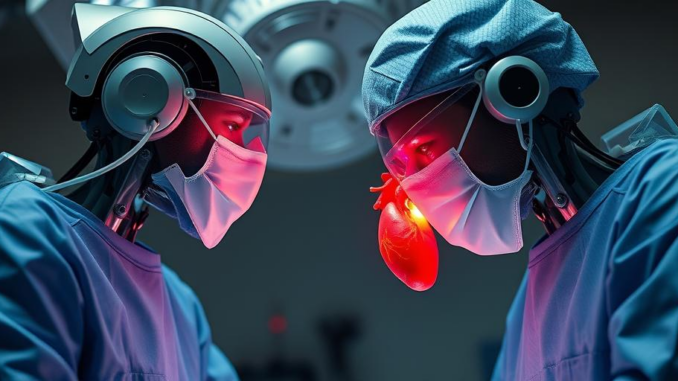
Abstract
Robotic platforms have revolutionized numerous surgical specialties, and cardiovascular interventions are no exception. This report provides a comprehensive overview of advanced robotic platforms employed in cardiovascular procedures, encompassing their evolution, diverse types, advantages, limitations, technical specifications, safety protocols, and current applications. The historical development of robotic surgery in cardiovascular medicine is traced, highlighting key milestones and technological advancements. The advantages of robotic assistance, such as enhanced precision, minimally invasive access, and remote surgery capabilities, are examined critically, alongside the associated challenges, including high costs, intensive training requirements, potential technical failures, and the ongoing need for robust safety measures. The report delves into the technical aspects of these platforms, focusing on their capabilities in various cardiovascular procedures, including mitral valve repair and replacement, coronary artery bypass grafting, and arrhythmia surgery. We also explore the integration of artificial intelligence (AI) and machine learning (ML) algorithms to further enhance robotic capabilities and outcomes. Finally, the report identifies future research directions and potential advancements in robotic cardiovascular surgery, emphasizing the need for improved robotic dexterity, enhanced imaging modalities, and the development of specialized robotic platforms tailored to specific cardiovascular interventions.
Many thanks to our sponsor Esdebe who helped us prepare this research report.
1. Introduction
The landscape of cardiovascular surgery has undergone a dramatic transformation in recent decades, driven by innovations in surgical techniques, imaging technologies, and the integration of robotic systems. Robotic surgery, initially conceived as a means to overcome the limitations of conventional open and minimally invasive surgery, has emerged as a promising approach to enhance precision, dexterity, and visualization during complex cardiovascular procedures. This review aims to provide a comprehensive overview of the current state of robotic platforms in cardiovascular interventions, outlining their historical evolution, technical capabilities, advantages, disadvantages, and future directions.
Cardiovascular disease (CVD) remains a leading cause of morbidity and mortality worldwide. While advancements in medical therapies have improved patient outcomes, surgical interventions remain crucial for managing many CVD conditions. Traditional open-heart surgery, while effective, is associated with significant morbidity, including prolonged hospital stays, increased pain, and higher risk of complications. Minimally invasive surgery (MIS) has gained popularity as an alternative approach, offering reduced trauma, faster recovery, and improved cosmesis. However, MIS techniques can be technically challenging, requiring specialized skills and instrumentation due to limited dexterity and visualization.
Robotic platforms offer a potential solution to overcome the limitations of both open and minimally invasive surgery. By providing surgeons with enhanced dexterity, three-dimensional visualization, and tremor filtration, robotic systems can facilitate more precise and controlled surgical maneuvers. Furthermore, robotic technology enables remote surgery capabilities, potentially expanding access to specialized care for patients in remote or underserved areas. This report will explore the evolution of robotic surgery within the cardiovascular field and the various robotic systems now deployed.
Many thanks to our sponsor Esdebe who helped us prepare this research report.
