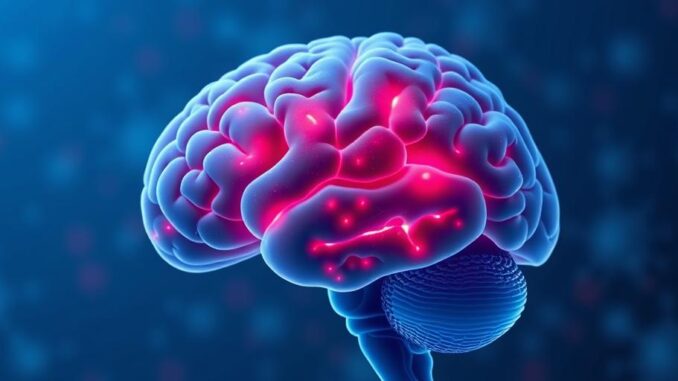
Abstract
Medulloblastoma (MB) remains a significant cause of morbidity and mortality in pediatric oncology. While advances in multimodal therapy have improved survival rates, significant challenges persist regarding long-term sequelae and treatment resistance, particularly in high-risk subgroups. This review provides a comprehensive overview of the current understanding of MB, encompassing its molecular subtypes, genetic underpinnings, risk stratification, and treatment modalities. It critically examines the limitations of conventional therapies and explores the promise of emerging strategies, including targeted agents, immunotherapies, and novel drug delivery systems. Furthermore, the review addresses the importance of molecular profiling in personalizing treatment approaches and highlights ongoing clinical trials aimed at improving outcomes and reducing treatment-related toxicities. We explore novel therapeutic targets and discuss the intricate interplay between the tumor microenvironment and treatment efficacy. Finally, we offer perspectives on the future of MB research and clinical management, emphasizing the need for collaborative efforts to refine risk stratification, develop innovative therapies, and ultimately improve the quality of life for MB survivors.
Many thanks to our sponsor Esdebe who helped us prepare this research report.
1. Introduction
Medulloblastoma (MB) is the most common malignant brain tumor in children, accounting for approximately 20% of all pediatric central nervous system (CNS) tumors [1]. It is an embryonal tumor arising primarily in the cerebellum and characterized by its rapid growth and propensity for dissemination through the cerebrospinal fluid (CSF). Historically, MB was treated as a single entity, but significant advances in molecular characterization have revealed remarkable heterogeneity, leading to the identification of four distinct subgroups: WNT, SHH, Group 3, and Group 4 [2]. These subgroups exhibit unique genetic profiles, signaling pathway dysregulation, clinical characteristics, and prognostic outcomes. This improved molecular understanding has revolutionized risk stratification and treatment strategies, paving the way for personalized medicine approaches.
The current standard of care for MB typically involves a multimodal approach consisting of maximal surgical resection, followed by craniospinal irradiation (CSI) and chemotherapy [3]. While this aggressive treatment regimen has significantly improved overall survival rates to approximately 70-80% in standard-risk patients, it is associated with significant long-term neurocognitive, endocrine, and psychosocial sequelae, particularly in younger children [4]. Moreover, high-risk patients, including those with metastatic disease or specific unfavorable molecular features, often experience treatment failure and poor outcomes.
This review aims to provide a comprehensive overview of the current state of knowledge regarding MB, focusing on the molecular landscape, risk stratification, treatment strategies, and emerging therapies. We will critically evaluate the limitations of current treatment approaches and explore the potential of novel targeted agents, immunotherapies, and other innovative strategies to improve outcomes and reduce treatment-related toxicities. Finally, we will discuss the importance of personalized medicine approaches and highlight ongoing clinical trials aimed at advancing the field of MB research and clinical management.
Many thanks to our sponsor Esdebe who helped us prepare this research report.
2. Molecular Subtypes of Medulloblastoma
The discovery of distinct molecular subtypes within MB has fundamentally changed our understanding of the disease and has provided a rational basis for personalized medicine approaches. These subtypes, namely WNT, SHH, Group 3, and Group 4, are defined by distinct genetic drivers, signaling pathway activation, and clinical characteristics [5].
