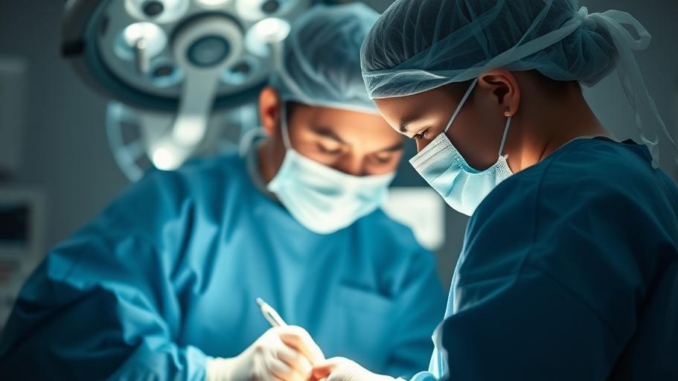
Abstract
Pediatric surgery, a rapidly evolving field, demands continuous innovation to address the unique anatomical, physiological, and psychological needs of young patients. This review examines the multifaceted advancements reshaping pediatric surgical practice, moving beyond a narrow focus on Enhanced Recovery After Surgery (ERAS) protocols to encompass a broader perspective. The report explores developments in minimally invasive surgery (MIS), robotic-assisted surgery (RAS), 3D printing and surgical simulation, fetal surgery, regenerative medicine, and the integration of artificial intelligence (AI) and machine learning (ML). Furthermore, it critically assesses the challenges associated with these advancements, including the ethical considerations, cost-effectiveness, and the need for specialized training. This review aims to provide a comprehensive overview of the current landscape and to identify key areas for future research and development, ultimately contributing to improved outcomes and quality of life for pediatric surgical patients.
Many thanks to our sponsor Esdebe who helped us prepare this research report.
1. Introduction
Pediatric surgery is a specialized surgical discipline dedicated to the diagnosis, treatment, and management of surgical conditions in infants, children, and adolescents. Unlike adult surgery, pediatric surgery necessitates a deep understanding of developmental biology, physiology, and the unique disease processes affecting the pediatric population. Over the past few decades, the field has witnessed remarkable progress, driven by technological innovation, improved understanding of pediatric pathophysiology, and a growing emphasis on patient-centered care. While Enhanced Recovery After Surgery (ERAS) protocols have undoubtedly contributed to improved patient outcomes, a holistic view of advancements in techniques, technologies, and translational research provides a more complete picture of the field’s evolution.
This review aims to comprehensively examine these advancements, moving beyond the immediate postoperative period. We will explore emerging surgical techniques, including advanced MIS and RAS, as well as innovative technologies like 3D printing and surgical simulation. We will also delve into the rapidly developing fields of fetal surgery and regenerative medicine, examining their potential to address complex congenital anomalies and promote tissue regeneration. Finally, we will discuss the integration of AI and ML into pediatric surgical practice, assessing their role in improving diagnostic accuracy, surgical planning, and postoperative monitoring. This review will not only highlight the successes of these advancements but also critically evaluate the associated challenges, including ethical considerations, cost-effectiveness, and the need for specialized training.
Many thanks to our sponsor Esdebe who helped us prepare this research report.
2. Minimally Invasive Surgery (MIS) and Robotic-Assisted Surgery (RAS)
2.1 Advancements in MIS
Minimally invasive surgery (MIS) has revolutionized pediatric surgical practice, offering significant advantages over traditional open surgery, including smaller incisions, reduced pain, shorter hospital stays, and improved cosmesis [1]. Technological advancements in instrumentation, imaging, and energy sources have further expanded the applicability of MIS across various pediatric surgical subspecialties.
Laparoscopy, thoracoscopy, and retroperitoneoscopy are now standard approaches for many common pediatric surgical procedures, such as appendectomy, cholecystectomy, fundoplication, and nephrectomy [2]. The development of smaller-diameter instruments and specialized pediatric trocars has facilitated MIS in even the smallest infants and neonates. Advanced imaging techniques, such as intraoperative ultrasound and fluorescence imaging, have improved visualization and precision during MIS procedures.
