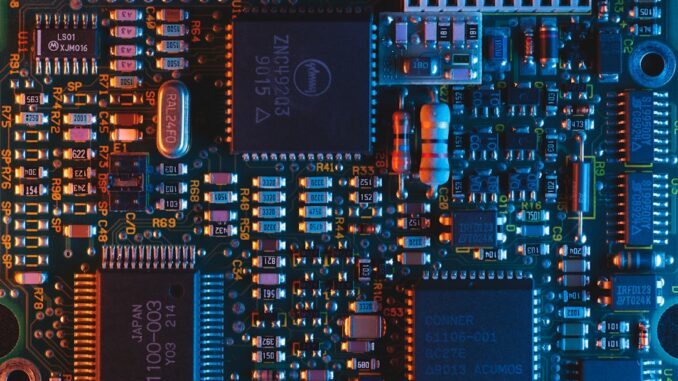
Abstract
This research report provides a comprehensive overview of advanced cardiac pacing, extending beyond conventional applications to encompass cutting-edge technologies, evolving clinical paradigms, and prospective innovations. We delve into the intricacies of physiological pacing modalities, including His-bundle pacing (HBP) and left bundle branch area pacing (LBBAP), highlighting their electrophysiological mechanisms and potential advantages over traditional right ventricular pacing. Furthermore, the report explores the role of leadless pacemakers, their implantation strategies, and long-term performance outcomes. Special attention is given to the integration of advanced sensor technologies and algorithms for rate-adaptive pacing and hemodynamic optimization. Emerging frontiers, such as biological pacemakers, wireless pacing systems, and artificial intelligence (AI)-driven pacing, are also discussed. Finally, the report addresses the challenges and future directions in cardiac pacing, emphasizing the need for personalized pacing strategies, improved device longevity, and enhanced remote monitoring capabilities. This report is intended for experts in the field of cardiac electrophysiology, providing a critical analysis of the current state of the art and a perspective on the future landscape of cardiac pacing.
Many thanks to our sponsor Esdebe who helped us prepare this research report.
1. Introduction
Cardiac pacing has revolutionized the management of bradyarrhythmias and heart failure, evolving from rudimentary devices delivering fixed-rate stimulation to sophisticated systems capable of physiological pacing, rate adaptation, and hemodynamic optimization. The primary goal of cardiac pacing is to restore or maintain adequate cardiac output by providing electrical impulses to the heart when the intrinsic conduction system is compromised. While conventional right ventricular (RV) pacing has been the mainstay for decades, it has been associated with adverse effects, including ventricular dyssynchrony, increased risk of heart failure, and atrial fibrillation. This has spurred the development of alternative pacing modalities aimed at preserving or restoring physiological ventricular activation.
This report explores the advancements in cardiac pacing technology and techniques, focusing on the principles, advantages, and limitations of physiological pacing strategies like His-bundle pacing (HBP) and left bundle branch area pacing (LBBAP). We also examine the evolution and clinical implications of leadless pacemakers. Moreover, the integration of advanced sensor technologies, algorithms, and remote monitoring capabilities are discussed. Finally, we delve into the potential of emerging technologies, such as biological pacemakers and AI-driven pacing, to reshape the future of cardiac rhythm management. This research report aims to provide a thorough and critical evaluation of the current state of cardiac pacing, offering insights into the evolving technological landscape and clinical considerations.
Many thanks to our sponsor Esdebe who helped us prepare this research report.
2. Physiological Pacing: His-Bundle and Left Bundle Branch Area Pacing
2.1 His-Bundle Pacing (HBP)
His-bundle pacing (HBP) involves directly stimulating the His-bundle, the proximal portion of the heart’s intrinsic conduction system. This preserves the natural sequence of ventricular activation, minimizing ventricular dyssynchrony and potentially mitigating the adverse effects associated with RV pacing. The advantages of HBP include the maintenance of physiological QRS morphology, the prevention of pacing-induced cardiomyopathy, and the ability to treat both bradycardia and certain forms of supraventricular tachycardia.