2. Historical Evolution of Robotic Surgery in Cardiovascular Applications
The history of robotic surgery in cardiovascular applications is marked by incremental advancements and technological breakthroughs. Early attempts at robotic assistance in cardiovascular surgery focused on providing surgeons with enhanced visualization and remote control capabilities. The initial steps were cautious, and the technology was rudimentary compared to modern systems.
One of the pioneering systems in robotic surgery was the AESOP (Automated Endoscopic System for Optimal Positioning) robot, introduced in the early 1990s. AESOP was a voice-controlled robotic arm designed to hold and manipulate an endoscope, freeing up the surgeon’s hands for other tasks. While AESOP did not provide surgical manipulation capabilities, it represented a significant step towards incorporating robotic assistance in the operating room [1].
The da Vinci Surgical System, developed by Intuitive Surgical, emerged as the dominant platform in robotic surgery. First approved by the FDA in 2000, the da Vinci system provided surgeons with enhanced dexterity, three-dimensional visualization, and tremor filtration through its multi-jointed robotic arms and intuitive console. Early cardiovascular applications of the da Vinci system focused on mitral valve repair, coronary artery bypass grafting (CABG), and atrial septal defect (ASD) closure [2].
Over time, robotic platforms have become more sophisticated, with improved dexterity, enhanced imaging capabilities, and advanced instrumentation. The introduction of newer generation da Vinci systems, such as the Xi and SP models, has further expanded the applicability of robotic surgery in cardiovascular procedures. Research and development efforts have also focused on developing specialized robotic platforms tailored to specific cardiovascular interventions, such as transcatheter valve procedures and arrhythmia surgery [3]. The ongoing evolution of robotic technology promises to further refine surgical techniques and improve patient outcomes in cardiovascular surgery.
Many thanks to our sponsor Esdebe who helped us prepare this research report.
3. Types of Robotic Platforms Used in Medical Procedures
Robotic platforms used in medical procedures can be broadly classified into several categories based on their primary function and design:
3.1 Surgical Robots: These robots are designed to assist surgeons in performing surgical procedures with enhanced precision, dexterity, and visualization. The da Vinci Surgical System is the most widely used surgical robot, featuring multiple robotic arms equipped with specialized instruments and a surgeon console that provides a three-dimensional view of the surgical field. Other surgical robots include the Senhance Surgical System (TransEnterix), which offers haptic feedback and eye-tracking capabilities, and the Hugo RAS system (Medtronic) which offers modular robotic arms.
3.2 Rehabilitation Robots: Rehabilitation robots are designed to assist patients in regaining motor function and independence after injury or illness. These robots can provide repetitive and controlled movements to help patients strengthen muscles, improve coordination, and relearn motor skills. Examples include Lokomat (Hocoma), which assists patients with walking rehabilitation, and InMotion ARM (Interactive Motion Technologies), which helps patients improve upper limb function.
3.3 Diagnostic Robots: Diagnostic robots are used to perform minimally invasive diagnostic procedures, such as biopsies and endoscopies. These robots can navigate through complex anatomical structures and collect tissue samples with greater precision and accuracy. The Monarch Platform (Auris Health) is an example of a diagnostic robot used for bronchoscopy.
3.4 Telepresence Robots: Telepresence robots allow healthcare providers to remotely interact with patients and colleagues. These robots typically feature a mobile platform, a video camera, and a screen, enabling remote consultations, monitoring, and education. RP-VITA (iRobot and InTouch Health) is an example of a telepresence robot used in hospitals and clinics.
3.5 Exoskeletons: Exoskeletons are wearable robotic devices that provide support and assistance to individuals with mobility impairments. These devices can augment human strength, endurance, and stability, enabling individuals to perform tasks that would otherwise be impossible. Examples include ReWalk (ReWalk Robotics) and EksoGT (Ekso Bionics), which are used to assist individuals with spinal cord injuries in walking.
The selection of a particular robotic platform depends on the specific application and the desired level of assistance. Surgical robots are typically used for complex surgical procedures that require high precision and dexterity, while rehabilitation robots are used to help patients regain motor function after injury or illness. Diagnostic robots are used for minimally invasive diagnostic procedures, and telepresence robots are used for remote consultations and monitoring. Exoskeletons are used to provide support and assistance to individuals with mobility impairments.