Opinion: The adoption of single-incision laparoscopic surgery (SILS) represents a further refinement of MIS. While SILS offers the potential for even better cosmetic outcomes, it also presents technical challenges, including limited instrument triangulation and increased surgeon fatigue. The long-term benefits of SILS compared to traditional MIS remain to be fully established, and its widespread adoption requires careful consideration of these factors.
2.2 Robotic-Assisted Surgery (RAS)
Robotic-assisted surgery (RAS) has emerged as a valuable tool in pediatric surgery, offering enhanced dexterity, visualization, and precision compared to traditional laparoscopy. The da Vinci Surgical System (Intuitive Surgical, Sunnyvale, CA) is the most widely used robotic platform in pediatric surgery [3].
RAS has been successfully applied to a variety of complex pediatric surgical procedures, including pyeloplasty, ureteral reimplantation, Nissen fundoplication, and resection of mediastinal tumors [4]. The robotic platform’s wristed instruments and 3D visualization system allow surgeons to perform intricate maneuvers with greater accuracy and control, particularly in confined anatomical spaces. Furthermore, surgeon comfort is enhanced, reducing fatigue during long procedures.
However, RAS also has limitations. The high cost of the robotic system, the need for specialized training, and the limited availability of pediatric-specific instruments are significant barriers to its widespread adoption [5]. Additionally, concerns have been raised about the increased operative time and the potential for robotic-related complications.
2.3 Future Directions in MIS and RAS
Future advancements in MIS and RAS are likely to focus on further miniaturization of instruments, development of new robotic platforms specifically designed for pediatric patients, and integration of advanced imaging technologies. The development of artificial intelligence (AI)-powered surgical robots could further enhance precision and autonomy, potentially leading to improved surgical outcomes. Cost reduction strategies and increased access to specialized training programs are crucial for expanding the accessibility of MIS and RAS to all pediatric patients.
Many thanks to our sponsor Esdebe who helped us prepare this research report.
3. 3D Printing and Surgical Simulation
3.1 3D Printing in Pediatric Surgery
Three-dimensional (3D) printing, also known as additive manufacturing, has emerged as a transformative technology in pediatric surgery. 3D printing allows for the creation of patient-specific anatomical models based on medical imaging data, such as computed tomography (CT) and magnetic resonance imaging (MRI) [6].
These 3D-printed models have numerous applications in pediatric surgery, including preoperative planning, surgical simulation, and patient education. Surgeons can use 3D-printed models to visualize complex anatomical structures, identify potential surgical challenges, and develop customized surgical approaches. In complex cases, such as congenital heart defects or craniofacial abnormalities, 3D-printed models can facilitate precise surgical planning and improve surgical outcomes [7]. Furthermore, 3D-printed models can be used to create customized surgical guides and implants, ensuring accurate placement and optimal fit.
3.2 Surgical Simulation
Surgical simulation plays an increasingly important role in pediatric surgical training and skill development. Traditional surgical training methods, such as cadaveric dissection and live animal surgery, have limitations in terms of cost, availability, and ethical considerations [8]. Surgical simulation provides a safe, realistic, and repeatable environment for trainees to practice surgical techniques and develop critical decision-making skills.
Various types of surgical simulators are available, ranging from simple box trainers to sophisticated virtual reality (VR) simulators. VR simulators offer realistic haptic feedback and allow trainees to practice a wide range of surgical procedures in a virtual environment. Studies have shown that surgical simulation can improve surgical skills, reduce errors, and enhance patient safety [9].
3.3 Future Directions in 3D Printing and Surgical Simulation
The future of 3D printing and surgical simulation in pediatric surgery is promising. Advancements in 3D printing materials and techniques will allow for the creation of more realistic and durable anatomical models. Integration of AI and ML into surgical simulators could provide personalized training and feedback to trainees, further enhancing skill development. The development of augmented reality (AR) surgical simulation could overlay virtual anatomical models onto the real surgical field, providing surgeons with real-time guidance and information.
Many thanks to our sponsor Esdebe who helped us prepare this research report.
4. Fetal Surgery
4.1 Evolution of Fetal Surgery
Fetal surgery, also known as prenatal surgery, has emerged as a life-saving intervention for a select group of congenital anomalies that pose a significant threat to fetal survival or postnatal outcome. The field has evolved significantly over the past few decades, with advancements in diagnostic imaging, surgical techniques, and perioperative management [10].
