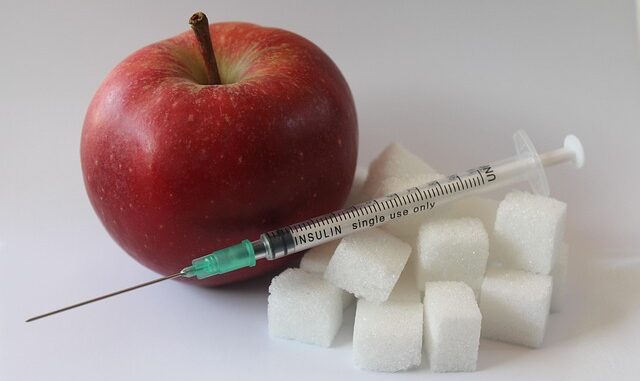
Abstract
Poor glucose control in individuals with Type 1 Diabetes (T1D) remains a significant clinical challenge, directly linked to increased morbidity and mortality due to micro- and macrovascular complications. This research report provides a comprehensive overview of glucose control in T1D, moving beyond conventional monitoring and management strategies. We delve into the intricate physiological mechanisms governing glucose homeostasis, explore cutting-edge technological innovations like advanced Continuous Glucose Monitoring (CGM) systems and closed-loop insulin delivery, and analyze the latest therapeutic strategies, including adjunct pharmacological interventions and emerging immunotherapies. Furthermore, we examine the psychosocial factors impacting adherence to treatment regimens and discuss strategies for personalized diabetes management, including the role of precision medicine and behavioral economics. Finally, we address the future directions of T1D research, focusing on preventative strategies and ultimately, a cure. The goal of this review is to provide experts in the field with an updated understanding of the complexities involved in achieving optimal glucose control and to stimulate further research towards improved outcomes for individuals with T1D.
Many thanks to our sponsor Esdebe who helped us prepare this research report.
1. Introduction
Type 1 Diabetes (T1D) is an autoimmune disease characterized by the destruction of insulin-producing beta cells in the pancreas, resulting in absolute insulin deficiency. This deficiency leads to hyperglycemia and, if left unmanaged, a cascade of metabolic disturbances culminating in long-term microvascular (retinopathy, nephropathy, neuropathy) and macrovascular (cardiovascular disease, stroke) complications [1]. Despite significant advancements in diabetes management, achieving optimal glycemic control remains a considerable challenge for many individuals with T1D [2].
The cornerstone of T1D management is exogenous insulin replacement therapy, traditionally delivered via multiple daily injections (MDI) or continuous subcutaneous insulin infusion (CSII) using insulin pumps. Technological advances such as Continuous Glucose Monitoring (CGM) and sensor-augmented pump therapy have revolutionized diabetes care, enabling more frequent and accurate glucose monitoring, as well as automated insulin delivery systems [3]. However, even with these innovations, many individuals with T1D still struggle to maintain target glucose levels, leading to fluctuations between hyperglycemia and hypoglycemia, both of which contribute to adverse health outcomes [4].
This report aims to provide a comprehensive overview of glucose control in T1D, encompassing not only conventional monitoring and management strategies but also delving into the underlying physiological mechanisms, emerging technologies, and novel therapeutic approaches. The discussion extends to the psychological and behavioral aspects of diabetes management, and concludes with an outlook on future research directions in T1D.
Many thanks to our sponsor Esdebe who helped us prepare this research report.
2. Physiological Mechanisms of Glucose Homeostasis in T1D
Understanding the intricate physiological mechanisms governing glucose homeostasis is crucial for developing effective strategies for T1D management. In healthy individuals, blood glucose levels are tightly regulated by the interplay of insulin and glucagon, secreted by the pancreatic beta and alpha cells, respectively [5]. Insulin facilitates glucose uptake by peripheral tissues (muscle, adipose tissue) and suppresses hepatic glucose production, while glucagon stimulates hepatic glucose production and glycogenolysis to raise blood glucose levels.
In T1D, the absence of insulin disrupts this delicate balance, leading to uncontrolled hyperglycemia. The liver, no longer under the inhibitory influence of insulin, continues to produce glucose, further exacerbating hyperglycemia [6]. Moreover, peripheral tissues are unable to effectively utilize glucose, resulting in cellular energy deficit and increased lipolysis, leading to elevated levels of free fatty acids and ketone body production [7].