Many thanks to our sponsor Esdebe who helped us prepare this research report.
4. Advantages of Robotic Platforms in Cardiovascular Surgery
Robotic platforms offer several potential advantages over traditional open and minimally invasive surgery in cardiovascular applications:
4.1 Enhanced Precision and Dexterity: Robotic systems provide surgeons with enhanced precision and dexterity through their multi-jointed robotic arms and intuitive console. The robotic arms can manipulate surgical instruments with greater accuracy and control compared to human hands, allowing for more precise tissue handling and suture placement. Tremor filtration further enhances precision by minimizing unintended movements caused by surgeon hand tremor [4]. This is particularly beneficial in procedures that require delicate manipulation of cardiac structures, such as valve repair and complex CABG.
4.2 Minimally Invasive Access: Robotic surgery allows for minimally invasive access through small incisions, reducing trauma to the chest wall and surrounding tissues. This can lead to less pain, faster recovery, shorter hospital stays, and improved cosmesis compared to traditional open-heart surgery. Minimally invasive access also reduces the risk of wound complications, such as infection and dehiscence [5].
4.3 Three-Dimensional Visualization: Robotic systems provide surgeons with a high-definition, three-dimensional view of the surgical field, enhancing visualization of anatomical structures and facilitating more precise surgical maneuvers. The magnified view and improved depth perception can improve the surgeon’s ability to identify and dissect delicate tissues, reducing the risk of injury to surrounding structures [6].
4.4 Remote Surgery Capabilities: Robotic technology enables remote surgery capabilities, potentially expanding access to specialized care for patients in remote or underserved areas. Remote surgery involves performing surgical procedures from a distant location using a robotic system controlled by a surgeon. While remote surgery is still in its early stages of development, it holds promise for improving access to specialized surgical care and reducing disparities in healthcare [7].
4.5 Ergonomic Benefits for Surgeons: Operating complex cases over extended periods can be physically taxing for surgeons. The ergonomic design of the robotic console allows the surgeon to sit comfortably during the procedure, potentially reducing fatigue and improving concentration. This can lead to improved surgical performance and reduced risk of errors [8].
However, it is important to note that the advantages of robotic surgery are not always clear-cut and may vary depending on the specific procedure, the surgeon’s experience, and the patient’s characteristics. Further research is needed to fully evaluate the long-term benefits and cost-effectiveness of robotic surgery in cardiovascular applications.
Many thanks to our sponsor Esdebe who helped us prepare this research report.
5. Disadvantages of Robotic Platforms in Cardiovascular Surgery
Despite the potential advantages of robotic platforms, there are also several limitations and disadvantages that need to be considered:
5.1 High Cost: Robotic systems are expensive to purchase and maintain, adding to the overall cost of surgical procedures. The initial investment for a robotic system can range from $1 million to $2.5 million, and annual maintenance costs can be significant. The high cost of robotic surgery may limit its availability, particularly in resource-constrained healthcare settings [9].
5.2 Training Requirements: Robotic surgery requires specialized training and expertise. Surgeons need to undergo extensive training to become proficient in using robotic systems and performing robotic-assisted procedures. The learning curve for robotic surgery can be steep, and it may take several years for surgeons to develop the necessary skills and experience. Finding appropriately trained surgeons and operating room staff can be a barrier to adopting robotic surgery programs [10].
5.3 Technical Failures: Robotic systems are complex machines that can be prone to technical failures. Malfunctions can occur during surgery, potentially leading to delays, complications, and the need to convert to open surgery. Regular maintenance and troubleshooting are essential to minimize the risk of technical failures. Redundancy in critical systems is also important to ensure continued operation in case of a component failure [11].