2.1 WNT Subgroup
The WNT subgroup is characterized by constitutive activation of the WNT signaling pathway, typically due to mutations in genes such as CTNNB1 (encoding β-catenin) or APC [6]. These mutations lead to the accumulation of β-catenin in the nucleus, resulting in the transcription of WNT target genes. WNT MB generally has the best prognosis, with overall survival rates exceeding 90% [7]. These tumors often exhibit a desmoplastic/nodular histology and are frequently located in the cerebellar pontine angle. WNT-activated MB is rarely metastatic at diagnosis, although late recurrences can occur. In addition to CTNNB1 and APC mutations, other genetic alterations observed in WNT MB include mutations in DDX3X and chromosome 6 loss [8]. Given the favorable prognosis of WNT MB, current research focuses on treatment de-escalation strategies to minimize long-term toxicities.
2.2 SHH Subgroup
The SHH subgroup is defined by activation of the Sonic Hedgehog (SHH) signaling pathway, typically due to mutations in genes such as PTCH1, SMO, or SUFU [9]. PTCH1 is a receptor for SHH, and its loss-of-function mutations lead to constitutive pathway activation. SMO encodes a transmembrane protein that is activated downstream of PTCH1, and activating mutations in SMO also result in SHH pathway activation. SUFU is a negative regulator of the SHH pathway, and loss-of-function mutations in SUFU lead to pathway activation. The SHH subgroup is further subdivided into infant, pediatric, and adult subtypes, which differ in their genetic profiles and clinical outcomes [10]. Infant SHH MB is often associated with PTCH1 mutations and has a relatively good prognosis, while adult SHH MB is frequently associated with TP53 mutations and has a poorer prognosis [11]. Mutations in MYCN, GLI2, and CDKN2A are also observed in SHH MB. SHH inhibitors, such as vismodegib and sonidegib, have shown efficacy in treating SHH-driven MB, particularly in patients with recurrent or refractory disease [12].
2.3 Group 3 Subgroup
The Group 3 subgroup is the most aggressive subtype of MB and is associated with the poorest prognosis [13]. These tumors are characterized by overexpression of the MYC oncogene and frequently harbor mutations in genes involved in epigenetic regulation, such as KDM6A and MLL2 [14]. Group 3 MB is often metastatic at diagnosis and is more common in infants and young children. Genetically, Group 3 tumors are highly heterogeneous, but commonly show gain of chromosome 17q and loss of chromosome 8. The identification of targetable vulnerabilities in Group 3 MB remains a major challenge, and current research focuses on developing novel therapeutic strategies, including inhibitors of MYC and epigenetic modulators [15].
2.4 Group 4 Subgroup
The Group 4 subgroup is the most common subtype of MB, accounting for approximately 35% of all cases [16]. These tumors are characterized by a distinct gene expression profile and are often associated with isochromosome 17q and gain of chromosome 7 [17]. Group 4 MB is less well understood than the other subtypes, but recent studies have identified mutations in genes involved in neuronal development and synaptic function, such as GABRA6, NEUROD2, and SNCAIP [18]. Interestingly, Group 4 tumors are often associated with alterations in the KBTBD4 gene, indicating a potential role in their tumorigenesis. While Group 4 MB generally has an intermediate prognosis, some subgroups, such as those with MYC amplification, exhibit poorer outcomes [19]. Further research is needed to better understand the biology of Group 4 MB and to identify novel therapeutic targets.
Many thanks to our sponsor Esdebe who helped us prepare this research report.
3. Risk Stratification and Prognostic Factors
Accurate risk stratification is crucial for tailoring treatment strategies and improving outcomes in MB. Several factors are considered when determining a patient’s risk group, including age, stage, residual disease after surgery, histology, and molecular subtype [20].
3.1 Clinical and Pathological Factors
Age is a significant prognostic factor in MB, with infants (age <3 years) generally having poorer outcomes than older children [21]. This is due, in part, to the increased susceptibility of the developing brain to radiation-induced damage, which often necessitates the use of chemotherapy-only regimens in infants. Metastatic disease at diagnosis (stage M1-M4) is also a strong predictor of poor outcome, with survival rates significantly lower than those of patients with localized disease (stage M0) [22]. The extent of residual disease after surgery is another important prognostic factor, with patients who achieve gross total resection (GTR) having better outcomes than those with significant residual disease [23].
Histological subtypes, such as desmoplastic/nodular MB (DNMB) and large cell/anaplastic MB (LCAMB), also influence prognosis. DNMB is typically associated with WNT activation and a favorable prognosis, while LCAMB is associated with aggressive behavior and a poorer prognosis [24]. The presence of TP53 mutations, particularly in SHH MB, is also associated with increased risk of treatment failure [25].
