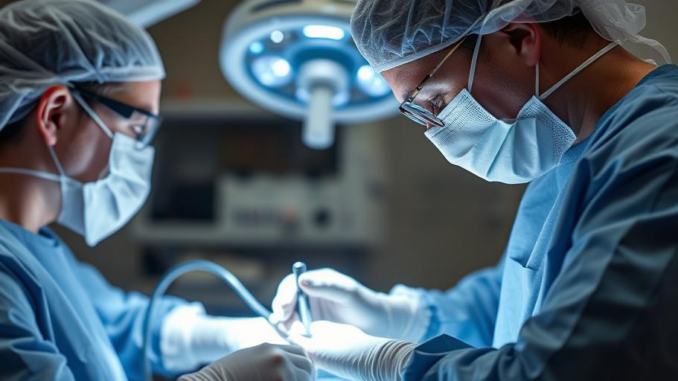
A Comprehensive Review of Electrosurgery: Principles, Evolution, and Future Directions
Abstract
Electrosurgery, a cornerstone of modern surgical practice, has undergone significant evolution since its inception. This review provides a comprehensive overview of electrosurgical principles, mechanisms of action, and the technological advancements that have shaped its trajectory. We examine the historical progression of electrosurgical generators, from early spark-gap devices to contemporary solid-state systems with adaptive tissue technology. Different electrosurgical modalities, including monopolar and bipolar techniques, cutting, coagulation, and desiccation, are discussed in detail, alongside their respective applications and limitations. Safety considerations, including the risks of thermal injury, smoke plume hazards, and electromagnetic interference, are thoroughly addressed, emphasizing strategies for mitigation. Furthermore, this review explores the impact of electrosurgery on surgical outcomes, efficiency, and the potential for future developments in areas such as plasma-based electrosurgery, robotic integration, and artificial intelligence-driven control systems. Ultimately, this paper aims to provide an expert-level perspective on the current state of electrosurgery and its potential to enhance surgical practice in the years to come.
1. Introduction
Electrosurgery, the application of electrical energy to biological tissue for therapeutic purposes, has revolutionized surgical procedures since its introduction in the early 20th century. From simple cutting and coagulation to complex ablation and tissue welding, electrosurgical techniques have become indispensable across a wide range of surgical specialties. Its ability to precisely control tissue interaction, minimize blood loss, and reduce operative time has significantly improved surgical outcomes and patient safety. This review aims to provide a comprehensive and in-depth analysis of electrosurgery, covering its underlying principles, historical evolution, current technologies, safety considerations, and future directions. The discussion will assume a background knowledge consistent with an experienced surgical practitioner or biomedical engineer familiar with the fundamentals of electricity and tissue biology.
2. Historical Perspective and Evolution of Electrosurgical Generators
Electrosurgery’s origins can be traced back to the pioneering work of Jacques-Arsène d’Arsonval and William T. Bovie. D’Arsonval’s experiments in the late 19th century demonstrated the therapeutic effects of high-frequency currents, while Bovie, in collaboration with Harvey Cushing, developed the first practical electrosurgical generator in 1926. This early device, a spark-gap generator, produced damped sinusoidal waveforms that were primarily used for coagulation and desiccation. The spark-gap generator worked by producing high-voltage alternating current, which would arc between two electrodes, creating heat to destroy tissue. Though effective for some applications, they were relatively imprecise, difficult to control, and produced significant tissue damage.
The introduction of vacuum-tube generators in the mid-20th century represented a significant improvement. These generators produced continuous sinusoidal waveforms, allowing for more precise cutting and coagulation with reduced tissue charring. This allowed for the development of new techniques, such as electrosurgical resection of the prostate (TURP) and advanced applications in gastrointestinal surgery. Vacuum-tube generators offered better control over power output and waveform characteristics compared to spark-gap devices.
The advent of solid-state electronics in the late 20th century ushered in a new era of electrosurgical technology. Transistorized and, subsequently, microprocessor-controlled generators offered improved reliability, stability, and control. Solid-state generators enabled the development of advanced electrosurgical modalities, such as argon plasma coagulation (APC) and bipolar electrosurgery, and allowed for integration with surgical navigation systems. Modern electrosurgical generators utilize sophisticated algorithms to monitor tissue impedance and adjust power output in real-time, optimizing surgical performance and minimizing thermal damage.
3. Principles of Electrosurgical Tissue Interaction
Electrosurgery relies on the conversion of electrical energy into heat within biological tissue. The extent and type of tissue damage depend on several factors, including the waveform characteristics, power density, electrode configuration, and tissue properties. The interaction between electrical current and tissue is governed by Joule’s law, which states that the heat generated is proportional to the square of the current, the resistance of the tissue, and the duration of current flow (H = I²Rt). The resistance of tissue varies significantly depending on its composition and water content.
Different electrosurgical waveforms produce distinct tissue effects. Continuous sinusoidal waveforms, typically associated with cutting, produce rapid heating and vaporization of intracellular water, resulting in cellular disruption and tissue separation. Intermittent or burst waveforms, used for coagulation, induce slower heating and protein denaturation, leading to hemostasis. Damped waveforms, produced by older spark-gap generators, generate significant tissue necrosis and are generally avoided in modern electrosurgery.
