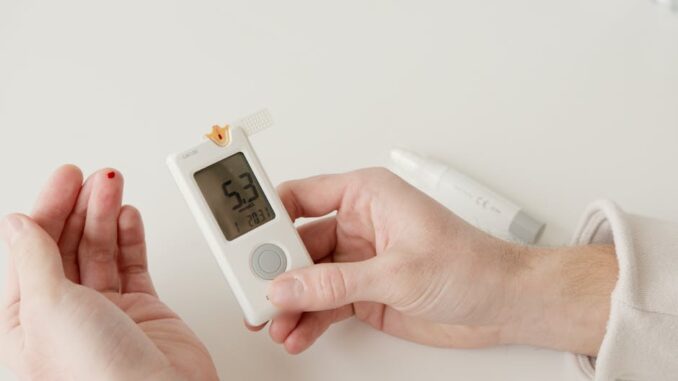
The Interplay of Metabolic Dysregulation and Cardiovascular Remodeling: A Comprehensive Review
Abstract
The escalating global prevalence of metabolic disorders, particularly type 2 diabetes mellitus (T2DM) and its precursor states like insulin resistance, poses a significant threat to cardiovascular health. This review delves into the complex and multifaceted relationship between metabolic dysregulation and cardiovascular remodeling, encompassing not only the direct effects of hyperglycemia but also the contributions of other metabolic disturbances, such as dyslipidemia, non-alcoholic fatty liver disease (NAFLD), and systemic inflammation. We examine the underlying molecular mechanisms driving these pathological processes, highlighting the roles of advanced glycation end-products (AGEs), oxidative stress, endoplasmic reticulum stress, and alterations in myocardial substrate utilization. Furthermore, we discuss the impact of sex differences on cardiovascular outcomes in the context of metabolic disease and evaluate current and emerging therapeutic strategies targeting both metabolic and cardiovascular dysfunction. Finally, we identify critical gaps in our understanding and suggest future research directions to improve prevention and treatment strategies for metabolic-related cardiovascular disease.
1. Introduction
Cardiovascular disease (CVD) remains the leading cause of morbidity and mortality worldwide. While traditional risk factors such as hypertension, hyperlipidemia, and smoking are well-established contributors to CVD, the increasing prevalence of metabolic disorders, including obesity, insulin resistance, and T2DM, has significantly amplified the burden of CVD. The intersection of metabolic dysregulation and cardiovascular pathophysiology represents a complex and evolving area of research, demanding a comprehensive understanding of the underlying mechanisms and the development of targeted therapeutic interventions.
Metabolic syndrome (MetS), characterized by a cluster of risk factors including abdominal obesity, insulin resistance, dyslipidemia, and hypertension, significantly increases the risk of CVD. The individual components of MetS, as well as their synergistic interactions, contribute to a pro-inflammatory and pro-thrombotic state, accelerating the development of atherosclerosis and increasing the likelihood of adverse cardiovascular events [1]. Moreover, the chronic hyperglycemia associated with T2DM directly damages the heart and vasculature, leading to the development of diabetic cardiomyopathy, coronary artery disease (CAD), and heart failure (HF) [2].
This review aims to provide an in-depth exploration of the interplay between metabolic dysregulation and cardiovascular remodeling. We will examine the specific mechanisms by which metabolic derangements contribute to cardiovascular dysfunction, discuss the impact of sex differences, and evaluate current and emerging therapeutic strategies. Finally, we will highlight key areas for future research to improve the prevention and treatment of metabolic-related CVD.
2. Mechanisms of Cardiovascular Remodeling in Metabolic Disease
Cardiovascular remodeling, a complex process involving alterations in cardiac structure and function, is a hallmark of metabolic disease. This remodeling encompasses both structural changes, such as cardiomyocyte hypertrophy and fibrosis, and functional impairments, including diastolic dysfunction and impaired contractility. Several interconnected mechanisms contribute to this remodeling process:
2.1. Advanced Glycation End-Products (AGEs) and Receptor for AGEs (RAGE) Axis:
Chronic hyperglycemia leads to the non-enzymatic glycation of proteins and lipids, resulting in the formation of AGEs. These AGEs accumulate in cardiac tissue, contributing to cross-linking of extracellular matrix proteins and impairing myocardial elasticity [3]. Furthermore, AGEs bind to the RAGE, a transmembrane receptor expressed on various cell types including cardiomyocytes, endothelial cells, and inflammatory cells. Activation of RAGE triggers intracellular signaling pathways, including NF-κB, leading to increased production of pro-inflammatory cytokines, oxidative stress, and extracellular matrix deposition [4]. The AGE-RAGE axis plays a crucial role in promoting cardiomyocyte hypertrophy, fibrosis, and endothelial dysfunction, contributing to the development of diabetic cardiomyopathy and atherosclerosis.