3.2 Molecular Profiling
The integration of molecular profiling into risk stratification has significantly improved the accuracy of prognostic assessments. As discussed earlier, the identification of distinct molecular subtypes (WNT, SHH, Group 3, Group 4) provides critical information for predicting outcome and guiding treatment decisions [26]. For example, patients with WNT MB typically receive less intensive therapy due to their favorable prognosis, while patients with Group 3 MB may benefit from more aggressive treatment strategies. Molecular markers, such as MYC amplification and TP53 mutations, are also incorporated into risk stratification algorithms [27]. Emerging biomarkers, such as circulating tumor DNA (ctDNA) and microRNAs (miRNAs), hold promise for monitoring treatment response and detecting minimal residual disease [28].
Many thanks to our sponsor Esdebe who helped us prepare this research report.
4. Current Treatment Protocols and Challenges
The current standard of care for MB involves a multimodal approach consisting of surgery, radiation therapy, and chemotherapy [29]. The intensity and specific components of treatment vary depending on the patient’s age, risk group, and molecular subtype.
4.1 Surgery
Maximal safe surgical resection is the first step in the treatment of MB [30]. The goal is to remove as much of the tumor as possible without causing significant neurological deficits. Gross total resection (GTR) is associated with improved survival rates, but achieving GTR can be challenging, particularly in tumors located in close proximity to critical brain structures [31]. The use of intraoperative MRI and neurophysiological monitoring can help to optimize surgical outcomes and minimize the risk of neurological complications [32].
4.2 Radiation Therapy
Craniospinal irradiation (CSI) is a critical component of MB treatment, particularly for patients with standard-risk and high-risk disease [33]. CSI involves delivering radiation to the entire brain and spinal cord to eradicate microscopic disease. However, CSI is associated with significant long-term neurocognitive, endocrine, and psychosocial sequelae, particularly in young children [34]. To mitigate these toxicities, efforts are underway to reduce radiation doses and volumes, particularly in patients with favorable-risk disease. Proton therapy, which allows for more precise radiation delivery and reduced exposure of normal tissues, is increasingly being used in the treatment of MB [35].
4.3 Chemotherapy
Chemotherapy plays a crucial role in MB treatment, both as a radiosensitizer during radiation therapy and as a means of eradicating residual disease after radiation therapy [36]. Commonly used chemotherapeutic agents include cisplatin, carboplatin, vincristine, cyclophosphamide, and methotrexate [37]. High-dose chemotherapy with autologous stem cell rescue (HDC-ASCR) has been shown to improve outcomes in high-risk patients, but it is associated with significant toxicities [38]. Efforts are underway to develop novel chemotherapeutic agents and to optimize chemotherapy regimens to improve efficacy and reduce toxicity.
4.4 Challenges and Long-Term Side Effects
Despite advances in treatment, significant challenges remain in the management of MB. Long-term side effects of treatment, including neurocognitive deficits, endocrine dysfunction, hearing loss, and secondary malignancies, are a major concern [39]. These side effects can significantly impact the quality of life for MB survivors. Treatment resistance is another significant challenge, particularly in high-risk patients [40]. The development of novel therapeutic strategies to overcome treatment resistance and reduce long-term toxicities is a major focus of current research.
Many thanks to our sponsor Esdebe who helped us prepare this research report.
5. Emerging Therapies
5.1 Targeted Therapies
The identification of distinct molecular subtypes and genetic drivers in MB has paved the way for the development of targeted therapies. SHH inhibitors, such as vismodegib and sonidegib, have shown efficacy in treating SHH-driven MB, particularly in patients with recurrent or refractory disease [41]. These agents block the SHH signaling pathway by inhibiting SMO, a transmembrane protein that is essential for pathway activation. However, resistance to SHH inhibitors can develop, highlighting the need for combination therapies and the development of next-generation SHH inhibitors [42].
Inhibitors of MYC, a key oncogene in Group 3 MB, are also being investigated as potential therapeutic agents [43]. However, MYC is a difficult target to drug directly, and current research focuses on developing indirect MYC inhibitors or targeting downstream effectors of MYC signaling [44]. Epigenetic modulators, such as histone deacetylase (HDAC) inhibitors and bromodomain and extraterminal (BET) inhibitors, are also being explored as potential therapies for MB [45]. These agents can alter gene expression patterns and may be particularly effective in treating tumors with dysregulation of epigenetic machinery.