Furthermore, beyond insulin and glucagon, other hormones, such as amylin, cortisol, epinephrine, and growth hormone, also play a role in glucose regulation [8]. Amylin, co-secreted with insulin from beta cells, slows gastric emptying, suppresses glucagon secretion, and promotes satiety [9]. In T1D, the absence of amylin contributes to postprandial hyperglycemia. Cortisol, epinephrine, and growth hormone, known as counter-regulatory hormones, are released in response to hypoglycemia, stimulating hepatic glucose production and inhibiting insulin secretion [10]. Understanding the complex interplay of these hormones is critical for optimizing insulin therapy and preventing hypoglycemia, a major limiting factor in achieving tight glycemic control.
Finally, research highlights the role of the gut microbiome in influencing glucose metabolism and insulin sensitivity [11]. Alterations in the gut microbiota composition have been linked to insulin resistance and impaired glucose tolerance. While research is ongoing, modulating the gut microbiome through dietary interventions or fecal microbiota transplantation may hold promise for improving glucose control in individuals with T1D.
Many thanks to our sponsor Esdebe who helped us prepare this research report.
3. Advancements in Glucose Monitoring Technologies
Accurate and frequent glucose monitoring is paramount for effective T1D management. Traditional methods of self-monitoring blood glucose (SMBG) using fingerstick testing provide only snapshot readings at specific time points, offering limited insight into glucose trends and variability [12]. Continuous Glucose Monitoring (CGM) has revolutionized glucose monitoring by providing real-time, continuous glucose readings every few minutes, allowing individuals to track glucose trends, identify patterns, and make informed decisions about insulin dosing and lifestyle adjustments [13].
3.1 Continuous Glucose Monitoring (CGM) Systems
CGM systems consist of a small sensor inserted subcutaneously that measures glucose levels in the interstitial fluid. The sensor transmits glucose data to a receiver or smartphone, displaying real-time glucose readings, trends, and alerts for high and low glucose levels. First-generation CGMs required frequent calibrations with fingerstick blood glucose measurements, but newer-generation CGMs, such as Dexcom G7 and Abbott FreeStyle Libre 3, are factory-calibrated and require minimal or no fingerstick calibrations [14, 15].
Advanced CGM features include predictive alerts, which warn users of impending hyperglycemia or hypoglycemia, allowing them to take proactive measures to prevent these events. Furthermore, data sharing capabilities allow individuals to share their glucose data with healthcare providers and caregivers, facilitating remote monitoring and personalized treatment adjustments [16].
3.2 Advanced CGM and Closed-Loop Systems
Closed-loop systems, also known as artificial pancreas systems, integrate CGM data with an insulin pump to automatically adjust insulin delivery based on real-time glucose levels [17]. These systems utilize sophisticated algorithms to predict glucose trends and adjust insulin delivery accordingly, minimizing the need for manual insulin adjustments. Hybrid closed-loop systems require user input for mealtime boluses, while fully automated closed-loop systems aim to automate all aspects of insulin delivery.
Recent advancements in closed-loop technology have led to the development of more sophisticated algorithms that can adapt to individual insulin sensitivity and activity levels. Dual-hormone closed-loop systems, which deliver both insulin and glucagon, are being developed to further improve glucose control and prevent hypoglycemia [18]. While still in the early stages of development, these systems hold tremendous promise for achieving near-normal glucose levels and reducing the burden of diabetes management.
3.3 Emerging Glucose Monitoring Technologies
Research is underway to develop non-invasive glucose monitoring technologies, such as optical sensors, radiofrequency sensors, and microneedle-based sensors [19]. These technologies aim to eliminate the need for fingersticks or subcutaneous sensor insertion, providing a more convenient and painless method of glucose monitoring. While these technologies are still in the early stages of development, they hold great potential for improving the lives of individuals with T1D.
Many thanks to our sponsor Esdebe who helped us prepare this research report.
4. Therapeutic Strategies for Optimal Glucose Control
Achieving optimal glucose control in T1D requires a multi-faceted approach, encompassing insulin therapy, dietary modifications, exercise, and psychosocial support.