2.2. Oxidative Stress:
Metabolic dysregulation is associated with increased production of reactive oxygen species (ROS) and impaired antioxidant defense mechanisms, leading to oxidative stress. Hyperglycemia promotes ROS generation through several mechanisms, including increased glucose auto-oxidation, activation of NADPH oxidase, and mitochondrial dysfunction [5]. ROS can directly damage cellular components, including DNA, proteins, and lipids, leading to cellular dysfunction and apoptosis. In the heart, oxidative stress contributes to cardiomyocyte hypertrophy, fibrosis, and impaired contractility. Furthermore, ROS promotes endothelial dysfunction by reducing nitric oxide (NO) bioavailability and increasing the expression of adhesion molecules, contributing to the development of atherosclerosis [6].
2.3. Endoplasmic Reticulum (ER) Stress:
The ER is a cellular organelle responsible for protein folding and processing. Metabolic stress, including hyperglycemia and lipotoxicity, can disrupt ER homeostasis, leading to the accumulation of unfolded or misfolded proteins in the ER lumen, a condition known as ER stress [7]. ER stress activates a complex signaling pathway known as the unfolded protein response (UPR), which aims to restore ER homeostasis. However, prolonged or unresolved ER stress can trigger apoptosis and contribute to cellular dysfunction. In the heart, ER stress contributes to cardiomyocyte hypertrophy, apoptosis, and impaired contractility, exacerbating diabetic cardiomyopathy.
2.4. Myocardial Substrate Utilization:
In the healthy heart, fatty acids are the primary source of energy. However, in the context of metabolic disease, particularly T2DM, there is a shift in myocardial substrate utilization towards increased fatty acid oxidation and decreased glucose utilization. While fatty acid oxidation generates more ATP per molecule than glucose oxidation, it is also less efficient and requires more oxygen [8]. This increased reliance on fatty acid oxidation leads to increased production of ROS and impairs cardiac efficiency. Furthermore, the accumulation of lipid intermediates, such as diacylglycerol and ceramide, in cardiomyocytes can activate intracellular signaling pathways that promote insulin resistance and lipotoxicity, further contributing to cardiac dysfunction [9].
2.5. Inflammation:
Metabolic dysregulation is characterized by chronic low-grade inflammation, characterized by elevated levels of pro-inflammatory cytokines such as tumor necrosis factor-alpha (TNF-α), interleukin-1β (IL-1β), and interleukin-6 (IL-6). Adipose tissue, particularly visceral adipose tissue, is a major source of these pro-inflammatory cytokines. These cytokines activate intracellular signaling pathways, including NF-κB and MAPK, leading to increased production of ROS, endothelial dysfunction, and extracellular matrix remodeling [10]. Inflammation also promotes insulin resistance in cardiomyocytes and other tissues, further exacerbating metabolic dysfunction. The inflammasome, a multiprotein complex that activates IL-1β, plays a critical role in mediating inflammation in the context of metabolic disease.
3. Sex Differences in Metabolic-Related Cardiovascular Disease
Sex differences in cardiovascular risk and outcomes are well-documented. Women generally have a lower risk of CVD than men at younger ages, but this advantage diminishes after menopause. The mechanisms underlying these sex differences are complex and involve hormonal, genetic, and environmental factors [11].
In the context of metabolic disease, sex differences in cardiovascular risk and outcomes are particularly evident. Women with T2DM are at a significantly higher risk of CVD compared to men with T2DM. This increased risk may be related to several factors, including differences in body composition, hormonal status, and metabolic profiles. Women tend to have a higher percentage of body fat and a lower muscle mass than men, which may contribute to increased insulin resistance and dyslipidemia. Furthermore, the decline in estrogen levels after menopause increases the risk of CVD in women, partly due to its effects on lipid metabolism, endothelial function, and inflammation [12].
