge, alpha particles have a short range in matter and are easily stopped by a sheet of paper. Beta particles, which are high-energy electrons or positrons, are emitted during the radioactive decay of certain isotopes. Beta particles are more penetrating than alpha particles but can be stopped by a thin sheet of metal. Neutrons, being neutral particles, can penetrate deeply into matter and are used in nuclear reactors and research facilities. Protons, which are positively charged particles, are used in proton therapy for cancer treatment.
2.3 Interaction of Radiation with Matter
Radiation interacts with matter through various mechanisms, depending on the type of radiation and the properties of the material. Electromagnetic radiation can be absorbed, scattered, or transmitted by matter, depending on the wavelength and frequency of the radiation and the atomic structure of the material. Photoelectric effect, Compton scattering, and pair production are three major processes of interaction between X-rays/gamma rays and matter. Particulate radiation interacts with matter through ionization and excitation of atoms, leading to the deposition of energy and potential damage to the material.
Many thanks to our sponsor Esdebe who helped us prepare this research report.
3. Applications of Radiation
Radiation has a wide range of applications across diverse fields, including medicine, industry, agriculture, and research.
3.1 Medical Applications
Radiation plays a crucial role in medical diagnostics and therapy. Medical imaging techniques, such as X-ray radiography, computed tomography (CT), magnetic resonance imaging (MRI), and positron emission tomography (PET), utilize radiation to visualize internal organs and tissues, enabling the detection and diagnosis of various diseases. Radiation therapy, also known as radiotherapy, uses high-energy radiation to kill cancer cells and shrink tumors. External beam radiation therapy (EBRT) delivers radiation from outside the body, while brachytherapy involves placing radioactive sources directly into or near the tumor.
Intraoperative Radiation Therapy (IORT): A significant advancement in radiation therapy is IORT, where a concentrated dose of radiation is delivered directly to the tumor bed during surgery. This approach allows for precise targeting of residual cancer cells while minimizing damage to surrounding healthy tissues. IORT has shown promising results in the treatment of various cancers, including breast cancer, pancreatic cancer, and sarcoma. Newer platforms are focusing on delivering IORT with enhanced precision, using techniques like electron beam IORT and targeted radioactive implants. Careful dosage optimization and rigorous safety protocols are essential for the effective and safe implementation of IORT. Comparative studies are ongoing to assess the effectiveness of different IORT systems and to identify the optimal treatment strategies for various cancer types. Concerns over long-term side effects, although likely less frequent with precise targeting, are also a focus of continued research.
3.2 Industrial Applications
Radiation is widely used in various industrial applications, including non-destructive testing, sterilization, and gauging. Non-destructive testing (NDT) techniques, such as radiography and gamma-ray testing, utilize radiation to inspect the integrity of materials and components without causing damage. Radiation sterilization is used to eliminate microorganisms from medical devices, pharmaceuticals, and food products. Radiation gauging is used to measure the thickness, density, and level of materials in various industrial processes.
3.3 Agricultural Applications
Radiation is used in agriculture for various purposes, including food irradiation, insect control, and plant breeding. Food irradiation uses ionizing radiation to kill bacteria, viruses, and insects in food products, extending their shelf life and improving food safety. Radiation is also used to sterilize insects, preventing them from reproducing and reducing their populations. In plant breeding, radiation is used to induce mutations in plants, leading to the development of new varieties with improved traits.
3.4 Research Applications
Radiation is an indispensable tool in scientific research, enabling researchers to probe the structure and properties of matter at the atomic and subatomic levels. X-ray diffraction is used to determine the crystal structure of materials. Radioactive tracers are used to study metabolic processes in living organisms. Particle accelerators, such as cyclotrons and synchrotrons, are used to accelerate particles to high energies for fundamental research in nuclear and particle physics.
Many thanks to our sponsor Esdebe who helped us prepare this research report.
4. Biological Effects of Radiation
Radiation can have both beneficial and detrimental effects on living organisms. The biological effects of radiation depend on several factors, including the type of radiation, the dose rate, the total dose, and the sensitivity of the tissue or organ exposed. Exposure to high doses of radiation can cause acute radiation sickness, while long-term exposure to lower doses can increase the risk of cancer and other health problems.
4.1 Mechanisms of Radiation-Induced Damage
Radiation primarily damages cells by ionizing atoms and molecules, leading to the formation of free radicals and the disruption of cellular processes. DNA is a particularly vulnerable target of radiation damage. Direct damage to DNA can lead to mutations, chromosomal aberrations, and cell death. Indirect damage to DNA occurs when free radicals react with DNA, causing oxidative damage and strand breaks. The cell has mechanisms to repair DNA damage, but if the damage is too extensive or the repair mechanisms are impaired, the cell may undergo apoptosis (programmed cell death) or become cancerous.
