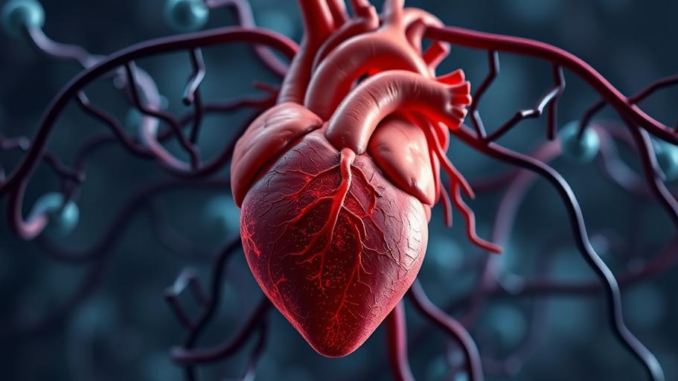
Abstract
Cardiomyopathy (CMP) encompasses a heterogeneous group of myocardial disorders characterized by structural and functional abnormalities of the heart muscle, frequently leading to heart failure, arrhythmias, and sudden cardiac death. While the etiology of CMP can be acquired, a significant proportion is genetically determined. This review provides a comprehensive overview of CMP, delving into the diverse forms, intricate genotype-phenotype correlations, the impact of local genetic factors, and recent breakthroughs in diagnostic and therapeutic strategies. We explore the underlying genetic mechanisms driving various CMP subtypes, emphasizing the critical role of genetic testing in diagnosis, risk stratification, and personalized management. Furthermore, we examine the influence of regional genetic variations on disease presentation and progression, highlighting the importance of considering ancestry and environmental factors. Finally, we discuss emerging therapies, including gene editing and targeted pharmacological interventions, that hold promise for improving outcomes in patients with CMP. This review is aimed at providing an in-depth understanding of CMP for experts in the field, paving the way for future research and improved clinical care.
Many thanks to our sponsor Esdebe who helped us prepare this research report.
1. Introduction
Cardiomyopathies represent a significant burden on global health, contributing substantially to morbidity and mortality. These diseases, defined by structural and functional abnormalities of the ventricular myocardium not caused by coronary artery disease, hypertension, valvular disease, or congenital heart disease (though these may be present as secondary findings), encompass a diverse range of phenotypes and etiologies [1]. The World Heart Federation classifies cardiomyopathy based on morpho-functional phenotypes, including dilated cardiomyopathy (DCM), hypertrophic cardiomyopathy (HCM), restrictive cardiomyopathy (RCM), arrhythmogenic cardiomyopathy (ACM), and left ventricular non-compaction cardiomyopathy (LVNC) [2]. Unclassified cardiomyopathies may also exist.
While acquired causes such as viral infections, alcohol abuse, and chemotherapeutic agents can induce cardiomyopathy, a substantial proportion, estimated to be up to 50%, are genetically determined [3]. These genetic cardiomyopathies are primarily inherited in an autosomal dominant manner, although autosomal recessive, X-linked, and mitochondrial inheritance patterns have also been observed. The identification of disease-causing genes has revolutionized our understanding of the molecular mechanisms underlying CMP and has enabled the development of genetic testing for diagnosis, risk stratification, and family screening [4].
This review aims to provide a comprehensive and updated overview of CMP, focusing on the genetic underpinnings, genotype-phenotype correlations, the influence of local genetic factors, and recent advances in therapeutic approaches. We will explore the diverse forms of CMP, the underlying genetic mechanisms driving disease pathogenesis, and the significance of genetic testing in diagnosis and risk assessment. Furthermore, we will examine the impact of regional genetic variations on disease presentation and progression, highlighting the importance of considering ancestry and environmental factors. Finally, we will discuss emerging therapies, including gene editing and targeted pharmacological interventions, that hold promise for improving outcomes in patients with CMP.
Many thanks to our sponsor Esdebe who helped us prepare this research report.
2. Classification and Phenotypes of Cardiomyopathy
Cardiomyopathies are broadly classified based on their morphological and functional characteristics. The most prevalent types include dilated cardiomyopathy (DCM), hypertrophic cardiomyopathy (HCM), restrictive cardiomyopathy (RCM), arrhythmogenic cardiomyopathy (ACM), and left ventricular non-compaction cardiomyopathy (LVNC) [2].
