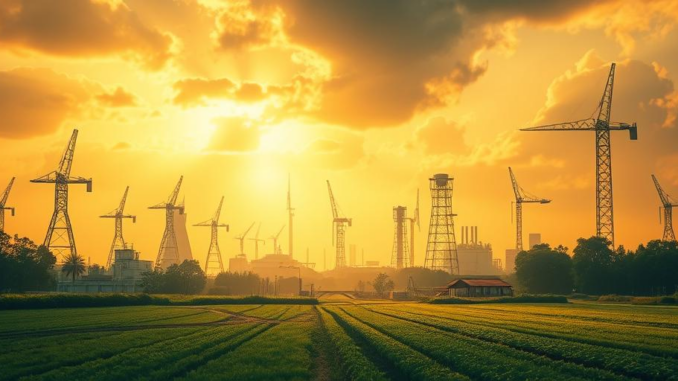
A Comprehensive Review of Radiation: Sources, Biological Effects, and Mitigation Strategies
Abstract: Radiation, an intrinsic component of the natural world and increasingly harnessed for technological applications, presents a complex interplay of benefits and risks. This report provides a comprehensive review of radiation, encompassing its diverse sources (natural and anthropogenic), mechanisms of interaction with biological matter, acute and chronic health effects, and cutting-edge mitigation strategies. We delve into the complexities of radiation dosimetry, discuss the latest research on low-dose radiation exposure, explore the role of individual susceptibility, and critically evaluate current safety standards and future research directions. This review is intended for experts in radiation science, medical physics, environmental health, and related fields.
1. Introduction: The Ubiquitous Nature of Radiation
Radiation, broadly defined as energy propagating through space or matter, is a fundamental phenomenon permeating the universe. It exists in diverse forms, spanning the electromagnetic spectrum from low-frequency radio waves to high-energy gamma rays, and includes particulate radiation such as alpha and beta particles. Our exposure to radiation is inevitable, arising from natural sources like cosmic rays, terrestrial radionuclides (e.g., uranium, thorium, radon), and internal radioactive isotopes (e.g., potassium-40). However, human activities, particularly the development and deployment of nuclear technologies, medical imaging, and industrial processes, have significantly augmented our exposure to anthropogenic radiation sources.
The double-edged sword of radiation is evident in its widespread applications and potential hazards. Radiation-based technologies are invaluable in medicine (diagnosis and therapy), industry (sterilization, gauging, non-destructive testing), and energy production (nuclear power). Yet, exposure to ionizing radiation, in particular, can cause substantial biological damage, ranging from transient cellular dysfunction to severe health consequences like cancer and genetic mutations. Understanding the intricate interactions of radiation with living organisms, quantifying associated risks, and developing effective mitigation strategies are crucial for safeguarding public health and ensuring the responsible use of radiation-based technologies.
2. Sources and Types of Radiation
A detailed categorization of radiation sources and types is paramount to comprehending their varying biological effects and implementing targeted protection measures.
- Natural Background Radiation: This constitutes the dominant source of human radiation exposure globally. Cosmic radiation, originating from extraterrestrial sources, interacts with the Earth’s atmosphere, producing secondary particles that reach the surface. Terrestrial radiation stems from naturally occurring radioactive materials (NORM) present in soil, rocks, and building materials. Radon gas, a decay product of uranium, is a significant contributor to indoor radiation exposure, particularly in areas with high uranium concentrations in the underlying geology. Internal radiation arises from radionuclides incorporated into the human body through food, water, and air.
- Anthropogenic Radiation: Human-made radiation sources encompass a wide array of technologies and applications. Medical imaging, including X-rays, computed tomography (CT), and nuclear medicine, represents the largest contributor to anthropogenic radiation exposure for the general population. Nuclear power generation, while contributing less than medical imaging, releases radioactive effluents during normal operations and poses the risk of accidental releases. Industrial applications of radiation, such as sterilization of medical supplies and food irradiation, also contribute to public exposure. Furthermore, occupational exposure to radiation is a concern in industries such as mining, manufacturing, and research.