Sex differences also exist in the presentation and progression of CVD in the context of metabolic disease. Women with T2DM are more likely to develop heart failure with preserved ejection fraction (HFpEF) than men, while men are more likely to develop heart failure with reduced ejection fraction (HFrEF) [13]. HFpEF is characterized by diastolic dysfunction and impaired ventricular relaxation, while HFrEF is characterized by systolic dysfunction and reduced ventricular contractility. The underlying mechanisms contributing to these sex differences in HF phenotypes are not fully understood, but may involve differences in myocardial structure, fibrosis, and inflammation.
Furthermore, women with T2DM may have a more pronounced inflammatory response and a greater degree of endothelial dysfunction than men. This may contribute to the increased risk of microvascular complications, such as diabetic nephropathy and retinopathy, in women with T2DM. Future research is needed to further elucidate the mechanisms underlying sex differences in metabolic-related CVD and to develop sex-specific prevention and treatment strategies.
4. Diagnostic Tools and Risk Stratification
Early detection and risk stratification are crucial for preventing and managing metabolic-related CVD. A variety of diagnostic tools are available to assess cardiovascular function and identify individuals at high risk.
4.1. Electrocardiogram (ECG):
The ECG is a non-invasive test that records the electrical activity of the heart. It can detect arrhythmias, conduction abnormalities, and evidence of myocardial ischemia or infarction. While the ECG is a useful screening tool, it may not be sensitive enough to detect early stages of cardiovascular remodeling in metabolic disease.
4.2. Echocardiogram:
The echocardiogram uses ultrasound to visualize the heart and assess its structure and function. It can detect abnormalities in chamber size, wall thickness, valve function, and contractility. Echocardiography is particularly useful for assessing diastolic function, which is often impaired in the early stages of diabetic cardiomyopathy. Strain imaging, a more advanced echocardiographic technique, can detect subtle abnormalities in myocardial deformation that may not be apparent with conventional echocardiography [14].
4.3. Stress Tests:
Stress tests assess the heart’s response to exercise or pharmacological stress. They can detect myocardial ischemia and assess functional capacity. Stress echocardiography, which combines echocardiography with stress testing, can provide more detailed information about cardiac function during stress.
4.4. Coronary Computed Tomography Angiography (CCTA):
CCTA is a non-invasive imaging technique that uses X-rays and contrast dye to visualize the coronary arteries. It can detect coronary artery stenosis and assess plaque burden. CCTA is particularly useful for identifying individuals with subclinical CAD who may be at increased risk of future cardiovascular events.
4.5. Biomarkers:
Several biomarkers have been identified as potential predictors of cardiovascular risk in individuals with metabolic disease. These include natriuretic peptides (BNP and NT-proBNP), which are released in response to cardiac stress; troponins, which are released in response to myocardial injury; and inflammatory markers such as CRP and IL-6. Elevated levels of these biomarkers may indicate increased risk of cardiovascular events and can be used to guide treatment decisions.
4.6. Risk Scores:
Several risk scores have been developed to estimate the risk of cardiovascular events in individuals with metabolic disease. These risk scores typically incorporate traditional risk factors such as age, sex, blood pressure, cholesterol levels, and smoking status, as well as metabolic parameters such as HbA1c and albuminuria. The Framingham Risk Score, the UKPDS Risk Engine, and the SCORE risk charts are commonly used risk scores for assessing cardiovascular risk in individuals with T2DM [15].
5. Treatment Strategies
The management of metabolic-related CVD requires a multifaceted approach that addresses both metabolic dysfunction and cardiovascular risk factors. Treatment strategies include lifestyle modifications, pharmacotherapy, and in some cases, revascularization procedures.
5.1. Lifestyle Modifications:
Lifestyle modifications, including dietary changes and regular physical activity, are the cornerstone of treatment for metabolic disease and CVD. A healthy diet, rich in fruits, vegetables, whole grains, and lean protein, can improve glycemic control, reduce cholesterol levels, and promote weight loss. Regular physical activity, including both aerobic and resistance exercise, can improve insulin sensitivity, lower blood pressure, and reduce inflammation. Weight loss, even modest weight loss of 5-10%, can significantly improve metabolic parameters and reduce cardiovascular risk [16].