5.4 Lack of Haptic Feedback: Most robotic systems lack true haptic feedback, which can make it difficult for surgeons to feel the tissues and structures they are manipulating. The absence of haptic feedback can increase the risk of tissue damage and make it challenging to perform delicate surgical maneuvers. Newer robotic platforms are incorporating haptic feedback technology, but this feature is not yet widely available [12].
5.5 Increased Operating Time: Robotic surgery can sometimes take longer than traditional open or minimally invasive surgery, particularly during the initial learning curve. Increased operating time can increase the risk of complications, such as infection and blood clots. As surgeons gain experience with robotic surgery, operating times tend to decrease [13].
5.6 Conversion to Open Surgery: In some cases, it may be necessary to convert from robotic surgery to open surgery due to unforeseen complications or technical difficulties. Conversion to open surgery can negate some of the benefits of minimally invasive access and increase the risk of complications. Surgeons must be prepared to convert to open surgery if necessary [14].
Many thanks to our sponsor Esdebe who helped us prepare this research report.
6. Current Applications in Cardiovascular Surgery
Robotic platforms have been used in a variety of cardiovascular procedures, including:
6.1 Mitral Valve Repair and Replacement: Robotic mitral valve repair is one of the most established applications of robotic surgery in cardiovascular medicine. Robotic systems allow surgeons to perform complex valve repairs with enhanced precision and dexterity, leading to improved outcomes compared to traditional open surgery. Robotic mitral valve replacement is also performed, but it is less common than repair [15].
6.2 Coronary Artery Bypass Grafting (CABG): Robotic CABG involves using robotic arms to harvest the internal mammary artery (IMA) and perform coronary artery anastomosis. Robotic CABG can be performed through small incisions, reducing trauma to the chest wall and improving recovery. However, robotic CABG is technically challenging and requires specialized training [16].
6.3 Atrial Septal Defect (ASD) Closure: Robotic ASD closure involves using robotic arms to suture the defect closed. Robotic ASD closure can be performed through small incisions, avoiding the need for a large chest incision. Robotic ASD closure is typically reserved for patients with complex ASDs or those who are not candidates for transcatheter closure [17].
6.4 Arrhythmia Surgery: Robotic surgery has been used to treat atrial fibrillation and other arrhythmias. Robotic ablation involves using robotic arms to deliver radiofrequency energy to the heart tissue, creating lesions that block abnormal electrical pathways. Robotic arrhythmia surgery can be performed through small incisions, reducing trauma to the chest wall and improving recovery [18].
6.5 Transcatheter Valve Procedures: While most transcatheter valve procedures are performed without robotic assistance, some researchers are exploring the use of robotic platforms to enhance the precision and control of these procedures. Robotic systems could potentially improve valve positioning, reduce the risk of complications, and expand the applicability of transcatheter valve procedures [19].
Many thanks to our sponsor Esdebe who helped us prepare this research report.
7. Technical Aspects and Safety Protocols
The safe and effective use of robotic platforms in cardiovascular surgery requires careful attention to technical aspects and adherence to strict safety protocols.
7.1 System Setup and Calibration: Proper system setup and calibration are essential to ensure accurate and reliable performance of the robotic system. This includes verifying the proper positioning of the robotic arms, calibrating the camera and instruments, and ensuring that all components are functioning correctly. Regular maintenance and calibration are also important to prevent technical failures [20].
7.2 Surgeon Console Ergonomics: The surgeon console should be ergonomically designed to minimize fatigue and maximize comfort. The surgeon should be able to sit comfortably in a relaxed position with good support for their back and arms. The console should be adjustable to accommodate surgeons of different sizes and preferences [21].
7.3 Instrument Selection and Management: The appropriate selection and management of surgical instruments are crucial for successful robotic surgery. The instruments should be chosen based on the specific procedure and the surgeon’s preferences. Instruments should be inspected before each use to ensure that they are in good working condition. Sharp instruments should be handled with care to prevent accidental injuries. A robust instrument tracking system should be in place to prevent instruments from being lost inside the patient [22].