Beyond the source, the type of radiation is crucial in determining its biological impact. Ionizing radiation, including alpha particles, beta particles, gamma rays, and X-rays, possesses sufficient energy to remove electrons from atoms and molecules, leading to ionization and the formation of free radicals. Non-ionizing radiation, such as radio waves, microwaves, and visible light, lacks the energy to cause ionization but can induce thermal effects and potentially other subtle biological changes.
3. Mechanisms of Radiation Interaction with Biological Matter
The biological effects of radiation are primarily mediated by the deposition of energy within cells and tissues. Ionizing radiation interacts with biological molecules, particularly water, leading to the generation of reactive oxygen species (ROS) and other free radicals. These free radicals can damage DNA, proteins, and lipids, initiating a cascade of cellular events. The direct ionization of DNA can also lead to strand breaks and base modifications.
The cellular response to radiation-induced damage is complex and involves DNA repair mechanisms, cell cycle checkpoints, and programmed cell death (apoptosis). The efficiency of DNA repair varies depending on the type and extent of damage, as well as the individual’s genetic background. If DNA damage is not adequately repaired, it can lead to mutations, genomic instability, and ultimately, cancer. Apoptosis serves as a protective mechanism to eliminate cells with irreparable damage, preventing the propagation of mutations. However, excessive apoptosis can contribute to tissue damage and organ dysfunction.
Low-dose radiation exposure (<100 mSv) is a particularly contentious area of research. While the linear no-threshold (LNT) model, which assumes that any dose of radiation, no matter how small, carries a risk of cancer, is widely used for regulatory purposes, some studies suggest that low doses may have hormetic effects, stimulating protective mechanisms and reducing the risk of certain diseases. The evidence for hormesis is still debated, and the underlying mechanisms remain unclear. Potential mechanisms include the activation of antioxidant defense systems, increased DNA repair capacity, and enhanced immune surveillance.
4. Acute and Chronic Health Effects of Radiation Exposure
The health effects of radiation exposure are broadly categorized as acute (short-term) and chronic (long-term). Acute effects typically occur after exposure to high doses of radiation (>1 Sv) over a short period, while chronic effects manifest years or decades after exposure, even at lower doses.
- Acute Radiation Syndrome (ARS): ARS, also known as radiation sickness, is a constellation of symptoms resulting from the rapid destruction of cells in radiosensitive tissues such as the bone marrow, gastrointestinal tract, and skin. The severity of ARS depends on the dose received. Symptoms can include nausea, vomiting, fatigue, hair loss, skin burns, and hemorrhage. In severe cases, ARS can lead to death due to bone marrow failure, infection, and multiple organ dysfunction.
- Chronic Health Effects: The most significant long-term health effect of radiation exposure is an increased risk of cancer. Numerous epidemiological studies have demonstrated a clear association between radiation exposure and increased incidence of leukemia, breast cancer, thyroid cancer, lung cancer, and other solid tumors. The risk of cancer increases with increasing dose, although the magnitude of the risk at low doses remains uncertain. Other potential chronic health effects of radiation exposure include cardiovascular disease, cataracts, and cognitive decline.
The latency period between radiation exposure and cancer development can be decades. This makes it challenging to establish causal relationships and to accurately estimate the lifetime risk of cancer following radiation exposure. Furthermore, other factors, such as genetic predisposition, lifestyle, and environmental exposures, can influence an individual’s susceptibility to radiation-induced cancer.
5. Individual Susceptibility and Modifying Factors
The biological response to radiation exposure is not uniform across individuals. Genetic factors, age, sex, and pre-existing health conditions can significantly influence an individual’s susceptibility to radiation-induced damage. Some individuals may be more efficient at DNA repair, while others may have a higher propensity for developing cancer.
- Genetic Predisposition: Mutations in genes involved in DNA repair, cell cycle control, and apoptosis can increase an individual’s risk of developing cancer following radiation exposure. For example, individuals with mutations in the BRCA1 or BRCA2 genes, which are involved in DNA repair, have a higher risk of developing breast and ovarian cancer. Other genetic factors, such as variations in genes involved in immune function and inflammation, may also influence radiation sensitivity.