Power density, defined as the amount of power delivered per unit area of tissue, is a critical determinant of tissue effect. High power densities result in rapid tissue vaporization and cutting, while lower power densities induce coagulation or desiccation. Electrode size and shape also influence power density. Smaller electrodes concentrate the current, producing higher power densities and enhanced cutting ability, while larger electrodes distribute the current over a wider area, promoting coagulation.
4. Electrosurgical Modalities: Monopolar and Bipolar
Electrosurgery is broadly classified into two main modalities: monopolar and bipolar.
4.1 Monopolar Electrosurgery: In monopolar electrosurgery, the active electrode is applied to the surgical site, and the return electrode (grounding pad) is placed elsewhere on the patient’s body. The electrical current flows from the generator, through the active electrode, through the patient’s body, and back to the generator via the return electrode. Monopolar electrosurgery is versatile and can be used for cutting, coagulation, desiccation, and fulguration. However, because the current passes through a large portion of the patient’s body, there is a risk of unintended thermal injury at the return electrode site, especially if the contact area is inadequate. It also tends to produce more plume than Bipolar.
4.2 Bipolar Electrosurgery: Bipolar electrosurgery confines the electrical current to a small area between the two electrodes of the instrument. Both electrodes are located at the surgical site, eliminating the need for a return electrode. This modality is particularly suitable for precise coagulation of small vessels and tissues, minimizing the risk of stray current injuries. Bipolar electrosurgery is widely used in neurosurgery, microsurgery, and laparoscopic procedures. Tissue desiccation, also called “drying”, uses lower voltages than coagulation to cause dehydration of the tissue. Bipolar is particularly effective in wet conditions.
5. Advanced Electrosurgical Technologies
5.1 Argon Plasma Coagulation (APC): APC utilizes argon gas to conduct electrical current to the tissue surface. The argon gas is ionized by the electrosurgical generator, creating a plasma arc that provides non-contact coagulation. APC is particularly useful for hemostasis of diffuse bleeding, such as in the gastrointestinal tract, and for debulking tumors. The non-contact nature of APC minimizes tissue adhesion and reduces the risk of perforation.
5.2 Ultrasonic Dissection and Coagulation: While not strictly electrosurgery, ultrasonic devices are often used in conjunction with electrosurgical techniques. Ultrasonic instruments use high-frequency mechanical vibrations to denature proteins and coagulate tissues. These devices offer precise dissection and coagulation with minimal thermal spread, making them suitable for delicate surgical procedures. Examples include Harmonic Scalpel.
5.3 Adaptive Tissue Technology: Modern electrosurgical generators incorporate adaptive tissue technology, which automatically adjusts the power output and waveform characteristics based on real-time tissue impedance measurements. This technology optimizes surgical performance, minimizes thermal damage, and reduces the need for manual adjustments. Adaptive tissue technology represents a significant advancement in electrosurgical safety and efficacy.
6. Safety Considerations and Complications
Electrosurgery, while generally safe, is associated with several potential risks and complications. Proper training, adherence to safety protocols, and careful selection of electrosurgical parameters are essential for minimizing these risks.
6.1 Thermal Injury: Thermal injury is the most common complication of electrosurgery. Unintended burns can occur at the active electrode site due to excessive power, prolonged application, or improper technique. In monopolar electrosurgery, burns can also occur at the return electrode site due to inadequate contact or improper placement. Strategies for preventing thermal injury include using the lowest effective power setting, applying the active electrode intermittently, ensuring adequate contact between the return electrode and the patient, and avoiding the use of flammable materials near the surgical site.
6.2 Smoke Plume Hazards: Electrosurgical smoke plume contains toxic gases, particulate matter, and potentially viable microorganisms. Inhalation of smoke plume can cause respiratory irritation, eye irritation, and potentially long-term health effects. Smoke evacuation systems should be used to effectively remove smoke plume from the surgical field. Proper ventilation and personal protective equipment, such as masks and eye protection, are essential for protecting surgical personnel.
6.3 Electromagnetic Interference (EMI): Electrosurgical devices can generate electromagnetic interference that can affect other electronic equipment in the operating room, such as cardiac monitors and pacemakers. Shielding cables, grounding equipment, and maintaining adequate distance between electrosurgical devices and other electronic equipment can minimize EMI. Consultation with biomedical engineers is recommended to ensure electromagnetic compatibility in the operating room. Patients with implanted electronic devices may require special monitoring and precautions during electrosurgical procedures.
6.4 Other Complications: Other potential complications of electrosurgery include bleeding, infection, delayed wound healing, and nerve damage. Careful surgical technique, proper wound care, and appropriate antibiotic prophylaxis can minimize these risks.