Technical challenges associated with HBP include achieving stable lead fixation at the His-bundle location, managing high pacing thresholds, and the risk of His-bundle capture failure or exit block. The learning curve for HBP lead implantation is relatively steep, requiring expertise in electrophysiological mapping and lead manipulation. Careful patient selection and procedural skill are crucial for achieving optimal HBP outcomes.
Clinical trials comparing HBP to RV pacing have demonstrated promising results, with HBP showing benefits in terms of improved cardiac function, reduced heart failure hospitalization rates, and lower incidence of atrial fibrillation [1, 2]. However, long-term studies with larger patient populations are needed to further validate these findings and establish the superiority of HBP over RV pacing in specific clinical scenarios. One such study [1], for example, indicated that HBP was associated with a lower risk of atrial fibrillation compared to RV pacing. Another study [2] highlighted the potential of HBP to improve cardiac function in patients with heart failure and atrioventricular block.
2.2 Left Bundle Branch Area Pacing (LBBAP)
Left bundle branch area pacing (LBBAP) is a more recently developed physiological pacing modality that involves stimulating the left bundle branch or its fascicles in the interventricular septum. Compared to HBP, LBBAP is technically easier to perform, offers more stable lead fixation, and is associated with lower pacing thresholds. LBBAP achieves ventricular synchronization by activating the left ventricle directly through the left bundle branch system, leading to improved hemodynamic performance and reduced ventricular dyssynchrony.
The mechanism of LBBAP involves capturing the left bundle branch or its fascicles, resulting in a characteristic pacing-induced QRS morphology. The optimal LBBAP location is determined by assessing the QRS morphology and pacing parameters during the implantation procedure. LBBAP has demonstrated promising results in patients with bradycardia and heart failure, showing improvements in cardiac function, exercise capacity, and quality of life [3, 4].
Compared to HBP, LBBAP offers several advantages, including a shorter learning curve, easier lead implantation, and more stable lead fixation. However, LBBAP also has certain limitations, such as the potential for capturing septal myocardium rather than the left bundle branch itself, and the risk of inducing left ventricular pacing-induced cardiomyopathy in some patients. Further research is needed to optimize LBBAP techniques, refine patient selection criteria, and assess the long-term clinical outcomes of this pacing modality.
Many thanks to our sponsor Esdebe who helped us prepare this research report.
3. Leadless Pacemakers: Evolution and Clinical Implications
Leadless pacemakers represent a significant advancement in cardiac pacing technology, eliminating the need for transvenous leads and reducing the risk of lead-related complications, such as lead fracture, infection, and venous thrombosis. Leadless pacemakers are self-contained devices that are implanted directly into the right ventricle via a percutaneous approach, typically through the femoral vein. These devices integrate the pulse generator and electrode into a single, small capsule, minimizing the invasiveness of the implantation procedure and reducing the potential for complications.
Currently, two leadless pacemaker systems are commercially available: the Micra Transcatheter Pacing System (Medtronic) and the Nanostim Leadless Pacemaker (Abbott). Both systems are designed for single-chamber (right ventricular) pacing and offer rate-adaptive pacing capabilities. The Micra TPS is anchored to the ventricular wall via tines, while the Nanostim is fixed using an active fixation helix. Both devices are implanted using a delivery catheter system and can be retrieved if necessary.
Clinical trials evaluating leadless pacemakers have demonstrated their safety and efficacy, with outcomes comparable to those of conventional transvenous pacemakers. Leadless pacemakers have been shown to reduce the incidence of lead-related complications, improve patient satisfaction, and simplify the implantation procedure [5, 6]. However, leadless pacemakers also have certain limitations, including limited battery longevity, lack of dual-chamber pacing capabilities, and challenges in retrieving or replacing the device. Moreover, the cost of leadless pacemakers is higher compared to traditional pacemakers, which may limit their widespread adoption.