2.1 Dilated Cardiomyopathy (DCM)
DCM is characterized by left ventricular or biventricular dilation and impaired systolic function. Patients with DCM typically present with heart failure symptoms such as dyspnea, fatigue, and edema. DCM can be caused by a variety of factors, including genetic mutations, viral infections, alcohol abuse, and chemotherapy. Genetic causes account for approximately 20-50% of DCM cases. Common genes implicated in DCM include TTN, LMNA, MYH7, and DES [5]. Truncating variants in TTN, encoding the giant protein titin, are the most common genetic cause of DCM, accounting for approximately 25% of familial DCM cases. LMNA encodes lamin A/C, a nuclear envelope protein. Mutations in LMNA are associated with a more severe form of DCM, often accompanied by conduction system disease, arrhythmias, and increased risk of sudden cardiac death [6]. Variants in genes encoding sarcomeric proteins, such as MYH7 (beta-myosin heavy chain), are less frequent causes of DCM but can be associated with both DCM and HCM phenotypes. Desmin (DES) encodes an intermediate filament protein that plays a crucial role in maintaining the structural integrity of muscle cells. Mutations in DES can cause DCM, as well as other muscle disorders [7].
2.2 Hypertrophic Cardiomyopathy (HCM)
HCM is defined by unexplained left ventricular hypertrophy, typically involving the interventricular septum. HCM is primarily caused by mutations in genes encoding sarcomeric proteins. The most frequently mutated genes include MYH7, MYBPC3 (myosin-binding protein C), and TNNT2 (troponin T). HCM can be associated with a variety of clinical manifestations, including chest pain, dyspnea, palpitations, and sudden cardiac death. The phenotypic expression of HCM is highly variable, even among individuals with the same mutation. Some patients may be asymptomatic, while others may develop severe heart failure or sudden cardiac arrest. The diagnosis of HCM is typically made based on echocardiography, which demonstrates left ventricular hypertrophy in the absence of other causes, such as hypertension or aortic stenosis [8]. Genetic testing is recommended for individuals with HCM to confirm the diagnosis, identify at-risk family members, and guide management decisions.
2.3 Restrictive Cardiomyopathy (RCM)
RCM is characterized by impaired ventricular filling due to increased myocardial stiffness, with normal or near-normal systolic function. RCM is the least common type of cardiomyopathy. RCM can be caused by a variety of factors, including infiltrative diseases such as amyloidosis, sarcoidosis, and hemochromatosis, as well as genetic mutations. Mutations in genes encoding sarcomeric proteins, such as TNNI3 (troponin I) and MYH7, have been associated with RCM. The clinical presentation of RCM is similar to that of constrictive pericarditis, making it difficult to distinguish between the two conditions. Echocardiography, cardiac MRI, and endomyocardial biopsy can be used to differentiate RCM from constrictive pericarditis [9].
2.4 Arrhythmogenic Cardiomyopathy (ACM)
ACM is characterized by progressive replacement of the myocardium with fibrofatty tissue, primarily affecting the right ventricle. ACM is a major cause of sudden cardiac death, particularly in young athletes. ACM is primarily caused by mutations in genes encoding desmosomal proteins, which are essential for cell-cell adhesion. The most frequently mutated genes include PKP2 (plakophilin-2), DSP (desmoplakin), DSG2 (desmoglein-2), DSC2 (desmocollin-2), and JUP (junction plakoglobin). Mutations in these genes disrupt the structure and function of desmosomes, leading to cardiomyocyte detachment, cell death, and fibrofatty replacement. The diagnosis of ACM is based on the revised Task Force Criteria, which include structural, functional, and histological abnormalities, as well as genetic testing [10].
2.5 Left Ventricular Non-Compaction Cardiomyopathy (LVNC)
LVNC is characterized by prominent trabeculations and deep intertrabecular recesses in the left ventricle. LVNC can be an isolated cardiomyopathy or can occur in association with other congenital heart defects or neuromuscular disorders. The genetic basis of LVNC is complex and heterogeneous, with mutations in genes encoding sarcomeric proteins, cytoskeletal proteins, and mitochondrial proteins implicated in disease pathogenesis. Genes like MYH7, MYBPC3, ACTC1 (actin, alpha cardiac muscle 1), and LMNA are associated with LVNC [11]. The diagnosis of LVNC is based on echocardiographic criteria, which include an increased ratio of non-compacted to compacted myocardium.