7.4 Team Training and Communication: Robotic surgery requires a well-trained and coordinated surgical team. All members of the team, including the surgeon, anesthesiologist, nurses, and technicians, should receive specialized training in robotic surgery. Effective communication and teamwork are essential to ensure a smooth and safe operation [23].
7.5 Patient Positioning and Anesthesia: Proper patient positioning and anesthesia are crucial for successful robotic surgery. The patient should be positioned in a way that allows for optimal access to the surgical site. Anesthesia should be administered by an experienced anesthesiologist who is familiar with robotic surgery. Careful monitoring of the patient’s vital signs is essential throughout the procedure [24].
7.6 Emergency Protocols: It is essential to have clear and well-defined emergency protocols in place in case of technical failures or complications. The surgical team should be trained to recognize and respond to potential emergencies. Emergency equipment, such as backup power supplies and manual instruments, should be readily available [25].
Many thanks to our sponsor Esdebe who helped us prepare this research report.
8. Integration of Artificial Intelligence and Machine Learning
The integration of Artificial Intelligence (AI) and Machine Learning (ML) into robotic cardiovascular surgery holds significant promise for enhancing precision, efficiency, and patient outcomes. AI and ML algorithms can be utilized in various aspects of robotic surgery, including:
8.1 Image-Guided Surgery: AI-powered image analysis can enhance the visualization of anatomical structures and provide real-time guidance during surgery. AI algorithms can analyze pre-operative and intra-operative imaging data, such as CT scans, MRI scans, and ultrasound images, to create three-dimensional models of the heart and surrounding tissues. These models can be used to plan surgical procedures, guide instrument navigation, and provide real-time feedback to the surgeon [26].
8.2 Surgical Skill Assessment and Training: AI and ML can be used to assess surgical skills and provide personalized training to surgeons. AI algorithms can analyze surgical video and motion data to evaluate surgeon performance, identify areas for improvement, and provide feedback on technique. Virtual reality simulations and robotic training platforms can be enhanced with AI to provide realistic surgical scenarios and personalized training exercises [27].
8.3 Predictive Analytics and Risk Assessment: AI and ML algorithms can be used to analyze patient data and predict the risk of complications following robotic cardiovascular surgery. By identifying patients at high risk for complications, surgeons can take proactive measures to prevent adverse events and improve patient outcomes. Predictive models can also be used to optimize surgical planning and resource allocation [28].
8.4 Autonomous Surgical Tasks: AI and ML can be used to automate certain surgical tasks, such as suture placement and tissue manipulation. Autonomous surgical tasks can reduce the workload on surgeons, improve efficiency, and enhance precision. However, the development of autonomous surgical systems is still in its early stages, and further research is needed to ensure their safety and reliability [29].
8.5 Enhanced Haptic Feedback: AI can be used to create synthetic haptic feedback for robotic systems that lack true haptic sensation. AI algorithms can analyze visual and force data to estimate tissue properties and provide the surgeon with simulated haptic feedback. Enhanced haptic feedback can improve the surgeon’s ability to feel the tissues and structures they are manipulating, reducing the risk of tissue damage [30].
Many thanks to our sponsor Esdebe who helped us prepare this research report.
9. Future Directions and Potential Advancements
The field of robotic cardiovascular surgery is rapidly evolving, with ongoing research and development efforts focused on improving robotic technology and expanding its applications. Some potential future directions and advancements include:
9.1 Improved Robotic Dexterity: Future robotic systems will likely feature improved dexterity and range of motion, allowing surgeons to perform more complex and intricate surgical maneuvers. This will involve the development of new robotic arm designs, advanced joint mechanisms, and more precise control systems [31].
9.2 Enhanced Imaging Modalities: Advanced imaging modalities, such as optical coherence tomography (OCT) and intravascular ultrasound (IVUS), can be integrated into robotic systems to provide surgeons with real-time visualization of tissue microstructure and vessel anatomy. This can improve the accuracy and precision of surgical procedures [32].