4.1 Insulin Therapy
Insulin therapy remains the cornerstone of T1D management. Various insulin formulations are available, including rapid-acting, short-acting, intermediate-acting, and long-acting insulins. Rapid-acting insulin analogs (e.g., lispro, aspart, glulisine) are typically used for mealtime boluses, while long-acting insulin analogs (e.g., glargine, detemir, degludec) are used to provide basal insulin coverage [20].
Insulin delivery methods include multiple daily injections (MDI) and continuous subcutaneous insulin infusion (CSII) using insulin pumps. MDI involves injecting insulin several times a day, typically before meals and at bedtime. CSII involves delivering insulin continuously through a small catheter inserted under the skin, providing a more flexible and precise method of insulin delivery [21].
Recent advancements in insulin therapy include the development of ultra-rapid-acting insulin analogs and inhaled insulin, which provide faster onset of action and may improve postprandial glucose control [22]. Furthermore, smart insulins are being developed that can automatically adjust insulin release based on glucose levels, providing a more physiological and personalized approach to insulin therapy [23].
4.2 Dietary Modifications
Dietary modifications play a crucial role in managing blood glucose levels in T1D. Carbohydrate counting is a widely used method for matching insulin doses to carbohydrate intake [24]. Individuals with T1D are typically advised to consume a balanced diet that is low in processed foods, sugary drinks, and saturated fats. The timing and composition of meals can significantly impact postprandial glucose levels, necessitating careful planning and monitoring.
Emerging dietary strategies include low-carbohydrate diets and ketogenic diets, which have shown promise in improving glucose control and reducing insulin requirements in some individuals with T1D [25]. However, these diets require careful monitoring and management to prevent complications such as ketoacidosis and nutrient deficiencies.
4.3 Exercise
Regular physical activity is beneficial for overall health and plays a vital role in managing blood glucose levels in T1D. Exercise increases insulin sensitivity, improves glucose utilization, and reduces the risk of cardiovascular disease [26]. However, exercise can also lead to hypoglycemia, particularly in individuals taking insulin. Careful planning and monitoring of blood glucose levels are essential before, during, and after exercise.
Different types of exercise have different effects on blood glucose levels. Aerobic exercise (e.g., running, swimming, cycling) tends to lower blood glucose levels, while resistance exercise (e.g., weightlifting) can increase blood glucose levels [27]. Individuals with T1D should work with their healthcare providers to develop an individualized exercise plan that takes into account their insulin regimen, dietary habits, and exercise preferences.
4.4 Adjunct Pharmacological Interventions
In addition to insulin therapy, other medications may be used as adjuncts to improve glucose control in T1D. Metformin, a commonly used medication for type 2 diabetes, can improve insulin sensitivity and reduce hepatic glucose production [28]. However, metformin is not FDA-approved for use in T1D and may not be effective in all individuals. SGLT2 inhibitors, which increase glucose excretion in the urine, have also been studied as adjuncts to insulin therapy in T1D [29]. While these medications can improve glucose control and reduce body weight, they are associated with an increased risk of diabetic ketoacidosis and genital infections.
4.5 Emerging Immunotherapies
As T1D is an autoimmune disease, immunotherapies that target the underlying immune dysregulation are being investigated as potential treatments. Clinical trials have evaluated various immunomodulatory agents, including anti-CD3 antibodies, interleukin-2, and glutamic acid decarboxylase (GAD) vaccines [30]. While some of these therapies have shown promise in preserving residual beta cell function in newly diagnosed T1D, none have yet been approved for widespread use. Further research is needed to identify effective and safe immunotherapies that can prevent or delay the progression of T1D.
Many thanks to our sponsor Esdebe who helped us prepare this research report.
5. Psychological and Behavioral Aspects of Diabetes Management
Effective T1D management requires not only medical and technological interventions but also addressing the psychological and behavioral aspects of the disease. Living with T1D can be emotionally and psychologically challenging, requiring constant monitoring, meticulous planning, and adherence to complex treatment regimens [31].
5.1 Psychological Barriers to Effective Self-Management
Many individuals with T1D experience psychological distress, including anxiety, depression, and diabetes-related distress. These psychological factors can significantly impact adherence to treatment regimens, leading to poor glucose control and increased risk of complications [32]. Fear of hypoglycemia is a common barrier to achieving tight glycemic control, as individuals may intentionally maintain higher blood glucose levels to avoid hypoglycemic episodes.