- Age: Children are generally more radiosensitive than adults due to their rapidly dividing cells and longer lifespan to develop cancer. Radiation exposure during childhood can increase the lifetime risk of cancer, particularly leukemia and thyroid cancer. Older adults may also be more vulnerable to radiation-induced damage due to age-related decline in DNA repair capacity and immune function.
- Sex: Some studies suggest that women may be more susceptible to radiation-induced cancer than men, particularly for breast and thyroid cancer. Hormonal factors may play a role in this increased susceptibility. However, further research is needed to confirm these findings.
- Pre-existing Health Conditions: Individuals with pre-existing health conditions, such as immune deficiencies, chronic inflammation, and certain genetic disorders, may be more vulnerable to radiation-induced damage. These conditions can impair DNA repair, immune function, and other cellular processes that are important for protecting against radiation-induced damage.
6. Radiation Dosimetry and Risk Assessment
Accurate radiation dosimetry is essential for assessing the risks associated with radiation exposure. Dosimetry involves measuring or estimating the amount of radiation energy absorbed by the body or specific organs. Different dosimetry methods are used depending on the type of radiation, the exposure scenario, and the desired level of accuracy.
- External Dosimetry: External dosimetry methods are used to measure radiation exposure from sources outside the body. These methods typically involve the use of radiation detectors, such as Geiger-Muller counters, ionization chambers, and thermoluminescent dosimeters (TLDs). TLDs are commonly used to monitor occupational radiation exposure.
- Internal Dosimetry: Internal dosimetry methods are used to estimate radiation exposure from radionuclides incorporated into the body. These methods involve measuring the amount of radioactivity in body fluids (e.g., urine, blood) or tissues, and using biokinetic models to estimate the radiation dose to specific organs. Whole-body counters are also used to measure the amount of radioactivity in the entire body.
Risk assessment involves estimating the probability of adverse health effects resulting from radiation exposure. Risk assessments are typically based on epidemiological data from studies of radiation-exposed populations, such as atomic bomb survivors and nuclear workers. The International Commission on Radiological Protection (ICRP) provides recommendations for radiation protection based on risk assessments. The ICRP’s recommendations are widely used by regulatory agencies around the world.
7. Mitigation Strategies and Radiation Protection Principles
The fundamental principles of radiation protection are justification, optimization, and limitation. Justification requires that any practice involving radiation exposure must be justified by its benefits outweighing the risks. Optimization requires that radiation doses should be kept as low as reasonably achievable (ALARA), taking into account economic and social factors. Limitation requires that radiation doses to individuals should not exceed specified limits.
- Shielding: Shielding involves placing barriers between the radiation source and individuals to reduce radiation exposure. The effectiveness of shielding depends on the type of radiation and the shielding material. For example, lead is an effective shielding material for X-rays and gamma rays, while concrete is effective for shielding neutrons.
- Distance: The intensity of radiation decreases with distance from the source. Increasing the distance between individuals and the radiation source is an effective way to reduce radiation exposure. The inverse square law dictates this relationship for point sources.
- Time: Limiting the time of exposure to radiation reduces the total dose received. This is particularly important in occupational settings where workers may be exposed to radiation on a regular basis. Rotating staff to reduce the time an individual is exposed to radiation is a common strategy.
- Medical Imaging Optimization: Optimization of medical imaging procedures is crucial for minimizing radiation exposure to patients. This involves using the lowest possible radiation dose that is necessary to obtain diagnostic images, avoiding unnecessary examinations, and using alternative imaging techniques with lower radiation doses when appropriate (e.g., MRI or ultrasound). Training radiographers and radiologists in best practices for radiation safety is also essential.
- Public Education and Communication: Effective communication of radiation risks to the public is essential for promoting informed decision-making and reducing anxiety. Public education campaigns can help to raise awareness about the sources of radiation exposure and the measures that individuals can take to reduce their exposure. Transparency and honesty are key to building public trust.
8. Future Research Directions
Despite significant advances in our understanding of radiation biology and protection, several areas require further research.