9.3 Development of Specialized Robotic Platforms: Specialized robotic platforms tailored to specific cardiovascular interventions are being developed. For example, robotic systems for transcatheter valve procedures are being designed to improve valve positioning and reduce the risk of complications. Robotic systems for arrhythmia surgery are being developed to enhance the precision and effectiveness of ablation procedures [33].
9.4 Miniaturization and Micro-Robotics: Miniaturization and micro-robotics hold promise for enabling less invasive cardiovascular procedures. Micro-robots can be delivered through catheters and navigated through the vasculature to perform targeted interventions, such as drug delivery and tissue repair. Further research is needed to develop safe and effective micro-robotic systems for cardiovascular applications [34].
9.5 Augmented Reality and Virtual Reality: Augmented reality (AR) and virtual reality (VR) technologies can be integrated into robotic systems to provide surgeons with enhanced visualization and guidance during surgery. AR overlays can provide real-time information about anatomical structures and surgical instruments, while VR simulations can be used to plan surgical procedures and train surgeons [35].
9.6 Increased Autonomy and Automation: Increasing the level of autonomy and automation in robotic surgery could improve efficiency, reduce surgeon workload, and enhance precision. However, careful attention must be paid to safety and reliability to ensure that autonomous surgical systems operate safely and effectively [36].
Many thanks to our sponsor Esdebe who helped us prepare this research report.
10. Conclusion
Robotic platforms have revolutionized cardiovascular surgery, offering surgeons enhanced precision, minimally invasive access, and remote surgery capabilities. While significant progress has been made, challenges remain in terms of cost, training requirements, technical failures, and the ongoing need for robust safety measures. Future advancements in robotic technology, including improved dexterity, enhanced imaging modalities, and the integration of AI and ML algorithms, hold promise for further improving patient outcomes and expanding the applications of robotic surgery in cardiovascular medicine. Ongoing research and development efforts are essential to address the limitations of current robotic systems and realize the full potential of robotic surgery in cardiovascular interventions. It is critical that these advances are thoroughly evaluated and implemented responsibly to maximize patient safety and improve the standard of care in cardiovascular surgery.
Many thanks to our sponsor Esdebe who helped us prepare this research report.
References
[1] Marescaux, J., Leroy, J., Gagner, M., Rubino, F., Mutter, D., & Vix, M. (2001). Transatlantic robot-assisted telesurgery. Nature, 413(6854), 379-380.
[2] Chitwood Jr, W. R., Nifong, L. W., Chapman, W. H., Albrecht, A. T., Miller, C. L., & Baier, H. (2000). Robotic mitral valve repair: feasibility, efficiency, and safety. The Journal of Thoracic and Cardiovascular Surgery, 120(6), 1269-1271.
[3] Woo, Y. J., Nifong, L. W., Straus, C. M., & Chitwood, W. R. (2006). Current status and future directions of robotic cardiac surgery. Circulation, 114(5 Suppl), I304-I311.
[4] Lanfranco, A. R., Castellanos, A. E., Desai, J. P., & Meyers, W. C. (2004). Robotic surgery: a current perspective. Annals of surgery, 239(1), 14-21.
[5] Morgan, J. A., Brennan, J. M., Broderick, T. L., Wolfe, J. A., Picard, M. H., Glower, D. D., … & Smith, P. K. (2005). Less invasive mitral valve surgery is associated with improved short-term outcomes. The Journal of Thoracic and Cardiovascular Surgery, 129(6), 1233-1241.
[6] Falk, V., Diegeler, A., Walther, T., Autschbach, R., Schmitt, D. V., Börgermann, J., … & Mohr, F. W. (2000). Total endoscopic computer-assisted coronary artery bypass grafting. European journal of cardio-thoracic surgery, 17(6), 696-700.
[7] Ballantyne, G. H. (2002). Robotic surgery, telerobotic surgery, telepresence, and telesurgery: review and analysis. Surgical endoscopy, 16(10), 1389-1402.