5.2. Pharmacotherapy:
A variety of medications are available to treat metabolic disease and CVD. These include:
- Antidiabetic Medications: Metformin, sulfonylureas, thiazolidinediones, DPP-4 inhibitors, SGLT2 inhibitors, and GLP-1 receptor agonists are used to improve glycemic control. SGLT2 inhibitors and GLP-1 receptor agonists have demonstrated significant cardiovascular benefits in clinical trials and are now recommended as first-line therapy for individuals with T2DM and established CVD or high cardiovascular risk [17].
- Lipid-Lowering Medications: Statins are the primary lipid-lowering medications used to reduce LDL cholesterol levels. Ezetimibe, PCSK9 inhibitors, and bempedoic acid can be used as adjunct therapy to further reduce LDL cholesterol levels. Fibrates and omega-3 fatty acids can be used to lower triglyceride levels [18].
- Antihypertensive Medications: ACE inhibitors, ARBs, beta-blockers, calcium channel blockers, and diuretics are used to lower blood pressure. ACE inhibitors and ARBs are particularly effective in reducing albuminuria and preventing diabetic nephropathy [19].
- Antiplatelet Medications: Aspirin and clopidogrel are used to prevent blood clots and reduce the risk of cardiovascular events. Dual antiplatelet therapy (DAPT) with aspirin and clopidogrel is typically used after percutaneous coronary intervention (PCI) or acute coronary syndrome (ACS).
- Heart Failure Medications: ACE inhibitors, ARBs, beta-blockers, mineralocorticoid receptor antagonists (MRAs), and angiotensin receptor-neprilysin inhibitors (ARNIs) are used to treat heart failure. SGLT2 inhibitors have also shown benefit in treating heart failure, regardless of diabetes status.
5.3. Revascularization Procedures:
Revascularization procedures, such as PCI and coronary artery bypass grafting (CABG), are used to restore blood flow to the heart in individuals with significant coronary artery disease. PCI involves inserting a catheter into the coronary artery and inflating a balloon to widen the narrowed artery, followed by the placement of a stent to keep the artery open. CABG involves grafting a healthy blood vessel from another part of the body to bypass the blocked coronary artery [20].
6. Future Directions
Despite significant advances in our understanding and treatment of metabolic-related CVD, several critical gaps remain. Future research should focus on:
- Elucidating the Mechanisms Underlying Sex Differences: Further research is needed to understand the mechanisms underlying sex differences in cardiovascular risk and outcomes in the context of metabolic disease. This research should focus on hormonal, genetic, and environmental factors that contribute to these differences.
- Developing Novel Therapeutic Targets: There is a need for novel therapeutic targets that specifically address the underlying mechanisms of cardiovascular remodeling in metabolic disease. These targets may include inhibitors of AGE-RAGE interaction, antioxidants, ER stress inhibitors, and inflammasome inhibitors.
- Improving Risk Stratification: Improved risk stratification tools are needed to identify individuals at high risk of cardiovascular events in the early stages of metabolic disease. These tools should incorporate novel biomarkers and imaging techniques to improve predictive accuracy.
- Personalized Medicine Approaches: Personalized medicine approaches, based on individual genetic and metabolic profiles, may be useful for tailoring prevention and treatment strategies for metabolic-related CVD. This may involve identifying individuals who are more likely to respond to specific therapies or who are at higher risk of developing specific cardiovascular complications.
- Longitudinal Studies: Long-term longitudinal studies are needed to assess the impact of different prevention and treatment strategies on cardiovascular outcomes in individuals with metabolic disease. These studies should focus on hard outcomes such as myocardial infarction, stroke, and death.
7. Conclusion
The interplay between metabolic dysregulation and cardiovascular remodeling is a complex and multifaceted process that contributes significantly to the global burden of CVD. A comprehensive understanding of the underlying molecular mechanisms, the impact of sex differences, and the development of targeted therapeutic interventions are crucial for preventing and managing metabolic-related CVD. Future research should focus on elucidating the mechanisms underlying sex differences, developing novel therapeutic targets, improving risk stratification, and implementing personalized medicine approaches. By addressing these critical gaps in our knowledge, we can improve the prevention and treatment of metabolic-related CVD and reduce the burden of this devastating disease.