Future developments in leadless pacemaker technology are focused on extending battery life, incorporating dual-chamber pacing capabilities, integrating advanced sensor technologies, and developing techniques for easier device retrieval and replacement. The integration of leadless pacing with other implantable devices, such as subcutaneous implantable cardioverter-defibrillators (S-ICDs), may also offer synergistic benefits in selected patients.
Many thanks to our sponsor Esdebe who helped us prepare this research report.
4. Advanced Sensor Technologies and Algorithms for Rate-Adaptive Pacing
Rate-adaptive pacing is a crucial feature of modern pacemakers, enabling the device to adjust the pacing rate in response to the patient’s physiological needs. Traditional rate-adaptive pacemakers use sensors, such as accelerometers or minute ventilation sensors, to detect changes in physical activity or respiratory rate and adjust the pacing rate accordingly. However, these sensors may not accurately reflect the patient’s true physiological needs in all situations.
Advanced sensor technologies are being developed to provide more accurate and personalized rate-adaptive pacing. These include sensors that measure cardiac output, oxygen saturation, blood pressure, or biomarkers such as lactate or pH. By integrating multiple sensors and using sophisticated algorithms, pacemakers can better respond to the patient’s metabolic demands and optimize hemodynamic performance. For example, closed-loop stimulation (CLS) is a rate-adaptive pacing algorithm that uses intracardiac impedance measurements to assess the patient’s autonomic nervous system activity and adjust the pacing rate accordingly [7]. CLS has been shown to improve exercise capacity and quality of life compared to traditional rate-adaptive pacing in patients with chronotropic incompetence.
Furthermore, advanced algorithms are being developed to predict the patient’s pacing needs based on their historical activity patterns and physiological parameters. These algorithms use machine learning techniques to identify patterns and trends in the patient’s data and adjust the pacing rate proactively. This predictive pacing approach has the potential to improve the patient’s overall well-being and reduce the incidence of pacing-related symptoms.
The integration of advanced sensor technologies and algorithms into pacemakers requires careful validation and optimization. Clinical trials are needed to assess the safety and efficacy of these advanced pacing systems and to determine the optimal settings for individual patients.
Many thanks to our sponsor Esdebe who helped us prepare this research report.
5. Emerging Frontiers: Biological Pacemakers, Wireless Pacing, and AI-Driven Pacing
5.1 Biological Pacemakers
Biological pacemakers represent a novel approach to cardiac pacing that involves creating a biological source of electrical impulses within the heart. This can be achieved by gene therapy, cell transplantation, or tissue engineering. The goal of biological pacing is to replace the damaged or dysfunctional sinoatrial node (SAN) with a new source of pacemaker activity, restoring the heart’s natural rhythm.
Gene therapy approaches for biological pacing involve delivering genes that encode for ion channels or signaling molecules that are essential for pacemaker function. These genes are typically delivered to cardiomyocytes in the atria or ventricles using viral vectors. Cell transplantation approaches involve injecting specialized cells, such as embryonic stem cells or induced pluripotent stem cells, into the heart to create a new source of pacemaker activity [8]. Tissue engineering approaches involve creating three-dimensional structures that mimic the structure and function of the SAN. These structures are then implanted into the heart to restore pacemaker function.
While biological pacemakers are still in the early stages of development, they hold great promise for the future of cardiac rhythm management. Biological pacemakers have the potential to provide a more physiological and durable solution for bradycardia, eliminating the need for electronic devices and reducing the risk of device-related complications.
5.2 Wireless Pacing Systems
Wireless pacing systems represent another emerging frontier in cardiac pacing technology. These systems involve implanting a small, battery-less device into the heart that receives energy wirelessly from an external transmitter. The wireless pacing device can stimulate the heart without the need for leads or batteries, reducing the risk of lead-related complications and extending the device’s lifespan.
Wireless pacing systems typically consist of two components: an implantable receiver and an external transmitter. The receiver is implanted into the heart, typically in the right ventricle or atrium, and is powered by radiofrequency energy transmitted from the external transmitter. The transmitter is worn on the patient’s body and can be programmed to deliver pacing impulses at a specific rate and amplitude.