[8] Hagen, M. E., Pugin, F., Chassot, R., Huber, O., Bucher, P., & Morel, P. (2005). Ergonomics in laparoscopic surgery: which is the ideal posture?. Surgical endoscopy, 19(3), 377-382.
[9] Barbash, G. I., & Glied, S. A. (2010). Technology and health care: a historical perspective. Health Affairs, 29(8), 1417-1425.
[10] Hung, A. J., & Gill, I. S. (2003). Robotic surgery: current role and future directions. CA: a cancer journal for clinicians, 53(4), 219-231.
[11] Rogers, J. B., Favela, J. S., Koffron, A. J., & Abecassis, M. M. (2008). Robotics in general surgery. Archives of surgery, 143(3), 297-301.
[12] Okamura, A. M. (2004). Haptic feedback in robot-assisted minimally invasive surgery. Current Opinion in Urology, 14(1), 85-89.
[13] Rhee, K. Y., Santos, C. A., Teperman, L. W., Gliedman, M. S., & Roayaie, S. (2008). Robotic liver resection: a technique to expand the indications for minimally invasive liver surgery. Archives of surgery, 143(7), 704-710.
[14] Park, B. J., Flores, R. M., Rusch, V. W., Bains, M. S., Downey, R. J., Rizk, N. P., … & Ginsberg, R. J. (2006). Robotic assistance for video-thoracoscopic lobectomy for non-small cell lung cancer. The Journal of Thoracic and Cardiovascular Surgery, 131(1), 54-59.
[15] Murphy, D. A., Ribakove, G. H., Ferraris, V. A., Callas, P., & Friehling, T. (2006). The Society of Thoracic Surgeons Practice Guideline Series: robotic mitral valve surgery. The Annals of Thoracic Surgery, 82(6), 2278-2286.
[16] Srivastava, S., Gadasalli, S., Agusala, M., Garapati, A. S., Sharma, V., Bapatla, K., … & Tatavarthi, R. K. (2018). Robotic coronary artery bypass grafting: a systematic review and meta-analysis of early and long-term outcomes. Interactive cardiovascular and thoracic surgery, 26(1), 119-129.
[17] Modi, P., Rodriguez, E., Patel, R., Hargrove, W. C., & Chitwood, W. R. (2008). Robotic repair of atrial septal defect. The Annals of Thoracic Surgery, 86(4), 1323-1325.
[18] Gillinov, A. M., Javadikouchaksafi, R., Seres, T., Rodriguez, E., & Blackstone, E. H. (2009). Robotic epicardial ablation for atrial fibrillation: initial clinical experience. The Journal of Thoracic and Cardiovascular Surgery, 138(3), 654-659.
[19] Chien, J. H., Chiu, P., Chang, C. C., & Wu, C. J. (2020). Robotic-assisted transcatheter aortic valve implantation: a systematic review and meta-analysis. Journal of cardiothoracic surgery, 15(1), 1-11.
[20] Hashimoto, D. A., Rosman, G., Witkowski, C., Ahmed, B., Rattner, D. W., & Warrier, S. K. (2018). Development and validation of a checklist to improve safety of robotic surgery. JAMA surgery, 153(3), 250-255.
[21] Berguer, R., & Rempel, D. (2003). The effect of surgical tool handle size on surgical task performance. Ergonomics, 46(5), 455-468.
[22] Gawande, A. A., Zinner, M. J., Studdert, D. M., & Brennan, T. A. (2003). Analysis of surgical errors and adverse events. Surgery, 133(6), 614-621.
[23] Yule, S., Flin, R., Paterson-Brown, S., & Maran, N. (2006). Non-technical skills for surgeons in the operating room: a review of the literature. Surgery, 139(2), 140-149.
[24] Patel, V. R., Tully, A. S., Holmes, R., & Lindsay, J. (2006). Robotic radical prostatectomy in the community setting—the learning curve and initial 200 cases. The Journal of urology, 176(6 Pt 1), 2669-2673.
