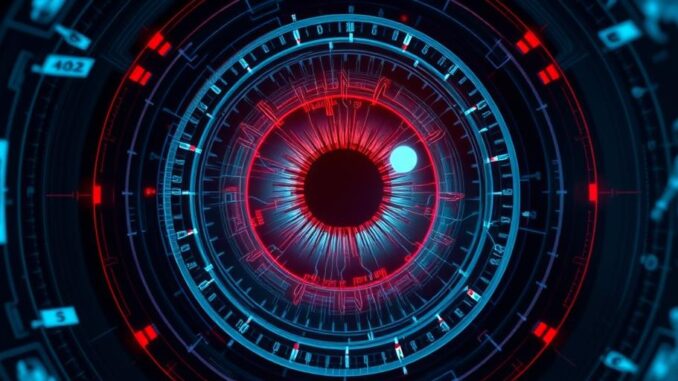
Advancements and Emerging Applications of Electroretinography: A Comprehensive Review
Many thanks to our sponsor Esdebe who helped us prepare this research report.
Abstract
Electroretinography (ERG) has evolved from a basic diagnostic tool for retinal disorders into a sophisticated technique capable of providing detailed insights into retinal function. This review examines the current state-of-the-art ERG, exploring advancements in technology, expanding applications beyond traditional retinal diseases, and addressing existing limitations. We delve into the nuances of full-field ERG, multifocal ERG (mfERG), pattern ERG (PERG), and photopic negative response (PhNR), highlighting their specific roles in assessing different retinal cell populations and functional pathways. Emerging applications in neuro-ophthalmology, drug development, gene therapy monitoring, and personalized medicine are discussed. The report also considers the challenges associated with ERG, including standardization issues, inter-subject variability, and the need for improved analytical methods. Finally, we offer perspectives on future directions, emphasizing the potential of ERG to contribute to a deeper understanding of retinal physiology and to improve patient outcomes.
Many thanks to our sponsor Esdebe who helped us prepare this research report.
1. Introduction
Electroretinography (ERG) is a non-invasive electrophysiological test that measures the electrical activity of the retina in response to light stimulation. Since its inception in the mid-20th century, ERG has become a cornerstone in the diagnosis and management of various retinal disorders. Originally conceived as a method to broadly assess retinal health, the technique has undergone significant refinements, leading to the development of diverse ERG protocols designed to isolate and evaluate specific retinal cell types and functional pathways. This evolution reflects both technological advancements in stimulus delivery and recording equipment, as well as an improved understanding of retinal neurophysiology.
The traditional full-field ERG (ffERG), which records the summed electrical response of the entire retina, remains a widely used method for detecting generalized retinal dysfunction. However, newer techniques such as multifocal ERG (mfERG) and pattern ERG (PERG) provide more localized and specific information about retinal function. mfERG enables the assessment of regional retinal activity, making it particularly useful for identifying localized retinal lesions and monitoring the progression of macular diseases. PERG, on the other hand, primarily reflects the function of retinal ganglion cells (RGCs) and their axons, providing valuable insights into optic nerve and inner retinal disorders.
This review aims to provide a comprehensive overview of the current landscape of ERG, covering its various methodologies, applications, limitations, and future directions. We will discuss the principles underlying each ERG technique, the interpretation of their respective waveforms, and their clinical utility in diagnosing and monitoring a wide range of retinal and neurological disorders. Furthermore, we will explore the emerging applications of ERG in drug development, gene therapy, and personalized medicine, highlighting its potential to contribute to a more targeted and effective approach to ophthalmic care.
Many thanks to our sponsor Esdebe who helped us prepare this research report.
2. ERG Methodologies: A Comparative Analysis
2.1 Full-Field ERG (ffERG)
The ffERG measures the global electrical response of the retina to a brief, intense flash of light. The resulting waveform consists of several components, each reflecting the activity of different retinal cell populations. The most prominent features are the a-wave, which primarily represents the activity of photoreceptors, and the b-wave, which is generated by the activity of bipolar cells and Müller cells. By analyzing the amplitude and timing of these waves, clinicians can assess the overall health and function of the outer and inner retina.