Other psychological barriers include diabetes burnout, a state of emotional exhaustion and disengagement from diabetes management, and negative attitudes towards insulin therapy. Addressing these psychological barriers requires a comprehensive approach that includes education, counseling, and support groups.
5.2 Strategies for Improving Adherence and Self-Efficacy
Various strategies can be employed to improve adherence to treatment regimens and enhance self-efficacy in individuals with T1D. These include motivational interviewing, a patient-centered counseling approach that helps individuals identify and address their ambivalence towards behavior change [33]. Cognitive behavioral therapy (CBT) can help individuals identify and modify negative thoughts and behaviors that contribute to poor diabetes management.
Peer support groups provide a valuable platform for individuals with T1D to share experiences, learn from each other, and receive emotional support. Technology-based interventions, such as mobile apps and online support groups, can also enhance self-management skills and improve adherence to treatment regimens [34].
5.3 The Role of Behavioral Economics
Behavioral economics offers insights into how people make decisions and can be used to design interventions that promote healthier behaviors in individuals with T1D. Nudges, small changes in the environment or presentation of information, can influence choices without restricting freedom of choice [35]. For example, providing visual reminders to check blood glucose levels or offering incentives for achieving target glucose levels can promote adherence to treatment regimens.
Many thanks to our sponsor Esdebe who helped us prepare this research report.
6. Personalized Diabetes Management and Precision Medicine
Recognizing the heterogeneity of T1D and individual differences in response to treatment, personalized diabetes management is becoming increasingly important. Precision medicine, which tailors treatment to individual characteristics based on genetic, environmental, and lifestyle factors, holds promise for improving outcomes in T1D [36].
6.1 Genetic Factors
Genetic factors play a significant role in the development and progression of T1D. Human leukocyte antigen (HLA) genes are the strongest genetic risk factors for T1D, but other genes involved in immune regulation and beta cell function also contribute to disease susceptibility [37]. Genetic testing can identify individuals at high risk for developing T1D and may guide preventative strategies. Furthermore, genetic variations may influence individual responses to insulin therapy and other treatments, allowing for more personalized treatment decisions.
6.2 Environmental Factors
Environmental factors, such as viral infections, dietary exposures, and gut microbiota composition, are also implicated in the pathogenesis of T1D [38]. Identifying and modifying these environmental factors may help prevent or delay the onset of T1D. For example, dietary interventions to modulate the gut microbiota may improve insulin sensitivity and reduce the risk of autoimmune destruction of beta cells.
6.3 Lifestyle Factors
Lifestyle factors, such as diet, exercise, and stress management, significantly impact glucose control and overall health in individuals with T1D. Personalized lifestyle recommendations, based on individual preferences, cultural backgrounds, and socioeconomic circumstances, are essential for promoting adherence and achieving optimal outcomes. Wearable sensors and mobile apps can be used to track physical activity, dietary intake, and sleep patterns, providing valuable data for personalized lifestyle coaching.
Many thanks to our sponsor Esdebe who helped us prepare this research report.
7. Long-Term Complications and Prevention Strategies
Poor glucose control in T1D leads to a multitude of long-term complications affecting virtually every organ system. These complications are primarily driven by chronic hyperglycemia and associated metabolic disturbances, leading to both microvascular and macrovascular damage.
7.1 Microvascular Complications
Microvascular complications, including retinopathy, nephropathy, and neuropathy, are hallmark features of chronic hyperglycemia. Retinopathy, affecting the small blood vessels of the retina, can lead to vision loss and blindness [39]. Nephropathy, affecting the kidneys, can progress to end-stage renal disease requiring dialysis or kidney transplantation [40]. Neuropathy, affecting the nerves, can cause pain, numbness, and tingling in the extremities, as well as autonomic dysfunction affecting cardiovascular, gastrointestinal, and genitourinary systems [41].
7.2 Macrovascular Complications
Macrovascular complications, including cardiovascular disease, stroke, and peripheral artery disease, are major causes of morbidity and mortality in individuals with T1D [42]. Chronic hyperglycemia, along with other risk factors such as dyslipidemia and hypertension, accelerates the development of atherosclerosis, leading to these cardiovascular complications.