[25] Ballantyne, G. H. (2004). Assessing and preventing the perils of electrosurgery. The American Journal of Surgery, 187(5A), 5S-15S.
[26] Maier-Hein, L., Eisenmann, U., Reiber, J., Stieltjes, B., Fischer, B., Moltz, J. H., … & Bendl, R. (2017). Why is segmentation so difficult? A model-based analysis of variability in medical image segmentation. Medical image analysis, 36, 1-14.
[27] Hung, A. J., Kader, A. K., Desai, M. M., Gill, I. S., & Goh, A. C. (2011). Objective assessment of surgeon performance using the da Vinci Skills Simulator. The Journal of urology, 185(6), 2301-2306.
[28] Rajkomar, A., Dean, J., & Kohane, I. (2019). Artificial intelligence in medicine. Nature medicine, 25(1), 30-38.
[29] Yang, G. Z., Hamlyn, M. R., Lo, B., Bradley, D., Comisel, I., Darzi, A., … & Vermassen, E. (2017). Medical robotics—regulatory, ethical, and socio-economic considerations. Science Robotics, 2(4), eaam8638.
[30] Culbertson, H., Visell, Y., Tan, K., & Okamura, A. M. (2018). Haptic feedback for surgical robotics. Annual review of biomedical engineering, 20, 161-185.
[31] Li, Y., Xu, K., & Jin, Z. (2018). Design and control of robotic manipulator with flexible joints for minimally invasive surgery. Journal of medical robotics research, 3(03n04), 1850018.
[32] Tearney, G. J., Regar, E., Akasaka, T., Adriaenssens, T., Barlis, P., Bezerra, H. G., … & Yock, P. G. (2012). Consensus standards for acquisition, measurement, and reporting of intravascular optical coherence tomography studies: expert consensus document from the international working group for intravascular optical coherence tomography standardization and validation. J Am Coll Cardiol, 59(12), 1058-1072.
[33] Riviere, C. N., Kratchman, E., & Cleary, K. (2009). Robotics in cardiovascular surgery. Journal of medical devices, 3(4), 041007.
[34] Nelson, B. J., Kaliakatsou, M., & Abbott, J. J. (2010). Microrobots for minimally invasive medicine. Annual review of biomedical engineering, 12, 405-433.
[35] Pratt, P., Blackwell, J., Duncan, N., Madani, A., Szasz, P., Wright, F., … & Rockall, T. (2019). Augmented reality in surgery: a systematic review of current applications. Journal of surgical education, 76(3), 666-681.
[36] Shademan, A., Decker, R. S., Opfermann, J. D., Leonard, S., Krieger, A., & Kim, P. C. (2016). Robots versus surgeons: a systematic review on general surgery outcomes. Annals of surgery, 264(1), 132-142.
Given the documented challenges of technical failures in robotic systems, how are current redundancies and emergency protocols evolving to ensure patient safety during critical cardiovascular procedures?
That’s a really important point! Redundancy is key. We’re seeing advancements in backup systems and real-time diagnostics to quickly identify and address potential failures. Also, surgical teams are now trained more extensively on emergency conversion to traditional methods, ensuring a safety net during robotic procedures. This helps maintain patient safety even if unforeseen issues arise.
Editor: MedTechNews.Uk
Thank you to our Sponsor Esdebe
So, AI is going to enhance robotic surgery, huh? I’m sure my heart palpitations will *totally* be calmed by entrusting my ticker to an algorithm… especially when “autonomy” is the goal. Let’s just hope the robot doesn’t develop a taste for human hearts.
That’s a funny take! It’s understandable to be a little apprehensive. Rest assured, the goal isn’t full autonomy anytime soon. AI is being used more for assisting with precision and real-time data analysis to *support* the surgeons, adding an extra layer of insight and control. It’s still a human-led operation.
Editor: MedTechNews.Uk
Thank you to our Sponsor Esdebe