The International Society for Clinical Electrophysiology of Vision (ISCEV) has established standardized protocols for ffERG, ensuring consistency and comparability across different laboratories and studies [1]. These protocols include various stimulus conditions, such as dark-adapted (scotopic) and light-adapted (photopic) recordings, as well as responses to different flash intensities and flicker frequencies. The scotopic ffERG provides information about rod photoreceptor function, while the photopic ffERG primarily reflects cone photoreceptor activity. By comparing the responses under different conditions, clinicians can differentiate between various types of retinal disorders, such as rod-cone dystrophies and cone-rod dystrophies.
2.2 Multifocal ERG (mfERG)
The mfERG is a technique that allows for the simultaneous recording of electrical responses from multiple regions of the retina [2]. This is achieved by presenting a stimulus consisting of multiple small, independently flickering elements, typically arranged in a hexagonal pattern. The responses from each element are then analyzed separately, providing a topographical map of retinal function. The mfERG is particularly useful for assessing localized retinal dysfunction, such as that seen in macular degeneration, diabetic retinopathy, and drug-induced retinal toxicity.
The mfERG response consists of a series of waveforms, the most prominent of which is the first-order kernel response. This response is thought to reflect the activity of photoreceptors, bipolar cells, and retinal ganglion cells in the corresponding retinal region. By analyzing the amplitude and implicit time of the first-order kernel response, clinicians can identify areas of reduced retinal function and monitor the progression of retinal diseases. The mfERG is also valuable for detecting subtle retinal changes that may not be apparent on ffERG.
2.3 Pattern ERG (PERG)
The PERG is a specialized ERG technique that measures the electrical activity of the retina in response to a reversing checkerboard or grating pattern [3]. Unlike ffERG, which primarily reflects the activity of photoreceptors and bipolar cells, PERG primarily reflects the function of retinal ganglion cells (RGCs) and their axons. The PERG response consists of two main components: the N95 wave, which is thought to originate from RGCs, and the P50 wave, which may reflect the activity of amacrine cells and other inner retinal neurons. By analyzing the amplitude and timing of these waves, clinicians can assess the health and function of the inner retina and optic nerve.
The PERG is particularly useful for diagnosing and monitoring optic nerve disorders, such as glaucoma and optic neuritis. In these conditions, the amplitude of the N95 wave is typically reduced, reflecting the loss or dysfunction of RGCs. The PERG can also be used to detect early signs of inner retinal dysfunction in patients with diabetes, multiple sclerosis, and other neurological disorders. Moreover, PERG is proving useful in assessing the efficacy of neuroprotective therapies aimed at preserving RGC function.
2.4 Photopic Negative Response (PhNR)
The photopic negative response (PhNR) is a slow negative-going potential that follows the b-wave in the photopic ffERG [4]. It is thought to originate from the activity of retinal ganglion cells and their axons, as well as from amacrine cells. The PhNR is particularly sensitive to inner retinal dysfunction and has been shown to be reduced in amplitude in patients with glaucoma, diabetic retinopathy, and other disorders affecting RGCs. The PhNR is typically measured from the baseline to the trough of the negative-going potential following the b-wave peak.
The PhNR is a valuable tool for detecting early signs of inner retinal dysfunction, even before visual field defects are apparent. It can also be used to monitor the progression of glaucoma and other optic nerve disorders, as well as to assess the efficacy of neuroprotective therapies. While the PhNR holds significant promise as a diagnostic and monitoring tool, further research is needed to fully elucidate its cellular origins and to standardize its measurement and interpretation.
Many thanks to our sponsor Esdebe who helped us prepare this research report.
3. Applications of ERG in Diagnosing Retinal and Neurological Disorders
3.1 Retinal Degenerations
ERG is indispensable in the diagnosis and characterization of inherited retinal degenerations (IRDs) such as retinitis pigmentosa (RP) and cone-rod dystrophies (CRD). ffERG can reveal characteristic patterns of retinal dysfunction, such as reduced or absent a- and b-waves, depending on the specific gene mutation and the stage of the disease. In RP, for example, the scotopic ffERG is typically more severely affected than the photopic ffERG, reflecting the primary involvement of rod photoreceptors. In CRD, the photopic ffERG is often more severely affected, indicating cone photoreceptor dysfunction. ERG can also help differentiate between different types of IRDs, such as Stargardt disease and Best disease, which have distinct ERG patterns [5].