7.3 Prevention Strategies
Aggressive glucose control, along with management of other risk factors such as blood pressure and cholesterol levels, is essential for preventing or delaying the onset and progression of long-term complications. Regular screening for microvascular complications, including annual eye exams, urine albumin testing, and foot exams, is crucial for early detection and intervention. Lifestyle modifications, such as smoking cessation, weight management, and regular exercise, can also reduce the risk of complications.
7.4 Emerging Prevention Strategies
Emerging prevention strategies include immunotherapies to preserve residual beta cell function in newly diagnosed T1D and interventions to prevent the development of T1D in individuals at high risk. Clinical trials are evaluating various immunomodulatory agents, as discussed earlier, to prevent or delay the progression of T1D. Furthermore, studies are investigating the potential role of dietary interventions and gut microbiota modulation in preventing the development of T1D in genetically predisposed individuals [43].
Many thanks to our sponsor Esdebe who helped us prepare this research report.
8. Future Directions and Conclusion
The field of T1D research is rapidly evolving, with significant advancements in glucose monitoring technologies, therapeutic strategies, and understanding of the underlying pathophysiology of the disease. Future research directions include the development of fully automated closed-loop systems, smart insulins, and non-invasive glucose monitoring technologies. Immunotherapies that can prevent or reverse the autoimmune destruction of beta cells remain a major goal of T1D research.
Furthermore, research is needed to better understand the role of genetic and environmental factors in the development and progression of T1D. Precision medicine approaches that tailor treatment to individual characteristics hold promise for improving outcomes in T1D. Finally, addressing the psychological and behavioral aspects of diabetes management is essential for promoting adherence and improving quality of life for individuals with T1D.
In conclusion, while significant progress has been made in the management of T1D, achieving optimal glucose control remains a considerable challenge. A multi-faceted approach that encompasses technological innovations, personalized therapeutic strategies, and psychosocial support is essential for improving outcomes and preventing long-term complications. Continued research efforts are needed to develop novel therapies and ultimately, find a cure for T1D.
Many thanks to our sponsor Esdebe who helped us prepare this research report.
References
[1] American Diabetes Association. (2023). Standards of medical care in diabetes—2023. Diabetes Care, 46(Supplement 1), S1-S291.
[2] Beck, R. W., Riddlesworth, T. D., Ruedy, K. J., Ahmann, A. J., Bergenstal, R. M., Haller, M. J., … & Tamborlane, W. V. (2017). Continuous glucose monitoring versus usual care in adults with type 1 diabetes: a randomized trial. Annals of Internal Medicine, 167(6), 365-374.
[3] Bergenstal, R. M., Beck, R. W., Close, K. L., Grunberger, G., Sacks, D. B., & Ratner, R. E. (2008). Glucose management indicator (GMI): A new term for estimating A1C from continuous glucose monitoring. Diabetes Care, 41(11), 2275-2280.
[4] Cryer, P. E. (2002). Hypoglycemia is the limiting factor in the glycemic management of type 1 and type 2 diabetes. Diabetes, 51(12), 3552-3561.
[5] DeFronzo, R. A. (1988). Lilly lecture 1987. The triumvirate: beta-cell, muscle, liver. A collusion responsible for NIDDM. Diabetes, 37(6), 667-687.
[6] Gerich, J. E. (1988). Glucose counterregulation and type 1 diabetes. Diabetes, 37(12), 1608-1617.
[7] Unger, R. H. (1991). Lipotoxicity in the pathogenesis of obesity-dependent NIDDM. Genetic and clinical implications. Diabetes, 40(Supplement 2), 49-53.
[8] Briscoe, V. J., Davis, S. N. (2006). Oral glucose tolerance test: physiological model measurement to assess glucose sensitivity. Diabetes spectrum, 19(4), 223.
[9] Young, A. A., Gedulin, B. R., Rink, T. J. (1992). Dose-related reduction of food intake and body weight by amylin in ob/ob mice. American Journal of Physiology-Regulatory, Integrative and Comparative Physiology, 263(5), R903-R907.
[10] Cryer, P. E. (1993). Glucose counterregulation in man. Diabetes, 42(8), 1191-1201.
[11] Gurung, M., Li, Z., You, H., Rodrigues, R., Jump, D. B., Morgun, A., & Shulzhenko, N. (2020). Role of gut microbiota in type 2 diabetes pathophysiology. EMBO molecular medicine, 12(5), e10895.