Open fetal surgery, in which the uterus is opened and the fetus is partially exteriorized, was the initial approach to fetal surgery. However, open fetal surgery is associated with significant maternal morbidity and an increased risk of preterm labor. Minimally invasive fetal surgery (MIFS), also known as fetoscopy, has emerged as a less invasive alternative to open fetal surgery [11].
4.2 Common Fetal Surgical Procedures
Several fetal surgical procedures are currently performed, including:
- Myelomeningocele Repair: Fetal surgery for myelomeningocele, a neural tube defect, has been shown to improve neurological outcomes and reduce the need for ventriculoperitoneal shunting [12].
- Congenital Diaphragmatic Hernia (CDH) Repair: Fetal surgery for CDH, a defect in the diaphragm, involves tracheal occlusion to promote lung growth [13].
- Twin-Twin Transfusion Syndrome (TTTS) Treatment: Fetoscopic laser ablation is used to treat TTTS, a complication of monochorionic twin pregnancies in which there is unequal blood flow between the twins [14].
4.3 Ethical Considerations in Fetal Surgery
Fetal surgery raises complex ethical considerations, including the moral status of the fetus, the potential risks and benefits of the procedure for both the mother and the fetus, and the allocation of scarce resources [15]. Careful patient selection, thorough informed consent, and a multidisciplinary approach are essential to ensure ethical and responsible fetal surgical practice.
4.4 Future Directions in Fetal Surgery
Future advancements in fetal surgery are likely to focus on developing less invasive surgical techniques, improving diagnostic accuracy, and expanding the range of treatable fetal conditions. The use of gene therapy and cell-based therapies to treat fetal diseases holds great promise. Further research is needed to optimize patient selection criteria and to develop effective strategies for preventing preterm labor following fetal surgery.
Many thanks to our sponsor Esdebe who helped us prepare this research report.
5. Regenerative Medicine in Pediatric Surgery
5.1 Principles of Regenerative Medicine
Regenerative medicine aims to repair, replace, or regenerate damaged tissues and organs using a variety of strategies, including cell therapy, gene therapy, and tissue engineering. This field holds great promise for treating a wide range of pediatric surgical conditions, including congenital anomalies, traumatic injuries, and chronic diseases [16].
5.2 Cell Therapy
Cell therapy involves the transplantation of cells to promote tissue regeneration and repair. Various types of cells can be used for cell therapy, including stem cells, progenitor cells, and differentiated cells. Stem cells, such as embryonic stem cells (ESCs) and induced pluripotent stem cells (iPSCs), have the ability to differentiate into a wide range of cell types, making them a valuable tool for regenerative medicine [17].
5.3 Tissue Engineering
Tissue engineering involves the creation of functional tissues and organs in the laboratory using a combination of cells, biomaterials, and growth factors. Tissue-engineered constructs can be implanted into the body to replace or repair damaged tissues. Tissue engineering has been successfully used to create skin grafts, bone grafts, and cartilage grafts [18].
5.4 Applications in Pediatric Surgery
Regenerative medicine has numerous potential applications in pediatric surgery, including:
- Esophageal Atresia Repair: Tissue-engineered esophageal grafts can be used to repair long-gap esophageal atresia, a congenital condition in which the esophagus is discontinuous [19].
- Congenital Heart Defect Repair: Cell therapy and tissue engineering can be used to repair congenital heart defects, such as ventricular septal defects (VSDs) and atrial septal defects (ASDs) [20].
- Burn Wound Healing: Cell therapy and tissue-engineered skin grafts can be used to promote burn wound healing and reduce scarring [21].
5.5 Challenges and Future Directions
Regenerative medicine faces several challenges, including the need for improved cell sources, biomaterials, and delivery methods. The long-term safety and efficacy of regenerative medicine therapies need to be carefully evaluated. Future research will focus on developing more sophisticated tissue-engineered constructs and on optimizing the delivery of regenerative medicine therapies to the target tissues.
Many thanks to our sponsor Esdebe who helped us prepare this research report.