3.2 Diabetic Retinopathy
ERG can be used to detect early signs of diabetic retinopathy, even before microvascular changes are visible on fundus examination. Studies have shown that PERG and PhNR amplitudes are reduced in patients with diabetes, reflecting inner retinal dysfunction. These findings suggest that RGCs and other inner retinal neurons are vulnerable to the effects of hyperglycemia and other metabolic abnormalities associated with diabetes. ERG can also be used to monitor the progression of diabetic retinopathy and to assess the efficacy of treatments aimed at preventing or slowing retinal damage.
3.3 Glaucoma
PERG and PhNR are valuable tools for diagnosing and monitoring glaucoma, a leading cause of irreversible blindness worldwide. In glaucoma, RGCs are selectively damaged, leading to a reduction in PERG and PhNR amplitudes. These changes can occur even before visual field defects are apparent, making PERG and PhNR potentially useful for early detection of glaucoma. ERG can also be used to monitor the progression of glaucoma and to assess the efficacy of intraocular pressure-lowering medications and other treatments [6].
3.4 Drug-Induced Retinal Toxicity
Certain drugs, such as hydroxychloroquine (Plaquenil), can cause retinal toxicity, leading to visual impairment. ERG is a sensitive method for detecting early signs of drug-induced retinal toxicity, even before symptoms develop. In hydroxychloroquine toxicity, for example, mfERG can reveal localized areas of reduced retinal function, particularly in the parafoveal region. ERG can also be used to monitor the progression of drug-induced retinal toxicity and to assess the efficacy of interventions, such as drug discontinuation, aimed at preventing further retinal damage [7].
3.5 Neurological Disorders
ERG has emerged as a valuable tool for investigating the retinal manifestations of neurological disorders. For example, studies have shown that ERG abnormalities are common in patients with multiple sclerosis (MS), Parkinson’s disease (PD), and Alzheimer’s disease (AD). In MS, PERG amplitude is often reduced, reflecting optic nerve dysfunction. In PD, both ffERG and PERG abnormalities have been reported, suggesting dysfunction of both the outer and inner retina. In AD, ERG abnormalities have been linked to amyloid deposition in the retina. These findings suggest that ERG may provide valuable insights into the pathophysiology of neurological disorders and may serve as a biomarker for disease progression or treatment response [8].
Many thanks to our sponsor Esdebe who helped us prepare this research report.
4. Emerging Applications and Future Directions
4.1 Gene Therapy Monitoring
ERG plays a crucial role in monitoring the efficacy of gene therapy for inherited retinal degenerations. In gene therapy trials, ERG is used to assess the functional improvement of the retina following gene delivery. For example, in patients with RPE65-related retinal dystrophy who have undergone gene therapy, ERG is used to track the restoration of retinal function and to evaluate the long-term safety and efficacy of the treatment [9].
4.2 Personalized Medicine
ERG holds promise for personalized medicine in ophthalmology. By combining ERG data with genetic information and other clinical findings, clinicians can tailor treatment strategies to individual patients. For example, in patients with inherited retinal degenerations, ERG can help identify the specific gene mutation responsible for the disease, which can then guide the selection of appropriate gene therapy or other targeted treatments.
4.3 Artificial Intelligence (AI) and Machine Learning
The application of AI and machine learning to ERG data analysis is an emerging area of research. AI algorithms can be trained to automatically analyze ERG waveforms, identify subtle abnormalities, and predict disease progression. This could lead to more efficient and accurate diagnosis and monitoring of retinal disorders [10]. AI can also be used to develop personalized treatment strategies based on ERG data and other clinical information.
4.4 Enhanced ERG Techniques
Ongoing research is focused on developing enhanced ERG techniques that can provide more detailed information about retinal function. For example, researchers are exploring the use of high-density mfERG to map retinal function with greater spatial resolution. They are also developing new ERG protocols that can selectively stimulate different retinal cell populations, such as amacrine cells and horizontal cells. These advancements will further expand the clinical utility of ERG and improve our understanding of retinal physiology.
Many thanks to our sponsor Esdebe who helped us prepare this research report.
5. Limitations and Challenges
Despite its numerous advantages, ERG has several limitations and challenges that need to be addressed. One major challenge is the standardization of ERG protocols. While ISCEV guidelines exist, there is still variability in the way ERG is performed and interpreted across different laboratories. This can lead to inconsistencies in results and make it difficult to compare data across studies. Efforts are needed to further standardize ERG protocols and to develop consensus guidelines for data interpretation.