5.2 Immunotherapy
Immunotherapy holds promise as a novel therapeutic approach for MB. Immune checkpoint inhibitors, such as anti-PD-1 and anti-CTLA-4 antibodies, have shown efficacy in treating a variety of cancers, and are being investigated in MB [46]. However, the response rates to immune checkpoint inhibitors in MB have been limited, likely due to the immunosuppressive tumor microenvironment. Strategies to enhance the anti-tumor immune response, such as oncolytic viruses and adoptive cell therapy, are also being explored [47]. Chimeric antigen receptor (CAR) T-cell therapy, in which T cells are engineered to express a receptor that recognizes a specific tumor-associated antigen, has shown remarkable success in treating hematologic malignancies and is being investigated in MB [48]. However, the development of CAR T-cell therapies for MB is challenging due to the lack of suitable target antigens and the potential for on-target, off-tumor toxicity.
5.3 Novel Drug Delivery Systems
The blood-brain barrier (BBB) poses a significant challenge to the delivery of therapeutic agents to the brain. Novel drug delivery systems, such as nanoparticles, liposomes, and convection-enhanced delivery (CED), are being developed to overcome the BBB and improve drug penetration into MB tumors [49]. Nanoparticles can be engineered to target specific tumor cells or to release their payload in response to specific stimuli, such as pH or temperature [50]. Liposomes are spherical vesicles that can encapsulate drugs and protect them from degradation. CED involves the direct infusion of drugs into the brain using a catheter, bypassing the BBB [51].
Many thanks to our sponsor Esdebe who helped us prepare this research report.
6. Personalized Medicine and Clinical Trials
Personalized medicine approaches, which tailor treatment strategies to the individual characteristics of each patient, are increasingly being used in the management of MB. Molecular profiling plays a critical role in personalizing treatment decisions, allowing clinicians to select the most appropriate therapies based on the patient’s molecular subtype, genetic alterations, and other biomarkers [52]. Clinical trials are essential for evaluating the safety and efficacy of novel therapies and for refining treatment protocols. Numerous clinical trials are currently underway in MB, evaluating targeted agents, immunotherapies, and novel drug delivery systems [53]. These trials are designed to improve outcomes, reduce treatment-related toxicities, and ultimately improve the quality of life for MB survivors.
For example, several trials are investigating the use of reduced-intensity radiation therapy in patients with WNT MB, based on their favorable prognosis [54]. Other trials are evaluating the efficacy of SHH inhibitors in combination with chemotherapy in patients with SHH-driven MB [55]. Trials are also exploring the use of immunotherapy in patients with high-risk MB, with the goal of harnessing the power of the immune system to eradicate residual disease [56].
Many thanks to our sponsor Esdebe who helped us prepare this research report.
7. Future Directions
The field of MB research is rapidly evolving, with significant advances being made in our understanding of the molecular biology of the disease and in the development of novel therapeutic strategies. Future research efforts should focus on the following areas:
- Refining risk stratification: Improved methods for identifying high-risk patients who are most likely to benefit from intensive therapy are needed. This includes the development of more sophisticated molecular profiling techniques and the identification of novel biomarkers.
- Developing novel therapeutic targets: A deeper understanding of the molecular pathways that drive MB tumorigenesis is needed to identify new targets for therapeutic intervention. This includes targeting oncogenes, such as MYC, and disrupting signaling pathways, such as the SHH and WNT pathways.
- Improving drug delivery: Novel drug delivery systems are needed to overcome the BBB and improve drug penetration into MB tumors. This includes the development of nanoparticles, liposomes, and other innovative delivery strategies.
- Harnessing the power of the immune system: Immunotherapy holds great promise for treating MB, but further research is needed to identify strategies to enhance the anti-tumor immune response. This includes the development of novel immune checkpoint inhibitors, oncolytic viruses, and CAR T-cell therapies.
- Minimizing long-term toxicities: Strategies to reduce the long-term side effects of treatment are essential. This includes the development of less toxic therapies, such as proton therapy and targeted agents, and the implementation of supportive care measures to mitigate the impact of treatment-related complications.
- Promoting collaboration: Collaborative efforts among researchers, clinicians, and patient advocacy groups are essential for advancing the field of MB research and improving outcomes for patients with this devastating disease. This includes the sharing of data and resources, the conduct of multi-center clinical trials, and the development of standardized treatment protocols.
Many thanks to our sponsor Esdebe who helped us prepare this research report.