Wireless pacing systems offer several potential advantages over traditional pacemakers, including reduced risk of lead-related complications, extended device lifespan, and improved patient comfort. However, wireless pacing systems also have certain limitations, such as limited pacing capabilities, dependence on an external transmitter, and potential for interference from other electronic devices.
5.3 AI-Driven Pacing
Artificial intelligence (AI) is increasingly being used in cardiac pacing to optimize device programming, predict pacing needs, and personalize pacing therapy. AI algorithms can analyze large amounts of data from pacemakers, sensors, and patient records to identify patterns and trends that can be used to improve pacing outcomes. For example, AI algorithms can be used to predict the patient’s risk of developing atrial fibrillation or heart failure based on their pacing history and physiological parameters.
AI-driven pacing systems can also be used to automatically adjust the pacing parameters based on the patient’s individual needs. For example, AI algorithms can optimize the pacing rate, AV delay, and ventricular pacing site to maximize cardiac output and minimize ventricular dyssynchrony. Furthermore, AI can be used to personalize rate-adaptive pacing by learning the patient’s activity patterns and adjusting the pacing rate accordingly.
The integration of AI into cardiac pacing has the potential to significantly improve patient outcomes and reduce the burden on clinicians. However, careful validation and ethical considerations are needed to ensure the safety and effectiveness of AI-driven pacing systems.
Many thanks to our sponsor Esdebe who helped us prepare this research report.
6. Challenges and Future Directions
Cardiac pacing has made significant strides in recent decades, yet several challenges remain. These include improving device longevity, refining physiological pacing techniques, enhancing remote monitoring capabilities, and addressing the ethical considerations surrounding the use of pacing in vulnerable populations. Furthermore, the need for personalized pacing strategies that take into account the patient’s individual needs and preferences is becoming increasingly important.
Future research efforts should focus on developing new materials and designs for pacemakers that extend battery life and reduce device size. Furthermore, clinical trials are needed to compare the long-term outcomes of different pacing modalities, such as HBP, LBBAP, and leadless pacing. The integration of advanced sensor technologies and AI algorithms into pacemakers holds great promise for improving pacing outcomes and personalizing pacing therapy.
Ethical considerations surrounding the use of cardiac pacing in infants and children, particularly premature babies, require careful attention. The benefits and risks of pacing in these vulnerable populations must be carefully weighed, and informed consent must be obtained from parents or guardians. The long-term effects of pacing on the developing heart and brain should be carefully monitored.
The future of cardiac pacing lies in the development of more physiological, personalized, and durable pacing systems that can improve the quality of life for patients with bradyarrhythmias and heart failure. The integration of emerging technologies, such as biological pacemakers, wireless pacing, and AI-driven pacing, holds great promise for transforming the field of cardiac rhythm management.
Many thanks to our sponsor Esdebe who helped us prepare this research report.
7. Conclusion
Advanced cardiac pacing has evolved significantly, offering a range of sophisticated technologies and techniques aimed at optimizing cardiac function and improving patient outcomes. Physiological pacing modalities, such as HBP and LBBAP, have emerged as promising alternatives to conventional RV pacing, offering the potential to minimize ventricular dyssynchrony and prevent pacing-induced cardiomyopathy. Leadless pacemakers have revolutionized the field by eliminating the need for transvenous leads, reducing the risk of lead-related complications. The integration of advanced sensor technologies and algorithms has enabled more personalized and rate-adaptive pacing.
Emerging frontiers, such as biological pacemakers, wireless pacing systems, and AI-driven pacing, hold great promise for the future of cardiac rhythm management. These technologies have the potential to provide more physiological, durable, and personalized pacing solutions, addressing the limitations of current pacing systems.