[12] Clarke, W. L., & Foster, J. R. (2012). A history of blood glucose meters and their role in self-monitoring of diabetes. British Journal of Biomedical Science, 69(2), 83-93.
[13] Heinemann, L., & Freckmann, G. (2015). Continuous glucose monitoring in type 1 diabetes: current status and future directions. Journal of Diabetes Science and Technology, 9(4), 702-714.
[14] Dexcom. (n.d.). Dexcom G7 CGM System. Retrieved from Dexcom Website
[15] Abbott. (n.d.). FreeStyle Libre 3. Retrieved from Abbott Website
[16] Hirsch, I. B., Grunberger, G., Bode, B. W., Garg, S., & Weinstock, R. S. (2021). Continuous glucose monitoring: the future is here. Diabetes Technology & Therapeutics, 23(S1), S2-S10.
[17] Bekiari, E., Kitsios, K., Thabit, H., Tauschmann, M., Athanasiadou, E., Karagiannis, T., … & Haidich, A. B. (2018). Artificial pancreas treatment for outpatients with type 1 diabetes: systematic review and meta-analysis. BMJ, 361, k1310.
[18] Russell, S. J., El-Khatib, F. H., Sinha, M., Magyar, K. L., Gale, E. A., & Nathan, D. M. (2016). Outpatient glycemic control with a bionic pancreas in type 1 diabetes. New England Journal of Medicine, 374(11), 1074-1075.
[19] Bandodkar, A. J., Wang, J. (2014). Non-invasive wearable electrochemical sensors: current progress and future potential. Trends in biotechnology, 32(7), 363-371.
[20] Pickup, J. C. (2012). Insulin preparations and their use. The Lancet, 379(9833), 2279-2292.
[21] Pickup, J. C., & Keen, H. (1982). Continuous subcutaneous insulin infusion: an overview. Diabetes Care, 5(3), 190-197.
[22] Cahn, A., Cefalu, W. T., Deanfield, J. E., Dinneen, S. F., Halvorsen, T., Holst, J. J., … & Schnell, O. (2021). Glycaemic variability: assessment, clinical significance and recommendations for use. Diabetes/metabolism research and reviews, 37(1), e3361.
[23] Klok, G. G., & Hood, L. (2017). Systems medicine: a new approach to diabetes care. Diabetes, 66(1), 1-7.
[24] Wheeler, M. L., Franz, M. J., Bantle, J. P., Finegood, D. T., Nerup, J., Peer, N., … & International Diabetes Federation. (2004). Macronutrient, fiber, and nonnutritive sweetener intake in diabetes: a position statement of the American Diabetes Association. Diabetes Care, 27(1), 242-245.
[25] Nielsen, J. V., Jonsson, E., & Ivarsson, A. (2005). A low carbohydrate diet in type 1 diabetes: a single-center, randomized, clinical trial. Diabetes, Obesity and Metabolism, 7(6), 683-690.
[26] Colberg, S. R., Sigal, R. J., Yardley, J. E., Riddell, M. C., Dunstan, D. W., Dempsey, P. C., … & Horton, E. S. (2016). Physical activity/exercise and type 2 diabetes: a consensus statement from the American Diabetes Association. Diabetes Care, 39(11), 2065-2079.
[27] Riddell, M. C., Gallen, I. W., Faeh, D., Rose, S., Sauriol, L., Guinhouya, B. C., … & Yardley, J. E. (2017). Exercise management in type 1 diabetes: a consensus statement. The Lancet Diabetes & Endocrinology, 5(5), 377-390.
[28] Holman, R. R., Coleman, R. L., Shine, B. S., Farmer, A. J. (2017). Effect of metformin on kidney function in type 2 diabetes. New England Journal of Medicine, 376(15), 1418-1428.
[29] Dandona, P., Mathieu, C., Hansen, T. K., Hansen, A. S., Schultz, N., & Thorsteinsson, B. (2015). Efficacy and safety of dapagliflozin in patients with inadequately controlled type 1 diabetes (DEPICT-1): 24 week results from a randomised controlled trial. The Lancet Diabetes & Endocrinology, 3(11), 864-873.