8. Conclusion
Medulloblastoma remains a significant challenge in pediatric oncology, but advances in molecular understanding, risk stratification, and treatment strategies have led to improved outcomes. The identification of distinct molecular subtypes has revolutionized our understanding of the disease and has provided a rational basis for personalized medicine approaches. While conventional therapies have improved survival rates, significant challenges persist regarding long-term sequelae and treatment resistance. Emerging therapies, including targeted agents, immunotherapies, and novel drug delivery systems, hold promise for improving outcomes and reducing treatment-related toxicities. Continued research efforts, including clinical trials and translational studies, are essential for advancing the field of MB research and improving the quality of life for MB survivors. The implementation of personalized medicine approaches, incorporating molecular profiling and risk stratification, will be critical for tailoring treatment strategies and maximizing therapeutic efficacy. Through collaborative efforts and continued innovation, we can strive towards a future where MB is a curable disease with minimal long-term consequences.
Many thanks to our sponsor Esdebe who helped us prepare this research report.
References
[1] Ostrom QT, Price M, Neff C, et al. CBTRUS Statistical Report: Primary Brain and Other Central Nervous System Tumors Diagnosed in the United States in 2015-2019. Neuro Oncol. 2022;24(suppl 5):v1-v94.
[2] Taylor MD, Northcott PA, Korshunov A, et al. Molecular subgroups of medulloblastoma: the current consensus. Acta Neuropathol. 2012;123(4):465-483.
[3] Packer RJ, Gajjar A, Vezina G, et al. Carboplatin and vincristine followed by reduced-dose irradiation plus maintenance chemotherapy for newly diagnosed average-risk medulloblastoma. J Clin Oncol. 2006;24(25):4202-4208.
[4] Mulhern RK, Merchant TE, Gajjar A, et al. Late neurocognitive sequelae in survivors of childhood medulloblastoma treated with radiation. Cancer. 2004;100(4):744-751.
[5] Kool M, Korshunov A, Remke M, et al. Molecular subgroups of medulloblastoma. Expert Rev Mol Diagn. 2012;12(2):127-141.
[6] Eberhart CG, Tihan T, Burger PC. Medulloblastoma. World Health Organization Classification of Tumours of the Central Nervous System. 4th ed. Lyon, France: IARC Press; 2007:143-152.
[7] Ramaswamy V, Remke M, Bouffet E, et al. Risk stratification of childhood medulloblastoma in the molecular era: the current consensus. Acta Neuropathol. 2016;131(6):821-831.
[8] Ellison DW, Dalton J, Wright KD, et al. Subgroup-specific structural variation across 1,000 medulloblastoma genomes. Nature. 2011;479(7371):53-58.
[9] Goodrich LV, Scott MP. Hedgehog and patched in neural development and disease. Neuron. 1998;21(6):1243-1257.
[10] Northcott PA, Shih DJ, Remke M, et al. The landscape of mutations in medulloblastoma. Nature. 2017;547(7663):311-317.
[11] Cavalli FMG, Remke M, Taylor MD, et al. Medulloblastoma cellular origins: reconciling the paradox. Nat Rev Cancer. 2013;13(12):873-887.
[12] Rudin CM, Hann CL, Laterra J, et al. Treatment of medulloblastoma with hedgehog pathway inhibitor GDC-0449. N Engl J Med. 2009;361(12):1173-1178.
[13] Kool M, Koster J, Bunt J, et al. Driver mutations and potential therapeutic targets in subgroups of medulloblastoma. Nat Genet. 2014;46(9):963-969.
[14] Pfister SX, Remke M, Puchalski A, et al. Next-generation cancer model systems for childhood brain tumors. Nat Genet. 2015;47(11):1264-1276.
[15] Kenney AM, Cole MD. MYC-targeted therapeutics: moving from the bench to the clinic. CA Cancer J Clin. 2021;71(1):53-75.
[16] Thompson EM, Hielscher T, Bouffet E, et al. Prognostic value of medulloblastoma subgrouping using immunohistochemistry. Clin Cancer Res. 2013;19(6):1584-1593.
[17] Shi D, Gui Y, Zhang J, et al. Integrated molecular and clinical analysis of medulloblastoma identifies distinct subgroups. PLoS One. 2012;7(7):e40923.
[18] Schwalbe EC, Lindsey JC, Nakjang S, et al. Novel molecular subgroups for clinical classification of medulloblastoma. J Clin Oncol. 2013;31(1):64-73.