Many thanks to our sponsor Esdebe who helped us prepare this research report.
3. Genetic Mechanisms and Genotype-Phenotype Correlations
The genetic landscape of cardiomyopathy is complex, with a wide range of genes implicated in disease pathogenesis. The most commonly mutated genes encode sarcomeric proteins, cytoskeletal proteins, desmosomal proteins, and proteins involved in calcium handling. Mutations in these genes can disrupt cardiomyocyte structure, function, and electrical stability, leading to the development of cardiomyopathy [12].
3.1 Sarcomeric Protein Genes
Mutations in genes encoding sarcomeric proteins are a major cause of HCM and DCM. These proteins, including myosin heavy chain (MYH7), myosin-binding protein C (MYBPC3), troponin T (TNNT2), troponin I (TNNI3), and alpha-actin (ACTC1), are essential for muscle contraction. Mutations in these genes can lead to abnormalities in sarcomere structure and function, resulting in hypertrophy, dilation, or impaired contractility [13].
- MYH7: Mutations in MYH7 are associated with both HCM and DCM. Different mutations within the same gene can lead to different phenotypes, highlighting the complexity of genotype-phenotype correlations. For example, some MYH7 mutations are associated with a more severe form of HCM, while others are associated with a milder phenotype or even DCM. The location of the mutation within the protein also influences the phenotype. Mutations in the head region of myosin are more likely to cause DCM, while mutations in the rod region are more likely to cause HCM [14].
- MYBPC3: MYBPC3 mutations are one of the most common cause of HCM. MYBPC3 truncating variants, causing haploinsufficiency, are particularly prevalent in South Asian populations. Most MYBPC3 mutations are associated with a relatively mild phenotype and a lower risk of sudden cardiac death [15].
- TNNT2: Mutations in TNNT2 are associated with both HCM and RCM. Some TNNT2 mutations are associated with a high risk of sudden cardiac death, particularly in young individuals [16].
3.2 Cytoskeletal Protein Genes
Mutations in genes encoding cytoskeletal proteins, such as desmin (DES) and lamin A/C (LMNA), are associated with DCM and RCM. These proteins provide structural support to cardiomyocytes and maintain their shape and integrity. Mutations in these genes can lead to disruption of the cytoskeleton, resulting in cardiomyocyte damage and dysfunction [17].
- DES: DES mutations are associated with DCM, RCM, and skeletal muscle disorders. The phenotype associated with DES mutations can vary depending on the location and type of mutation. Some DES mutations are associated with a more severe form of DCM, while others are associated with a milder phenotype [7].
- LMNA: LMNA mutations are associated with a wide range of phenotypes, including DCM, conduction system disease, arrhythmias, and muscular dystrophy. LMNA mutations often result in a more aggressive DCM phenotype with a higher risk of sudden death [6].
3.3 Desmosomal Protein Genes
Mutations in genes encoding desmosomal proteins, such as plakophilin-2 (PKP2), desmoplakin (DSP), desmoglein-2 (DSG2), desmocollin-2 (DSC2), and junction plakoglobin (JUP), are a major cause of ACM. These proteins form the desmosomes, which are cell-cell junctions that provide mechanical support to cardiomyocytes. Mutations in these genes disrupt the structure and function of desmosomes, leading to cardiomyocyte detachment, cell death, and fibrofatty replacement [10].
3.4 Calcium Handling Genes
Mutations in genes encoding proteins involved in calcium handling, such as ryanodine receptor 2 (RYR2) and calsequestrin 2 (CASQ2), are associated with catecholaminergic polymorphic ventricular tachycardia (CPVT) and DCM. These proteins regulate intracellular calcium levels, which are essential for muscle contraction. Mutations in these genes can lead to abnormal calcium handling, resulting in arrhythmias and impaired contractility [18].