[30] Haller, M. J., Gitelman, S. E., Gottlieb, P. A., Michels, A. W., Rosenthal, S. M., Shuster, J. J., … & Type 1 Diabetes TrialNet Study Group. (2019). Anti-thymocyte globulin/alemtuzumab combination therapy in adults with recent-onset type 1 diabetes: 2 year results. Diabetes, 68(1), 187-194.
[31] Fisher, L., Mullan, J., Arean, P. A., Glasgow, R. E., & Hessler, D. (2007). Diabetes distress, depression, and adherence as distinct but related aspects of self-management in adults with type 2 diabetes. Diabetes Care, 30(12), 3088-3093.
[32] Young-Hyman, D., de Groot, M., Hill-Briggs, F., Gonzalez, J. S., Hood, K., Peyrot, M. (2016). Living well with diabetes: a position statement of the American Diabetes Association. Diabetes Care, 39(3), 528-535.
[33] Miller, W. R., & Rollnick, S. (2012). Motivational interviewing: Helping people change. Guilford press.
[34] Quinn, C. C., Shardell, M. D., Terrin, M. L., Barrington, C. L., Gruber-Baldini, A. L., & Jabs, E. W. (2011). Mobile phone text messaging and personal digital assistants for diabetes self-management: a systematic review. Journal of the American Medical Informatics Association, 18(6), 748-754.
[35] Thaler, R. H., & Sunstein, C. R. (2008). Nudge: Improving decisions about health, wealth, and happiness. Yale University Press.
[36] Hood, L., & Friend, S. H. (2011). Predictive, personalized, and preventive medicine. Science Translational Medicine, 3(73), 73ps12.
[37] Todd, J. A. (2010). Etiology of type 1 diabetes. Immunity, 32(4), 457-467.
[38] Knip, M., Simell, O., Ilonen, J., Hyöty, H., Veijola, R., Reunanen, A., … & Ziegler, A. G. (2005). Environmental triggers and determinants of type 1 diabetes. Diabetes, 54(Supplement 2), S125-S136.
[39] Fong, D. S., Aiello, L. P., Gardner, T. W., King, G. L., Blankenship, G., Cavallerano, J. D., … & Ferris, F. L. (2004). Retinopathy in diabetes. Diabetes Care, 27(Supplement 1), S84-S87.
[40] Mogensen, C. E., Christensen, C. K., & Vittinghus, E. (1983). The stages in diabetic nephropathy: with emphasis on the stage of microalbuminuria. Diabetes, 32(Supplement 2), 64-78.
[41] Vinik, A. I., Erbas, T., Park, T. S., Nolan, R., Pittenger, G. L. (2003). Diabetic autonomic neuropathy. Endocrine reviews, 24(3), 293-344.
[42] Juutilainen, A., Lehto, S., Rönnemaa, T., Pyörälä, K., & Laakso, M. (1995). Type 1 diabetes as a risk factor for coronary heart disease. Diabetes Care, 18(10), 1304-1309.
[43] Giongo, A., Gano, K. A., Crabb, D. B., Romano, K. A., Lacy, D. B., Kelly, C. J., … & Lynch, K. S. (2011). Toward defining the autoimmune microbiome for type 1 diabetes. The ISME journal, 5(11), 1623-1631.
So, the gut microbiome can affect glucose control, you say? Does that mean if I feed my sourdough starter REALLY well, I can claim it as a tax deduction for medical expenses? Asking for, uh, science!
That’s a *very* interesting question! While I can’t offer tax advice, you’ve hit on a fascinating point. Further research explores how specific gut bacteria influence glucose metabolism. So while feeding your sourdough may not be a deduction, exploring prebiotic and probiotic rich foods might be something to discuss with your doctor. Thanks for the thought-provoking comment!
Editor: MedTechNews.Uk
Thank you to our Sponsor Esdebe
This report highlights the crucial role of technology, especially closed-loop systems, in managing T1D. What are your thoughts on the accessibility and affordability of these advanced technologies for individuals across different socioeconomic backgrounds, and how can we bridge this gap?
That’s a great point about the accessibility and affordability of closed-loop systems! It’s definitely a barrier for many. I think exploring more flexible financing models and advocating for increased insurance coverage are key steps to ensuring these technologies are available to everyone who needs them. Perhaps subsidies could also be explored! What are your thoughts on the effectiveness of government funding in these areas?
Editor: MedTechNews.Uk
Thank you to our Sponsor Esdebe