- Low-Dose Radiation Effects: More research is needed to clarify the health effects of low-dose radiation exposure, particularly the potential for hormetic effects. Large-scale epidemiological studies with accurate dosimetry are needed to improve our estimates of cancer risk at low doses. Furthermore, research is needed to identify biomarkers of radiation exposure and susceptibility.
- Individual Susceptibility: Further research is needed to identify the genetic and environmental factors that influence individual susceptibility to radiation-induced damage. This knowledge could be used to develop personalized radiation protection strategies.
- Radiation Countermeasures: Research is needed to develop effective countermeasures to mitigate the effects of radiation exposure. These countermeasures could include radioprotectors, which protect cells from radiation damage, and radiomitigators, which promote tissue repair after radiation exposure.
- Advanced Dosimetry Techniques: Advancements in dosimetry techniques are needed to improve the accuracy of radiation dose estimates, particularly for internal exposure. This includes the development of more sensitive and specific methods for measuring radionuclides in body fluids and tissues.
- Risk Communication: Improved methods for communicating radiation risks to the public are needed. These methods should be tailored to different audiences and should address common misconceptions about radiation.
9. Conclusion
Radiation is an inescapable part of our environment, with both natural and anthropogenic sources contributing to human exposure. While radiation-based technologies offer numerous benefits in medicine, industry, and energy production, it is crucial to understand the potential health risks associated with radiation exposure and to implement effective mitigation strategies. Ongoing research is essential to refine our understanding of low-dose radiation effects, identify factors influencing individual susceptibility, and develop new radiation countermeasures. By adhering to the principles of justification, optimization, and limitation, we can ensure the responsible use of radiation and protect public health.
References
- United Nations Scientific Committee on the Effects of Atomic Radiation (UNSCEAR). (2008). Sources and effects of ionizing radiation: UNSCEAR 2008 report to the General Assembly, with scientific annexes. United Nations.
- International Commission on Radiological Protection (ICRP). (2007). The 2007 recommendations of the International Commission on Radiological Protection. ICRP Publication 103. Annals of the ICRP, 37(2-4).
- National Research Council (NRC). (2006). Health risks from exposure to low levels of ionizing radiation: BEIR VII Phase 2. National Academies Press.
- Tubiana, M., Feinendegen, L. E., Yang, C., & Kaminski, J. M. (2009). The linear no-threshold relationship is inconsistent with radiation biologic and experimental data. Radiology, 251(1), 13-22.
- Pollycove, M., & Feinendegen, L. E. (2001). Radiation-induced versus endogenous DNA damage: possible effect of induced adaptive responses. Human & Experimental Toxicology, 20(6), 275-286.
- Little, M. P., Azizova, T. V., Bazyka, D., Bouffler, S. D., Cardis, E., Chekin, S., … & Zablotska, L. B. (2012). Systematic review and meta-analysis of epidemiological studies of thyroid cancer and ionizing radiation. Environmental Health Perspectives, 120(12), 1595-1603.
- Brenner, D. J., & Hall, E. J. (2007). Computed tomography—an increasing source of radiation exposure. New England Journal of Medicine, 357(22), 2277-2284.
- Mettler Jr, F. A., Bhargavan, M., Faulkner, K., Gilley, D. B., Gray, J. E., Ibbott, G. S., … & Mahesh, M. (2009). Patient exposure from radiologic and nuclear medicine procedures in the United States: procedure volume survey. Radiology, 253(3), 652-661.
- Hendee, W. R., Willis, C. E., & Bornier, R. B. (1998). Medical radiation exposure of patients in the United States: preliminary summary of a 1995 assessment. American Journal of Roentgenology, 170(3), 647-652.
- Committee on the Public Health Implications of Exposure to Naturally Occurring Radioactive Materials (NORM), National Research Council. Radiation on Earth: Exposure of the Population to Natural and Man-Made Sources. Washington, DC: The National Academies Press, 1999.
The discussion of individual susceptibility is particularly insightful. Exploring the interplay between genetic predisposition and environmental factors could lead to more personalized radiation protection strategies and improved risk assessments.