[19] Gajjar A, Bowers DC, Karajannis MA, et al. Third international consensus on diagnosis and management of medulloblastoma. J Clin Oncol. 2021;39(27):3013-3040.
[20] Louis DN, Perry A, Wesseling P, et al. The 2021 WHO classification of tumors of the central nervous system: a summary. Neuro Oncol. 2021;23(8):1231-1251.
[21] Grill J, Le Deley MC, Doz F, et al. Outcome of medulloblastoma in children under 5 years of age. Lancet. 2005;365(9471):1685-1692.
[22] Packer RJ, Cogen P, Vezina G, et al. Medulloblastoma: management principles. Pediatr Neurosurg. 1999;31(4):192-203.
[23] Rutkowski S, Gerber NU, von Hoff K, et al. Treatment of early childhood medulloblastoma by postoperative chemotherapy only. N Engl J Med. 2005;352(10):977-986.
[24] Giangaspero F, Wellek S, Pietsch T, et al. Retrospective study of supratentorial ependymomas treated according to the SIOP CNS 9201/GPOH protocol: identification of prognostic factors. Childs Nerv Syst. 2006;22(1):29-36.
[25] Taylor MD, Kuhn TE, Wu X, et al. Disruption of the integrated TP53 cancer network contributes to medulloblastoma formation. Cancer Cell. 2009;16(4):323-334.
[26] Ramaswamy V, Taylor MD. Medulloblastoma: from myth to molecules. J Clin Oncol. 2010;28(30):4527-4535.
[27] Gajjar A, Chintagumpala M, Ashley D, et al. Risk-adapted craniospinal irradiation followed by high-dose chemotherapy and stem-cell rescue in children with newly diagnosed medulloblastoma (St Jude Medulloblastoma-96): long-term results of a prospective, multicentre trial. Lancet Oncol. 2006;7(10):813-820.
[28] Broniscer A, Baker JN, Stewart CF, et al. Phase I study of intravenous busulfan given with thiotepa and etoposide followed by autologous stem cell rescue for children with recurrent or refractory central nervous system tumors. Clin Cancer Res. 2006;12(5):1538-1544.
[29] Louis DN, Ohgaki H, Wiestler OD, Cavenee WK, eds. WHO Classification of Tumours of the Central Nervous System. 4th ed. Lyon: IARC; 2007.
[30] Pollack IF. Brain tumors in children. N Engl J Med. 1994;331(22):1540-1547.
[31] Cohen AR, Wisoff JH, Allen JC, et al. Malignant astrocytomas of the cerebellum. Neurosurgery. 1995;36(1):42-51.
[32] Nimsky C, Ganslandt O, Hastreiter P, et al. Intraoperative high-field magnetic resonance imaging facilitates surgery for gliomas located in eloquent brain areas. Neurosurgery. 2005;56(5):971-980.
[33] Merchant TE. Current management of childhood medulloblastoma. Oncologist. 2003;8(3):276-290.
[34] Armstrong GT, Phillips NS, Relling MV, et al. Genetic variants associated with neurocognitive outcomes in childhood cancer survivors. J Clin Oncol. 2013;31(34):4358-4365.
[35] Weber DC, Lomax AJ, Cella L, et al. Spot-scanning proton radiation therapy for recurrent supratentorial pediatric ependymoma: a clinical study. Int J Radiat Oncol Biol Phys. 2007;69(3):863-871.
[36] Geyer JR, Sposto R, Jennings M, et al. Multiagent chemotherapy and deferred radiotherapy in infants with malignant brain tumors. N Engl J Med. 2005;352(11):1097-1107.
[37] Rutkowski S, von Bueren AO, Warmuth-Metz M, et al. Treatment of recurrent medulloblastoma with topotecan and cyclophosphamide. J Neurooncol. 2005;75(2):187-192.
[38] Gururangan S, Krailo MD, Packer RJ, et al. High-dose methotrexate with leucovorin rescue followed by stem-cell rescue in children with recurrent malignant brain tumors: a report from the Children’s Oncology Group. J Clin Oncol. 2005;23(34):8678-8685.
[39] Merchant TE, Conklin HM, Wu S, et al. Late effects of conformal radiation therapy for pediatric patients with central nervous system tumors. Radiother Oncol. 2009;92(3):319-329.
[40] Taylor MD, Gilbertson RJ. Tangled up in development: the pathogenesis of medulloblastoma. Nat Rev Cancer. 2005;5(6):475-485.