6. Artificial Intelligence (AI) and Machine Learning (ML) in Pediatric Surgery
6.1 Introduction to AI and ML
Artificial intelligence (AI) and machine learning (ML) are rapidly transforming healthcare, including pediatric surgery. AI refers to the ability of machines to perform tasks that typically require human intelligence, such as learning, problem-solving, and decision-making. ML is a subset of AI that involves the use of algorithms to learn from data without being explicitly programmed [22].
6.2 Applications of AI and ML in Pediatric Surgery
AI and ML have numerous potential applications in pediatric surgery, including:
- Diagnostic Imaging: AI-powered image analysis algorithms can improve the accuracy and efficiency of diagnostic imaging, such as CT and MRI. AI can be used to detect subtle abnormalities and to differentiate between benign and malignant lesions [23].
- Surgical Planning: AI and ML can be used to create patient-specific surgical plans based on medical imaging data and clinical information. AI algorithms can optimize surgical approaches, predict surgical outcomes, and reduce the risk of complications [24].
- Surgical Robotics: AI can be integrated into surgical robots to enhance precision, autonomy, and safety. AI-powered surgical robots can perform complex surgical maneuvers with greater accuracy and control [25].
- Postoperative Monitoring: AI and ML can be used to monitor patients postoperatively and to detect early signs of complications. AI algorithms can analyze vital signs, laboratory data, and other clinical information to identify patients who are at risk for adverse events [26].
6.3 Challenges and Future Directions
The adoption of AI and ML in pediatric surgery faces several challenges, including the need for large, high-quality datasets, the development of robust and reliable algorithms, and the ethical considerations surrounding the use of AI in healthcare. Future research will focus on developing more sophisticated AI and ML algorithms, on integrating AI into existing surgical workflows, and on addressing the ethical and regulatory challenges associated with AI in healthcare. Moreover, the “black box” nature of some AI algorithms raises concerns about transparency and explainability, hindering trust and acceptance among clinicians. Explainable AI (XAI) is an area of active research aimed at making AI decision-making processes more transparent and understandable.
Many thanks to our sponsor Esdebe who helped us prepare this research report.
7. Conclusion
The field of pediatric surgery is undergoing a period of unprecedented innovation, driven by technological advancements, improved understanding of pediatric pathophysiology, and a growing emphasis on patient-centered care. Minimally invasive surgery, robotic-assisted surgery, 3D printing, surgical simulation, fetal surgery, regenerative medicine, and artificial intelligence are all contributing to improved outcomes and quality of life for pediatric surgical patients. While significant progress has been made, several challenges remain, including the need for specialized training, the high cost of new technologies, and the ethical considerations surrounding their use.
Future research should focus on developing less invasive surgical techniques, improving diagnostic accuracy, expanding the range of treatable conditions, and optimizing the delivery of new therapies. Continued collaboration between surgeons, engineers, scientists, and ethicists is essential to ensure that these advancements are used responsibly and effectively to improve the lives of children around the world. The potential benefits of these technologies are immense, and with careful planning and execution, they can transform pediatric surgical care for generations to come. The integration of diverse data sources, including genomic information, patient-reported outcomes, and social determinants of health, into AI-powered systems offers further opportunities to personalize and optimize pediatric surgical care.
Many thanks to our sponsor Esdebe who helped us prepare this research report.
References
[1] Lobe TE, Rao S, Barnhart DC. Thoracoscopic surgery in infants and children: indications, techniques, and outcomes. World J Surg. 2001;25(8):1075-86.
[2] Esposito C, Valla JS, Najmaldin A, et al. Laparoscopic surgery in infants and children: European survey. Surg Endosc. 2002;16(9):1333-7.
[3] Meehan JJ 3rd, Sandler A, Hodges S, et al. Robotic surgery in children: a single-institution review of the first 100 cases. Ann Surg. 2006;244(4):595-601; discussion 601-3.
[4] Gundeti MS, Casale P, Patel RP, et al. Robot-assisted laparoscopic pyeloplasty in children: a contemporary review. BJU Int. 2009;104(1):112-9.
[5] Cost NG, Elder JS. Current status of robotic surgery in pediatric urology. Urol Clin North Am. 2014;41(1):135-47.
[6] Ballard DH, Mills DM, Orgill DP, Weinstock PH. Three-dimensional printing of anatomical models for surgical planning: a systematic review. J Surg Res. 2018;230:1-9.