Another challenge is the inter-subject variability in ERG responses. Even in healthy individuals, there can be significant variations in ERG amplitudes and timing. This variability can be influenced by factors such as age, refractive error, and pupil size. It is important to take these factors into account when interpreting ERG results and to compare results to age-matched normative data.
Finally, the interpretation of ERG waveforms can be complex and requires specialized expertise. The ERG response is influenced by multiple retinal cell populations and functional pathways, and it can be difficult to isolate the contribution of specific cell types. Further research is needed to better understand the cellular origins of ERG waveforms and to develop more sophisticated analytical methods.
Many thanks to our sponsor Esdebe who helped us prepare this research report.
6. Conclusion
Electroretinography remains a vital tool for assessing retinal function in a wide range of clinical and research settings. The evolution of ERG methodologies, from the traditional ffERG to the more specialized mfERG, PERG, and PhNR, has significantly expanded its diagnostic capabilities. Emerging applications in gene therapy monitoring, personalized medicine, and the integration of AI and machine learning hold great promise for the future of ERG. However, challenges related to standardization, inter-subject variability, and data interpretation need to be addressed to further optimize the clinical utility of this valuable technique. Ultimately, continued advancements in ERG technology and a deeper understanding of retinal neurophysiology will pave the way for improved diagnosis, monitoring, and treatment of retinal and neurological disorders.
Many thanks to our sponsor Esdebe who helped us prepare this research report.
References
[1] Marmor, M. F., Fulton, A. B., Holder, G. E., Straatsma, B. R., Thompson, J. T., & International Society for Clinical Electrophysiology of Vision. (2011). ISCEV Standard for full-field clinical electroretinography (2008 update). Documenta Ophthalmologica, 122(1), 1-14.
[2] Hood, D. C. (2000). Assessing retinal function with the multifocal electroretinogram. Progress in Retinal and Eye Research, 19(5), 607-646.
[3] Bach, M., Hawlina, M., Holder, G. E., Marmor, M. F., Meigen, T., Vaegan, & International Society for Clinical Electrophysiology of Vision. (2013). Standard for pattern electroretinography. Documenta Ophthalmologica, 126(1), 1-11.
[4] Viswanathan, S., Dickinson, J. E., & Keating, D. (2007). The photopic negative response (PhNR) of the flash electroretinogram: its origins and clinical utility. Clinical & Experimental Ophthalmology, 35(8), 754-764.
[5] Birch, D. G., & Anderson, J. L. (2008). Standardized full-field electroretinography: interpretation and clinical applications. Ophthalmology Clinics of North America, 21(4), 533-544.
[6] Ventura, L. M., Porciatti, V., & Feuer, W. J. (2011). Pattern electroretinogram (PERG) in early glaucoma: meta-analysis of diagnostic accuracy. Eye, 25(2), 145-153.
[7] Marmor, M. F., Kellner, U., Lai, T. Y. Y., Melles, R. B., & Weber, A. L. (2016). Recommendations on Screening for Chloroquine and Hydroxychloroquine Retinopathy (2016 Revision). Ophthalmology, 123(6), 1386-1394.
[8] Parisi, V., Parisi, L., Suppa, A., et al. (2017). Electrophysiological assessment of retinal and cortical function in Parkinson’s disease patients. Brain, 140(1), 30-40.
[9] Maguire, A. M., Simonelli, F., Pierce, E. A., et al. (2008). Safety and efficacy of gene transfer for Leber’s congenital amaurosis. New England Journal of Medicine, 358(21), 2240-2248.
[10] Liu, J., Li, S., Wu, S., et al. (2020). Deep learning for automated electroretinogram analysis: a systematic review. Translational Vision Science & Technology, 9(13), 3.
The review’s discussion of personalized medicine using ERG is compelling. Combining ERG data with genetic information could revolutionize treatment strategies for inherited retinal degenerations, offering more targeted and effective interventions.
Thanks for highlighting the personalized medicine aspect! The potential for combining ERG with genetic data is very exciting. Imagine tailoring therapies based on an individual’s retinal function and genetic makeup. It could really change how we approach inherited retinal diseases and improve patient outcomes in the future.
Editor: MedTechNews.Uk
Thank you to our Sponsor Esdebe
The discussion of limitations is important. Standardizing ERG protocols and analysis across different labs would greatly enhance the reliability and comparability of results, fostering collaborative research and more consistent clinical applications.