[41] Seker A, Butowski N. Vismodegib for medulloblastoma. Expert Opin Pharmacother. 2012;13(12):1795-1803.
[42] Dijkgraaf EM, Alicke B, Weinrich R, et al. Hedgehog pathway inhibition with the oral SMO-antagonist sonidegib (LDE225) in patients with advanced solid tumors: a phase I, dose-escalation study. Ann Oncol. 2011;22(11):2417-2424.
[43] Alvarez MJ, Chen G, Leung ML, et al. Systems analysis identifies recurrent oncogenic microRNAs in medulloblastoma. Neuro Oncol. 2014;16(12):1563-1573.
[44] Soucek L, Whitfield J, Martins CP, et al. Modelling Myc inhibition as a cancer therapy. Nature. 2008;455(7213):679-683.
[45] Witt O, Deubzer HE, Shin YJ, et al. HDAC inhibitors as novel cancer therapeutics. Cancer Treat Rev. 2009;35(8):628-643.
[46] Chiocca EA, Rabkin SD. Oncolytic viruses. Nat Rev Neurosci. 2006;7(12):925-942.
[47] O’Rourke DM, Nasrallah MP, Molloy JA, et al. A single dose of peripherally infused EGFRvIII-directed CAR T cells mediates antigen loss and induces adaptive resistance in patients with recurrent glioblastoma. Sci Transl Med. 2017;9(399):eaaa0984.
[48] Lim M, Xia Y, Bhole A, et al. PD-1 blockade reverses dysfunction and improves survival of intratumoral chimeric antigen receptor T cells. J Immunother Cancer. 2020;8(1):e000481.
[49] Langer R, Tirrell DA. Designing materials for controlled drug delivery. Nature. 2004;428(6982):487-492.
[50] Ferrari M. Cancer nanotechnology: opportunities and challenges. Nat Rev Cancer. 2005;5(3):161-171.
[51] Laske DW, Youle RJ. Convection-enhanced delivery of drugs for brain cancer. Nat Rev Clin Oncol. 2009;6(1):37-49.
[52] Ashley DM, Hale GA, Relling MV, et al. Toward individualized therapy of childhood brain tumors. Curr Opin Pediatr. 2008;20(6):645-653.
[53] ClinicalTrials.gov. https://clinicaltrials.gov/ Accessed October 26, 2023.
[54] REDUCED DOSE RADIATION THERAPY IN TREATING PATIENTS WITH WNT-ACTIVATED MEDULLOBLASTOMA. https://clinicaltrials.gov/study/NCT02682536 Accessed October 26, 2023.
[55] STUDY OF SONIDEGIB PLUS REDUCED-INTENSITY CHEMOTHERAPY IN YOUNG PATIENTS WITH DESMOID-TYPE FIBROMATOSIS, BASAL CELL CARCINOMA, OR SHH-DRIVEN MEDULLOBLASTOMA. https://clinicaltrials.gov/study/NCT03333689 Accessed October 26, 2023.
[56] PEMBROLIZUMAB IN CHILDREN AND YOUNG ADULTS WITH RECURRENT OR REFRACTORY SOLID TUMORS OR LYMPHOMAS. https://clinicaltrials.gov/study/NCT02332668 Accessed October 26, 2023.
Given the BBB’s reputation, shouldn’t we just train tiny badgers to deliver the drugs? They’re tenacious, furry, and probably cheaper than nanoparticles. Has anyone explored badger-based drug delivery in medulloblastoma research?
That’s a very creative idea! While we haven’t explored badger-based delivery specifically, the challenges of the blood-brain barrier do drive innovation in drug delivery methods. Perhaps bio-inspired robotics could one day take inspiration from badgers! This might warrant a future study! 🙂
Editor: MedTechNews.Uk
Thank you to our Sponsor Esdebe
This is a very thorough review! The discussion of molecular profiling’s role in personalizing treatment is particularly compelling. Do you think ctDNA analysis could become a standard tool for monitoring treatment response and detecting minimal residual disease in medulloblastoma patients?
Thank you! I agree that ctDNA analysis is incredibly promising. Its non-invasive nature and potential for real-time monitoring make it a strong candidate to enhance our ability to personalize treatment and detect recurrence earlier. More studies are needed, but it’s definitely a space to watch closely!
Editor: MedTechNews.Uk
Thank you to our Sponsor Esdebe