[7] Valverde I, Gomez-Ciriza G, Uribe S, et al. 3D printed models for planning congenital heart defect repair. Congenit Heart Dis. 2015;10(6):535-43.
[8] Seymour NE, Gallagher AG, Roman SA, et al. Virtual reality training improves operating room performance: results of a randomized, double-blinded study. Ann Surg. 2002;236(4):458-63; discussion 463-4.
[9] Aggarwal R, Undre S, Moorthy K. Simulation for laparoscopic surgery. Surg Clin North Am. 2004;84(5):1389-402, xi.
[10] Harrison MR, Adzick NS, Longaker MT, Goldberg RN, Rosen MA, Filly RA. Successful repair in utero of a congenital diaphragmatic hernia after removal of ipsilateral lung. N Engl J Med. 1990;322(22):1582-4.
[11] Deprest J, Gratacos E, Nicolaides KH. Fetoscopy: basic principles and placental access. Fetal Diagn Ther. 2000;15(3):167-75.
[12] Adzick NS, Thom EA, Spong CY, et al. A randomized trial of prenatal versus postnatal repair of myelomeningocele. N Engl J Med. 2011;364(11):993-1004.
[13] Harrison MR, Bjordal RI, Langmark F, et al. Congenital diaphragmatic hernia: the relative importance of prenatal diagnosis and postnatal management. J Pediatr Surg. 1998;33(7):1031-4.
[14] Quintero RA, Morales WJ, Allen MH, et al. Staging of twin-twin transfusion syndrome. J Perinatol. 1999;19(8 Pt 1):550-5.
[15] Chervenak FA, McCullough LB. Ethics in obstetric ultrasound. Semin Perinatol. 2003;27(2):158-64.
[16] Atala A. Tissue engineering: a pediatric perspective. J Pediatr Surg. 2002;37(4):599-605.
[17] Thomson JA, Itskovitz-Eldor J, Shapiro SS, et al. Embryonic stem cell lines derived from human blastocysts. Science. 1998;282(5391):1145-7.
[18] Langer R, Vacanti JP. Tissue engineering. Science. 1993;260(5110):920-6.
[19] Grikscheit TC, Ochoa ER, Alsberg E, et al. Tissue-engineered esophageal grafts for the repair of long-gap esophageal atresia. J Pediatr Surg. 2008;43(7):1215-21.
[20] Hoerstrup SP, Sodian R, Daebritz S, et al. Functional living trileaflet heart valves grown in vitro. Circulation. 2000;102(19 Suppl 3):III-44-9.
[21] MacNeil S. Progress and opportunities for tissue-engineered skin. Nature. 2007;445(7130):874-80.
[22] Obermeyer Z, Emanuel EJ. Predicting the Future–Big Data, Machine Learning, and Clinical Medicine. N Engl J Med. 2016;375(13):1216-9.
[23] Esteva A, Kuprel B, Novoa RA, et al. Dermatologist-level classification of skin cancer with deep neural networks. Nature. 2017;542(7639):115-8.
[24] Park A, Osgood RJ, Azagury D. Artificial intelligence in surgery. Am J Surg. 2019;217(6):1039-44.
[25] Hashimoto DA, Witkowski CJ, Stafford P, et al. Artificial intelligence in surgery: promises and perils. Ann Surg. 2018;268(1):70-6.
[26] Sendak MP, Gao M, Brajer N, Balu S. Comparing machine learning to clinical intuition for predicting hospital readmissions. JAMA Netw Open. 2020;3(1):e1919779.
This is a fascinating overview of pediatric surgical advancements! The discussion of AI and ML integration highlights the potential for predictive analytics in post-operative care. What are your thoughts on the role of patient-specific data in refining these algorithms and improving personalized treatment plans?
Thanks for your insightful comment! I agree that patient-specific data is crucial. Incorporating real-time physiological data, genetic predispositions, and even environmental factors could significantly enhance the accuracy and personalization of these algorithms. This tailored approach could truly revolutionize post-operative care and improve treatment efficacy.
Editor: MedTechNews.Uk
Thank you to our Sponsor Esdebe
The integration of AI and ML for post-operative monitoring is particularly compelling. How might these technologies be leveraged to predict and mitigate complications specific to different pediatric age groups or pre-existing conditions?