3.5 Genotype-Phenotype Correlations
While genetic testing has improved our understanding of the molecular basis of CMP, genotype-phenotype correlations are often complex and incomplete. The same mutation can lead to different phenotypes in different individuals, and different mutations in the same gene can lead to the same phenotype. Several factors contribute to this complexity, including genetic modifiers, environmental factors, and epigenetic modifications [19].
- Genetic Modifiers: Genetic modifiers are genes that influence the expression of other genes. These genes can modify the phenotype associated with a particular mutation, making it more or less severe. For example, a mutation in a modifier gene may compensate for the effect of a disease-causing mutation, resulting in a milder phenotype.
- Environmental Factors: Environmental factors, such as diet, exercise, and exposure to toxins, can also influence the phenotype associated with a particular mutation. For example, individuals with a genetic predisposition to HCM may develop a more severe phenotype if they engage in strenuous exercise.
- Epigenetic Modifications: Epigenetic modifications are changes in gene expression that do not involve changes in the DNA sequence. These modifications can be influenced by environmental factors and can affect the phenotype associated with a particular mutation. For example, DNA methylation and histone modification can alter gene expression and influence disease susceptibility.
Many thanks to our sponsor Esdebe who helped us prepare this research report.
4. Influence of Local Genetic Factors and Ancestry
The prevalence and genetic architecture of cardiomyopathy can vary significantly across different populations and geographic regions. These variations are influenced by local genetic factors, founder effects, and environmental exposures [20].
4.1 Founder Effects
Founder effects occur when a specific mutation is present at a higher frequency in a particular population due to the ancestry of the population. For example, specific mutations in MYBPC3 are highly prevalent in South Asian populations due to a founder effect. These mutations are associated with a relatively mild form of HCM and a lower risk of sudden cardiac death [15]. Similarly, certain LMNA mutations are more common in specific European populations, leading to a higher prevalence of LMNA-related DCM in these regions [6].
4.2 Genetic Admixture
Genetic admixture, the mixing of genes from different populations, can also influence the genetic architecture of cardiomyopathy. For example, African Americans have a higher prevalence of certain genetic variants associated with DCM, such as mutations in LMNA and TTN [21]. This is likely due to genetic admixture between African and European populations.
4.3 Environmental Exposures
Environmental exposures can also play a role in the pathogenesis of cardiomyopathy. For example, exposure to certain toxins, such as alcohol and chemotherapeutic agents, can increase the risk of developing DCM. Nutritional deficiencies, such as thiamine deficiency, can also contribute to the development of cardiomyopathy. Furthermore, infection by specific viruses such as Coxsackievirus can induce DCM, although the genetic background of the individual can influence the response to viral infection.
4.4 Implications for Genetic Testing and Risk Assessment
The influence of local genetic factors and ancestry has important implications for genetic testing and risk assessment. Genetic testing should be tailored to the specific population being tested, taking into account the prevalence of different mutations in that population. Risk assessment should also consider ancestry and environmental exposures, as these factors can influence the phenotype associated with a particular mutation. In summary, genetic testing and interpretation should not occur in a vacuum; understanding the patient’s ancestry is essential.
Many thanks to our sponsor Esdebe who helped us prepare this research report.
5. Breakthroughs in Research and Therapeutic Strategies
Recent advances in our understanding of the genetic and molecular mechanisms underlying cardiomyopathy have led to the development of new diagnostic and therapeutic strategies. These breakthroughs include improved genetic testing, targeted pharmacological interventions, and gene editing [22].
5.1 Advanced Genetic Testing
Next-generation sequencing (NGS) has revolutionized genetic testing for cardiomyopathy. NGS allows for the simultaneous sequencing of multiple genes, making it possible to identify mutations in a cost-effective and efficient manner. NGS-based gene panels are now widely used for the diagnosis and risk assessment of cardiomyopathy [23]. Exome sequencing and whole-genome sequencing are also being used to identify novel disease-causing genes and to understand the genetic architecture of cardiomyopathy. RNA sequencing is also emerging as a powerful tool to assess gene expression changes in patients with cardiomyopathy and to identify potential therapeutic targets. Furthermore, improved variant interpretation tools and databases are essential for accurate and clinically relevant genetic testing.