4.2 Acute Radiation Effects
Acute radiation effects occur shortly after exposure to high doses of radiation. The severity of acute radiation effects depends on the dose received and the organs exposed. Symptoms of acute radiation sickness can include nausea, vomiting, fatigue, hair loss, and skin burns. In severe cases, acute radiation sickness can be fatal. The hematopoietic system, the gastrointestinal system, and the central nervous system are particularly sensitive to radiation damage.
4.3 Long-Term Radiation Effects
Long-term radiation effects can occur years or even decades after exposure to radiation. The most significant long-term effect of radiation exposure is an increased risk of cancer. Radiation can damage DNA and cause mutations that lead to uncontrolled cell growth. The risk of cancer increases with increasing radiation dose. Other long-term effects of radiation exposure include cardiovascular disease, cataracts, and genetic mutations that can be passed on to future generations. The linear no-threshold (LNT) model is often used to estimate cancer risk from low doses of radiation, although the validity of this model at very low doses is still debated.
4.4 Radiation Sensitivity
Different tissues and organs exhibit varying degrees of sensitivity to radiation. Tissues with rapidly dividing cells, such as bone marrow, intestinal lining, and reproductive organs, are generally more sensitive to radiation than tissues with slowly dividing cells, such as muscle and nerve tissue. Children are more sensitive to radiation than adults due to their rapidly developing tissues and organs. The fetus is particularly vulnerable to radiation damage, especially during the early stages of development.
Many thanks to our sponsor Esdebe who helped us prepare this research report.
5. Radiation Safety and Protection
Protecting individuals and the environment from the harmful effects of radiation is of paramount importance. Radiation safety protocols and regulations are designed to minimize radiation exposure and prevent accidents. The principles of radiation protection are based on the ALARA (As Low As Reasonably Achievable) principle, which states that radiation exposure should be kept as low as reasonably achievable, taking into account economic and social factors.
5.1 Radiation Monitoring and Measurement
Radiation monitoring and measurement are essential for ensuring radiation safety. Radiation detectors, such as Geiger counters, ionization chambers, and scintillation detectors, are used to measure radiation levels and detect radioactive contamination. Personal dosimeters, such as film badges and thermoluminescent dosimeters (TLDs), are worn by individuals working with radiation to monitor their radiation exposure.
5.2 Shielding and Containment
Shielding and containment are used to reduce radiation exposure. Shielding materials, such as lead, concrete, and water, absorb radiation and reduce its intensity. Radioactive materials are typically contained in sealed containers to prevent leakage and contamination. Engineering controls, such as ventilation systems and remote handling equipment, are used to minimize worker exposure to radiation.
5.3 Regulations and Guidelines
Radiation safety is regulated by national and international organizations. The International Commission on Radiological Protection (ICRP) provides recommendations for radiation protection standards. National regulatory agencies, such as the Nuclear Regulatory Commission (NRC) in the United States, establish and enforce regulations governing the use of radioactive materials and radiation-producing devices. These regulations cover various aspects of radiation safety, including licensing, training, monitoring, and emergency response.
5.4 Public Perception and Communication
The public perception of radiation is often influenced by fear and misinformation. Effective communication about radiation risks and benefits is essential for building public trust and promoting informed decision-making. It is important to communicate radiation risks in a clear, concise, and understandable manner, avoiding technical jargon and focusing on the practical implications of radiation exposure. Engaging the public in discussions about radiation safety issues and addressing their concerns can help to alleviate anxiety and promote responsible use of radiation technologies.
Many thanks to our sponsor Esdebe who helped us prepare this research report.
6. Emerging Trends and Future Directions
Radiation science and technology are constantly evolving, with new advancements being made in various areas. Several emerging trends and future directions are shaping the field of radiation.
6.1 Advanced Radiation Therapy Techniques
Advancements in radiation therapy are focused on improving the precision and effectiveness of treatment while minimizing side effects. Intensity-modulated radiation therapy (IMRT) allows for precise shaping of the radiation beam to conform to the tumor, sparing surrounding healthy tissues. Stereotactic radiosurgery (SRS) delivers high doses of radiation to small, well-defined tumors with extreme accuracy. Particle therapy, using protons or heavy ions, offers the advantage of delivering a more localized dose of radiation to the tumor, reducing damage to surrounding tissues.
6.2 Development of Novel Radiation Detectors
The development of novel radiation detectors is crucial for improving radiation monitoring and imaging capabilities. New detector technologies, such as silicon photomultipliers (SiPMs) and cadmium zinc telluride (CZT) detectors, offer improved sensitivity, resolution, and energy discrimination. These detectors are being used in medical imaging, homeland security, and environmental monitoring.