7. Impact on Surgical Outcomes and Efficiency
Electrosurgery has significantly improved surgical outcomes and efficiency in a wide range of surgical specialties. The ability to precisely cut and coagulate tissues has reduced blood loss, shortened operative times, and improved visualization of the surgical field. Electrosurgery has also enabled the development of minimally invasive surgical techniques, such as laparoscopy and endoscopy, which offer reduced pain, shorter hospital stays, and faster recovery times compared to traditional open surgery.
Adaptive tissue technology has further enhanced the benefits of electrosurgery. By automatically adjusting power output and waveform characteristics based on real-time tissue impedance measurements, adaptive tissue technology optimizes surgical performance, minimizes thermal damage, and reduces the need for manual adjustments. This technology has been shown to improve hemostasis, reduce tissue charring, and accelerate wound healing.
8. Future Directions and Emerging Technologies
The field of electrosurgery continues to evolve, with ongoing research and development focused on improving safety, efficacy, and versatility. Several promising areas of development include:
8.1 Plasma-Based Electrosurgery: Plasma-based electrosurgery utilizes ionized gas to create a plasma arc that interacts with tissue. Plasma-based devices offer precise cutting and coagulation with minimal thermal spread, making them suitable for delicate surgical procedures. Emerging plasma technologies include cold atmospheric plasma (CAP), which has shown promise for wound healing and sterilization.
8.2 Robotic Integration: Integration of electrosurgical devices with robotic surgical systems offers enhanced precision, dexterity, and control. Robotic electrosurgery allows surgeons to perform complex procedures with greater accuracy and efficiency. Future developments may include haptic feedback and automated tissue recognition to further enhance robotic electrosurgical performance.
8.3 Artificial Intelligence (AI)-Driven Control Systems: AI-driven control systems have the potential to optimize electrosurgical parameters based on real-time tissue analysis. AI algorithms can analyze tissue impedance, optical properties, and other parameters to automatically adjust power output, waveform characteristics, and electrode configuration. AI-driven control systems could improve surgical outcomes, reduce complications, and simplify electrosurgical procedures.
8.4 Energy-Based Tissue Welding: Rather than rely on suturing or stapling, energy-based tissue welding techniques (e.g., using radiofrequency energy) are gaining traction. These methods offer the potential to create strong, leak-proof anastomoses with reduced inflammatory response and improved healing. However, standardized protocols and long-term outcome data are still needed.
9. Conclusion
Electrosurgery has fundamentally transformed modern surgical practice, offering a versatile and effective means of cutting, coagulating, and ablating tissues. From the early spark-gap generators to contemporary solid-state systems with adaptive tissue technology, electrosurgery has undergone continuous evolution. Understanding the principles of electrosurgical tissue interaction, the different electrosurgical modalities, and the associated safety considerations is essential for ensuring optimal surgical outcomes and minimizing complications. Ongoing research and development in areas such as plasma-based electrosurgery, robotic integration, and AI-driven control systems promise to further enhance the safety, efficacy, and versatility of electrosurgery in the years to come. As electrosurgery technology continues to advance, it is crucial for surgeons and biomedical engineers to stay abreast of the latest developments and to incorporate these advancements into their practice to improve patient care and surgical outcomes.
References
- Pearce, J. A. (2018). Electrosurgery. John Wiley & Sons.
- Sigel, J. E., III, Chaudry, G., & Bleier, B. S. (2018). Electrosurgery 101: Principles and best practices. Otolaryngologic Clinics of North America, 51(5), 895-905.
- Farah, R. S., Dipti, A., Shahrudin, N. A., & Shahidan, P. P. (2021). Electrosurgical generators: Principles, classification, and clinical implications. Journal of Biomedical Science and Engineering, 14(02), 31.
- Cody, E., & Thomson, J. F. (2011). Electrosurgery. Surgical Clinics of North America, 91(5), 1047-1064.
- Becker, D. G., Lillis, P., & Vertrees, A. E. (2017). Radiofrequency ablation for varicose veins. Seminars in Interventional Radiology, 34(04), 388-396.
- Kennedy, G. D., Martin, D. C., & Overstreet, K. A. (2014). Smoke evacuation in the operating room: the quest for best practice. Perioperative Nursing Clinics, 9(3), 301-308.
- Ohtake, H., & Komasawa, N. (2021). Prevention of electromagnetic interference of electrosurgery on medical devices: a narrative review. Journal of Anesthesia, 35(3), 443-451.
- Szczesny-Stankiewicz, B., Gubbels, S., Walczak, P., & van Vliet, E. A. (2023). Cold atmospheric plasma technology: a review of its applications in wound treatment, disinfection and oncology. Antioxidants, 12(2), 307.
- Ballantyne GH. Robotic surgery, telerobotic surgery, telepresence, and telementoring. Review of early clinical results. Surg Endosc 2002;16:1389-402.
- Khan, M. A., et al. (2023). Artificial intelligence in surgery: applications, challenges, and future directions. Annals of Surgery, 277(4), 593-604.
- Vidal PM, Jürgensen K, Tittel A, et al. Tissue welding for pancreatic anastomosis: A systematic review and meta-analysis of preclinical and clinical studies. J Surg Res. 2022;278:236-248.
Be the first to comment