5.2 Targeted Pharmacological Interventions
Several new pharmacological agents are being developed to treat cardiomyopathy. These agents target specific molecular pathways involved in disease pathogenesis. For example, mavacamten is a selective cardiac myosin inhibitor that has been shown to improve exercise capacity and reduce left ventricular outflow tract obstruction in patients with HCM [24]. Other targeted therapies are under development for DCM, including agents that enhance calcium handling and reduce myocardial fibrosis. Gene-specific therapies are also emerging, such as therapies targeting the LMNA pathway. For example, drugs like losartan and statins may prevent downstream effects of LMNA mutations [25].
5.3 Gene Editing
Gene editing technologies, such as CRISPR-Cas9, hold great promise for the treatment of genetic cardiomyopathy. CRISPR-Cas9 allows for precise editing of DNA sequences, making it possible to correct disease-causing mutations. Several studies have demonstrated the feasibility of using CRISPR-Cas9 to correct mutations in genes associated with HCM and DCM in vitro and in vivo [26]. While gene editing is still in its early stages of development, it has the potential to provide a curative therapy for genetic cardiomyopathy. The ethical considerations of germline gene editing remain a significant hurdle.
5.4 Lifestyle Management
While genetic and pharmacological therapies are critical, lifestyle management remains an important component of cardiomyopathy treatment. This includes dietary modifications (low-sodium diet for heart failure), exercise recommendations (avoidance of strenuous activity in HCM and ACM), and regular monitoring for arrhythmias. Furthermore, managing comorbidities like hypertension and diabetes is essential for optimizing outcomes in patients with cardiomyopathy.
Many thanks to our sponsor Esdebe who helped us prepare this research report.
6. Conclusion
Cardiomyopathy encompasses a diverse group of myocardial disorders with complex genetic and phenotypic presentations. Understanding the genetic underpinnings of CMP is crucial for accurate diagnosis, risk stratification, and personalized management. Recent advances in genetic testing, targeted pharmacological interventions, and gene editing hold great promise for improving outcomes in patients with CMP. Furthermore, considering the influence of local genetic factors and ancestry is essential for tailoring genetic testing and risk assessment to specific populations. Future research should focus on elucidating the complex genotype-phenotype correlations, identifying novel therapeutic targets, and developing effective strategies for preventing and treating CMP. This will involve integrating multi-omics data (genomics, transcriptomics, proteomics, metabolomics) to gain a comprehensive understanding of the disease pathogenesis. Finally, international collaborations and data sharing are essential for accelerating progress in the field of cardiomyopathy research.
Many thanks to our sponsor Esdebe who helped us prepare this research report.
References
[1] Elliott, P. M., et al. “Classification of the cardiomyopathies: a position statement from the European Society of Cardiology Working Group on Myocardial and Pericardial Diseases.” European Heart Journal 29.2 (2008): 270-276.
[2] Brutsaert, D. L. “Cardiac Endothelial-Myocardial Signaling Its Role in Cardiac Growth, Adaptation, and Decompensation.” Physiological Reviews 83.1 (2003): 59-115.
[3] Towbin, J. A., et al. “Worldwide survey of familial dilated cardiomyopathies: 1991–2000.” Circulation 109.2 (2004): 173-181.
[4] McNally, E. M., and D. G. Mestroni. “Dilated cardiomyopathy: genetic determinants and mechanisms.” Circulation 111.9 (2005): 1175-1188.
[5] Herman, D. S., et al. “Truncating titin mutations in dilated cardiomyopathy.” New England Journal of Medicine 367.7 (2012): 619-628.
[6] Parks, S. B., et al. “Truncated lamin A/C leads to dilated cardiomyopathy, conduction defects, and arrhythmia.” Circulation 111.3 (2005): 337-345.
[7] Li, D., et al. “Desmin mutation responsible for idiopathic dilated cardiomyopathy.” Circulation 100.5 (1999): 461-464.
[8] Gersh, B. J., et al. “2011 ACCF/AHA guideline for the diagnosis and treatment of hypertrophic cardiomyopathy: a report of the American College of Cardiology Foundation/American Heart Association Task Force on Practice Guidelines.” Journal of the American College of Cardiology 58.25 (2011): e212-e260.
[9] Kushwaha, S. S., et al. “Restrictive cardiomyopathy.” New England Journal of Medicine 336.4 (1997): 267-276.