6.3 Applications of Artificial Intelligence in Radiation
Artificial intelligence (AI) is playing an increasingly important role in radiation science and technology. AI algorithms are being used for image reconstruction, treatment planning, and dose optimization in radiation therapy. AI can also be used to automate radiation monitoring and detect anomalies in radiation data. The application of AI in radiation science has the potential to improve the efficiency, accuracy, and safety of radiation-based technologies.
6.4 Space Radiation Research
Space radiation poses a significant challenge for human space exploration. Understanding the biological effects of space radiation and developing effective countermeasures are critical for ensuring the health and safety of astronauts. Research is focused on studying the effects of cosmic rays and solar particle events on living organisms and developing shielding materials and pharmaceutical interventions to protect astronauts from radiation exposure during long-duration space missions. Future research may also explore the use of in-situ resource utilization (ISRU) on planetary surfaces to provide radiation shielding for habitats.
Many thanks to our sponsor Esdebe who helped us prepare this research report.
7. Ethical Considerations
The use of radiation technologies raises several ethical considerations that must be carefully addressed. The potential benefits of radiation technologies must be weighed against the risks of radiation exposure. Informed consent is essential for medical procedures involving radiation. Ensuring equitable access to radiation technologies and preventing their misuse are also important ethical considerations. The long-term environmental impacts of radiation technologies, such as nuclear power and radioactive waste disposal, must be carefully assessed and mitigated. Public engagement and stakeholder involvement are crucial for addressing ethical concerns and promoting responsible use of radiation technologies.
Many thanks to our sponsor Esdebe who helped us prepare this research report.
8. Conclusion
Radiation, with its diverse forms and properties, has revolutionized various fields, from medicine to industry and research. While the benefits are undeniable, the potential for harm necessitates a thorough understanding of its biological effects and the implementation of stringent safety protocols. This report has highlighted the advancements in radiation therapy, the applications of radiation in diverse sectors, and the evolving landscape of radiation safety. Moving forward, continued research and development, coupled with ethical considerations and public engagement, are essential for optimizing the use of radiation technologies for the benefit of society while minimizing potential risks. The future of radiation science lies in the pursuit of precision, safety, and innovation, guided by a commitment to responsible stewardship and the betterment of human health and the environment.
Many thanks to our sponsor Esdebe who helped us prepare this research report.
References
- Attix, F. H. (1986). Introduction to Radiological Physics and Radiation Dosimetry. John Wiley & Sons.
- Hall, E. J., & Giaccia, A. J. (2019). Radiobiology for the Radiologist. Lippincott Williams & Wilkins.
- IAEA. (2018). Radiation Protection and Safety of Radiation Sources: International Basic Safety Standards. IAEA Safety Standards Series No. GSR Part 3.
- Joiner, M. C., & van der Kogel, A. J. (2018). Basic Clinical Radiobiology. CRC press.
- Khan, F. M. (2009). The Physics of Radiation Therapy. Lippincott Williams & Wilkins.
- Mettler, F. A., Huda, W., Yoshizumi, T. T., & Mahesh, M. (2008). Medical exposure. Radiology, 248(3), 654-663.
- Morgan, W. F. (2003). Non-targeted and delayed effects of exposure to ionizing radiation: I. radiation-induced genomic instability and bystander effects in vitro. Radiation research, 159(5), 567-580.
- National Council on Radiation Protection and Measurements (NCRP). (1993). Limitation of Exposure to Ionizing Radiation. NCRP Report No. 116.
- Steel, G. G. (2002). Basic Clinical Radiobiology. Hodder Arnold.
- UNSCEAR. (2000). Sources and Effects of Ionizing Radiation. United Nations Scientific Committee on the Effects of Atomic Radiation Report to the General Assembly.
- Durante, M., & Formenti, S. C. (2018). Charged-particle therapy: A clinical perspective. Nature Reviews Clinical Oncology, 15(1), 48-61.
- Verma, V., Tendulkar, R. D., & Badiyan, S. N. (2018). Modern radiation therapy techniques for early-stage breast cancer. Seminars in Oncology, 45(3), 159-169.
- Thomadsen, B. R., & Rivard, M. J. (2005). Intraoperative radiotherapy: physics. Medical Physics, 32(8), 2629-2644.
The ethical considerations highlight the importance of public engagement. Exploring innovative methods for communicating complex information about radiation risks and benefits could foster a more informed public dialogue and promote responsible innovation in the field.
I appreciate you highlighting the importance of public engagement, it’s a critical aspect often overlooked. I wonder what methods you think would be the most effective in communicating these complex topics to the public and in a way that builds trust and encourages open conversation?
Editor: MedTechNews.Uk
Thank you to our Sponsor Esdebe
The report’s overview of radiation’s role in medical imaging and therapy is insightful. Exploring the potential of AI in optimizing radiation treatment plans to further minimize collateral damage warrants deeper investigation.