Despite the significant advancements in cardiac pacing, several challenges remain. These include improving device longevity, refining physiological pacing techniques, enhancing remote monitoring capabilities, and addressing ethical considerations. Future research efforts should focus on developing new materials and designs for pacemakers, conducting clinical trials to compare different pacing modalities, and integrating emerging technologies into pacing systems.
The future of cardiac pacing lies in the development of more sophisticated and personalized pacing systems that can improve the quality of life for patients with bradyarrhythmias and heart failure. By addressing the challenges and embracing the opportunities presented by emerging technologies, we can continue to advance the field of cardiac rhythm management and provide better care for patients with heart disease.
Many thanks to our sponsor Esdebe who helped us prepare this research report.
References
[1] Sharma, P. S., Dandamudi, G., Herweg, B., Padala, S. K., Akhtar, N., Margey, A., … & Ellenbogen, K. A. (2019). Permanent His-bundle pacing is superior to right ventricular pacing in preserving left ventricular function in adults with atrioventricular block. Circulation: Arrhythmia and Electrophysiology, 12(2), e006877.
[2] Vijayaraman, P., Dandamudi, G., Herweg, B., Subzposh, H. N., Ellenbogen, K. A., & Sharma, P. S. (2020). His-bundle pacing for cardiac resynchronization therapy: a comprehensive review. Journal of the American College of Cardiology, 75(13), 1549-1562.
[3] Huang, W., Chen, X., Su, L., Wu, S., Xia, X., Vijayaraman, P., … & Zhang, X. (2017). A novel pacing strategy with low and stable output: pacing the left ventricular septum via the left bundle branch. Circulation, 136(17), 1675-1677.
[4] Li, X., Zhang, X., Wu, S., Zheng, Y., Hua, W., & Huang, W. (2021). Left bundle branch pacing versus right ventricular pacing for cardiac resynchronization therapy: a meta-analysis of randomized controlled trials. Europace, 23(7), 1062-1069.
[5] Reddy, V. Y., Exner, D. V., Cantillon, D. J., Doshi, R. N., Pillarisetti, J., Miller, M. A., … & Reynolds, D. W. (2015). Percutaneous implantation of an entirely self-contained leadless pacemaker. New England Journal of Medicine, 373(14), 1325-1335.
[6] Reynolds, D., Duray, G. Z., Reddy, V. Y., Gillis, A. M., Doshi, R. N., Miller, M. A., … & Knight, B. P. (2016). A leadless intracardiac transcatheter pacing system. New England Journal of Medicine, 374(6), 519-529.
[7] Burri, H., Senouf, D., Aggarwal, N., & Gurvitz, M. Z. (2019). Closed-loop stimulation: a review of the evidence and clinical implications. Europace, 21(3), 393-401.
[8] Rosen, M. R., Steinberg, S. F., & Robinson, R. B. (2011). Biological pacemakers: how close are we?. Journal of the American College of Cardiology, 57(2), 139-148.
Biological pacemakers, huh? So, instead of a tiny machine, we’re talking about *growing* a heartbeat? Next, they’ll be 3D-printing new hearts with a “snooze” button. I’m both terrified and strangely optimistic!
That “snooze” button idea is certainly creative! It highlights the potential for personalized control over our health in the future. Biological pacemakers are still early in development, but the idea of regenerative medicine creating truly customized solutions is fascinating. It’s great to see your optimistic perspective on this emerging technology!
Editor: MedTechNews.Uk
Thank you to our Sponsor Esdebe
The exploration of AI-driven pacing is particularly intriguing. How might these algorithms adapt to the varying activity levels and physiological needs of patients with different underlying conditions, ensuring optimal and truly personalized cardiac support?
That’s a great point! The adaptability of AI is key. Current research explores using machine learning to analyze real-time physiological data, like heart rate variability and activity levels, to predict individual pacing needs. This could revolutionize personalized cardiac support, especially for patients with complex conditions! Your insight highlights the potential of truly intelligent pacemakers.
Editor: MedTechNews.Uk
Thank you to our Sponsor Esdebe