References
[1] Grundy SM. Metabolic syndrome pandemic. Arterioscler Thromb Vasc Biol. 2008;28(6):1017-1019.
[2] Jia G, Hill MA, Sowers JR. Diabetic cardiomyopathy: an update of mechanisms contributing to this clinical entity. Circ Res. 2018;122(4):624-638.
[3] Yamagishi S, Matsui T. Advanced glycation end products, their receptors and diabetic complications. Curr Drug Targets. 2008;9(6):469-482.
[4] Schmidt AM, Yan SD, Stern R, et al. The dark side of glucose. Nat Med. 1996;2(9):951-952.
[5] Brownlee M. Biochemistry and molecular cell biology of diabetic complications. Nature. 2001;414(6865):813-820.
[6] Madamanchi NR, Runge MS. Redox signaling in cardiovascular disease: basic mechanisms and clinical applications. Arterioscler Thromb Vasc Biol. 2007;27(3):480-488.
[7] Ozcan U, Tabas I. Calcium, ER stress and lipid metabolism. J Clin Invest. 2012;122(10):3348-3356.
[8] Lopaschuk GD, Ussher JR, Folmes CDL, Jaswal JS, Stanley WC. Myocardial fatty acid metabolism in health and disease. Physiol Rev. 2010;90(1):207-269.
[9] Unger RH, Scherer PE. Gluttony, sloth and the metabolic syndrome: a roadmap to lipotoxicity. Trends Endocrinol Metab. 2010;21(9):345-352.
[10] Libby P. Inflammation in atherosclerosis. Nature. 2002;420(6917):868-874.
[11] Maas AH, Appelman YE. Sex differences in coronary heart disease. Arch Med Sci. 2010;6(5):817-826.
[12] Carr MC. The emergence of the metabolic syndrome with menopause. J Clin Endocrinol Metab. 2003;88(6):2404-2411.
[13] Lam CSP, Arnott C, Beale AL, et al. Sex differences in heart failure. Eur Heart J. 2019;40(47):3900-3908c.
[14] Marwick TH. Measurement of strain and strain rate by echocardiography: ready for prime time?. J Am Coll Cardiol. 2006;47(7):1313-1327.
[15] D’Agostino RB Sr, Vasan RS, Pencina MJ, et al. General cardiovascular risk profile for use in primary care: the Framingham Heart Study. Circulation. 2008;117(6):743-753.
[16] Knowler WC, Barrett-Connor E, Fowler SE, et al. Reduction in the incidence of type 2 diabetes with lifestyle intervention or metformin. N Engl J Med. 2002;346(6):393-403.
[17] Zelniker TA, Wiviott SD, Raz I, et al. SGLT2 inhibitors for primary and secondary prevention of cardiovascular and renal outcomes in type 2 diabetes: a meta-analysis of cardiovascular outcome trials. Lancet. 2019;393(10166):31-39.
[18] Grundy SM, Stone NJ, Bailey AL, et al. 2018 AHA/ACC/AACVPR/AAPA/ABC/ACPM/ADA/AGS/PCNA Guideline on the Management of Blood Cholesterol: A Report of the American College of Cardiology/American Heart Association Task Force on Clinical Practice Guidelines. Circulation. 2019;139(25):e1082-e1143.
[19] Lewis EJ, Hunsicker LG, Clarke WR, et al. Renoprotective effect of the angiotensin-receptor blocker irbesartan in patients with nephropathy due to type 2 diabetes. N Engl J Med. 2001;345(12):851-860.
[20] Yusuf S, Zucker D, Peduzzi P, et al. Effect of coronary artery bypass graft surgery on survival: overview of 10-year results from randomised trials by the Coronary Artery Bypass Graft Surgery Trialists Collaboration. Lancet. 1994;344(8922):563-570.
This review highlights the importance of understanding sex differences in metabolic-related CVD. Could future research explore how personalized lifestyle interventions, tailored to individual hormonal and metabolic profiles, might improve cardiovascular outcomes in women with T2DM?