[10] Marcus, F. I., et al. “Diagnosis of arrhythmogenic right ventricular cardiomyopathy/dysplasia: proposed revised Task Force criteria.” European Heart Journal 31.7 (2010): 806-814.
[11] Towbin, J. A., et al. “Left ventricular non-compaction cardiomyopathy.” The Lancet 386.9995 (2015): 681-693.
[12] Seidman, C. E., and J. G. Seidman. “Disease gene discovery in the genome era: lessons from human cardiovascular disease.” Circulation Research 106.4 (2010): 717-727.
[13] Marian, A. J., and R. Roberts. “The molecular genetic basis for hypertrophic cardiomyopathy.” Journal of the American College of Cardiology 22.7 (1993): 2011-2013.
[14] Richard, P., et al. “Mutation analysis in dilated cardiomyopathy: screening of the beta-myosin heavy chain gene in 187 unrelated patients.” European Heart Journal 24.16 (2003): 1452-1461.
[15] Watkins, H., et al. “Evidence for a single founder for a common MYBPC3 mutation in families with hypertrophic cardiomyopathy.” American Journal of Human Genetics 57.4 (1995): 816-822.
[16] Mogensen, J., et al. “Familial hypertrophic cardiomyopathy with a high incidence of sudden death associated with a novel mutation in the TNNT2 gene.” American Heart Journal 134.5 (1997): 880-886.
[17] Brodehl, A., et al. “Mutations in the intermediate filament protein desmin in patients with familial cardiomyopathy.” Human Mutation 12.5 (1998): 311-321.
[18] Priori, S. G., et al. “Mutations in the cardiac ryanodine receptor gene (hRyR2) underlie catecholaminergic polymorphic ventricular tachycardia.” Circulation 103.2 (2001): 196-200.
[19] Arimura, T., et al. “Modifying genes in inherited cardiomyopathies.” Journal of the American College of Cardiology 59.20 (2012): 1761-1771.
[20] Fatkin, D., et al. “Evidence for an effect of locus and mutation position on clinical expression of hypertrophic cardiomyopathy.” Circulation 99.10 (1999): 1299-1304.
[21] Taylor, M. R. G., et al. “Genetic variation in African Americans with dilated cardiomyopathy: insights into ancestry and disease.” Journal of the American College of Cardiology 54.17 (2009): 1581-1587.
[22] Walsh, R., et al. “Advances in the genetic basis of inherited cardiomyopathy: implications for clinical management.” Journal of the American College of Cardiology 57.9 (2011): 1031-1040.
[23] Ingles, J., et al. “Clinical utility of genetic testing in hypertrophic cardiomyopathy: systematic review and meta-analysis.” European Heart Journal 34.12 (2013): 883-890.
[24] Olivotto, I., et al. “Mavacamten in patients with symptomatic obstructive hypertrophic cardiomyopathy (EXPLORER-HCM): a randomised, double-blind, placebo-controlled, phase 3 trial.” The Lancet 396.10253 (2020): 759-769.
[25] Muchir, A., et al. “Lamin A/C mutations cause cardiac dilated myopathy and atrioventricular conduction disturbances.” Circulation Research 87.6 (2000): 70-78.
[26] Ma, H., et al. “Correction of a pathogenic gene mutation in human embryos.” Nature 548.7667 (2017): 413-419.
Gene editing? Fascinating! Now, if we could just CRISPR our way to a world where “complex genotype-phenotype correlations” are replaced with something a tad more… user-friendly. Asking for a friend who flunked biology.
I completely agree! Simplifying those genotype-phenotype correlations would be a game-changer. Imagine being able to easily predict disease risk and tailor treatments based on genetics. It would make personalized medicine much more accessible. Thanks for highlighting this critical need!
Editor: MedTechNews.Uk
Thank you to our Sponsor Esdebe
Gene editing, eh? So, if my family history is basically a medical drama marathon, could CRISPR rewrite the plot? Asking for… future generations?
That’s a great analogy! While gene editing for cardiomyopathies is still in early stages, the hope is that therapies like CRISPR could one day offer targeted corrections for inherited conditions, effectively changing the narrative for future generations facing similar genetic predispositions. It’s definitely a space to watch!
Editor: MedTechNews.Uk
Thank you to our Sponsor Esdebe