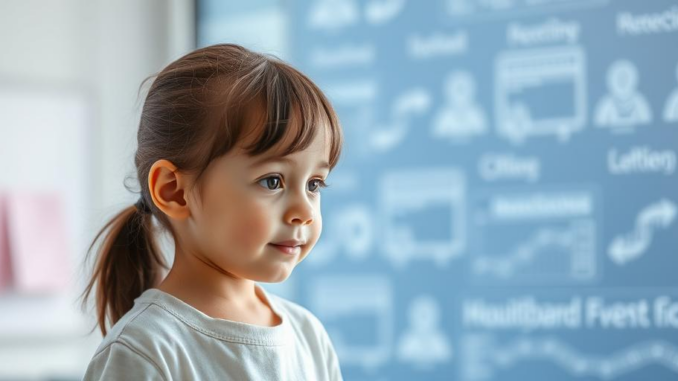
Abstract
Genetic testing has revolutionized the diagnosis and management of neurological disorders, particularly in pediatric populations. This research report provides a comprehensive overview of the evolving landscape of genetic testing in pediatric neurology, encompassing the types of tests employed, the complexities of interpreting results, ethical considerations, and emerging technologies. We delve into various diagnostic approaches, from targeted single-gene testing to comprehensive genomic sequencing, highlighting their strengths and limitations. Furthermore, we examine the impact of genetic findings on clinical decision-making, including therapeutic interventions and genetic counseling. A critical discussion of the ethical challenges surrounding genetic testing in children, such as informed consent, incidental findings, and potential for genetic discrimination, is presented. Finally, we explore advancements in genetic technologies, including long-read sequencing and epigenetic analysis, and their promise for enhancing diagnostic accuracy and personalized treatment strategies for pediatric neurological disorders. The report emphasizes the importance of equitable access to genetic testing and the need for ongoing research to refine our understanding of the genetic basis of these complex conditions.
Many thanks to our sponsor Esdebe who helped us prepare this research report.
1. Introduction
Neurological disorders represent a significant cause of morbidity and mortality in children, impacting development, cognition, and overall quality of life. While some neurological conditions are acquired, a substantial proportion are attributed to genetic etiologies. Consequently, genetic testing has emerged as a crucial diagnostic tool in pediatric neurology, enabling accurate diagnosis, risk assessment, and informed clinical management. This report aims to provide a comprehensive review of the current state of genetic testing in pediatric neurological disorders, encompassing the available technologies, interpretation of results, ethical considerations, and future directions. The ever-increasing complexity of genomic data necessitates a thorough understanding of these aspects to optimize patient care.
Many thanks to our sponsor Esdebe who helped us prepare this research report.
2. Types of Genetic Tests Relevant to Pediatric Neurological Disorders
A diverse array of genetic tests are available for the investigation of neurological disorders in children. The selection of the appropriate test depends on the clinical presentation, family history, and suspected underlying genetic mechanisms.
2.1. Targeted Single-Gene Testing
Targeted single-gene testing, typically performed via Sanger sequencing, remains a valuable approach when a specific genetic etiology is strongly suspected based on clinical features. This method involves sequencing a particular gene known to be associated with the suspected disorder. For example, in suspected cases of Spinal Muscular Atrophy (SMA), SMN1 gene testing is a common first-line investigation. The advantages of single-gene testing include its relatively low cost, rapid turnaround time, and high sensitivity for detecting specific mutations. However, its major limitation is the inability to identify mutations in other genes that may present with similar phenotypes. Targeted approaches are also appropriate when confirming a variant previously identified in a family member.
2.2. Gene Panels
Gene panels represent an intermediate approach, targeting a pre-defined set of genes associated with a specific group of disorders or a particular phenotype. These panels are typically analyzed using next-generation sequencing (NGS) technologies, allowing for simultaneous analysis of multiple genes. For instance, a comprehensive epilepsy gene panel may include genes associated with various epilepsy syndromes, such as SCN1A, KCNQ2, and CDKL5. Gene panels offer a broader diagnostic scope than single-gene testing, increasing the likelihood of identifying a causative variant in phenotypically heterogeneous disorders. The choice of panel should be carefully considered based on the patient’s clinical presentation to maximize diagnostic yield and minimize the identification of variants of uncertain significance (VUS).
2.3. Exome Sequencing
Exome sequencing (ES) involves sequencing the protein-coding regions (exons) of all genes in the human genome, representing approximately 1-2% of the total genome but containing the vast majority of disease-causing variants. ES has become a powerful tool for diagnosing genetically heterogeneous disorders, especially when single-gene testing and gene panels have been uninformative. In pediatric neurology, ES is often employed in cases of undiagnosed developmental delay, intellectual disability, and complex neurological syndromes. The major advantage of ES is its ability to identify novel disease-causing genes and rare variants not included in targeted panels. However, ES also presents challenges, including the high cost of sequencing and analysis, the increased likelihood of identifying VUS, and the ethical considerations associated with incidental findings.
2.4. Genome Sequencing
Genome sequencing (GS) involves sequencing the entire human genome, including both coding and non-coding regions. While more expensive and computationally demanding than ES, GS offers the most comprehensive assessment of genetic variation. GS can identify variants in non-coding regions, such as regulatory elements and deep intronic regions, which may be missed by ES. Furthermore, GS provides information on structural variants, copy number variations (CNVs), and repetitive sequences. Although GS is not yet widely used in routine clinical practice, it is increasingly being employed in research settings and for particularly challenging diagnostic cases. The increasing accessibility and decreasing cost of GS suggest that it will play a more prominent role in the future of pediatric genetic testing for neurological disorders.
2.5. Chromosomal Microarray Analysis (CMA)
Chromosomal microarray analysis (CMA) is a widely used technique for detecting copy number variations (CNVs), such as deletions and duplications of chromosomal regions. CNVs can disrupt gene dosage and function, leading to a variety of neurological disorders, including intellectual disability, autism spectrum disorder (ASD), and epilepsy. CMA is typically performed using oligonucleotide arrays or single nucleotide polymorphism (SNP) arrays. Oligonucleotide arrays measure the relative abundance of DNA sequences throughout the genome, while SNP arrays assess the presence of specific SNPs, providing information on both CNVs and loss of heterozygosity (LOH). CMA is a valuable first-tier test for children with unexplained developmental delay or intellectual disability.
2.6. Mitochondrial DNA Sequencing
Mitochondrial DNA (mtDNA) encodes genes essential for mitochondrial function, and mutations in mtDNA can cause a variety of neurological disorders, including mitochondrial encephalopathies, myopathies, and neurodegenerative diseases. mtDNA sequencing is used to detect mutations in the mitochondrial genome, either by targeted sequencing of specific regions or by sequencing the entire mtDNA molecule. Unlike nuclear DNA, mtDNA is maternally inherited, and individuals can harbor a mixture of mutant and wild-type mtDNA, a phenomenon known as heteroplasmy. The level of heteroplasmy can vary between tissues and individuals, influencing the severity of the phenotype. mtDNA sequencing is essential for diagnosing mitochondrial disorders and for guiding genetic counseling.
Many thanks to our sponsor Esdebe who helped us prepare this research report.
3. The Process of Genetic Testing
The genetic testing process involves several key steps, from initial consultation to interpretation of results and post-test counseling.
3.1. Pre-Test Counseling and Informed Consent
Prior to genetic testing, thorough pre-test counseling is essential. This involves discussing the purpose of the test, the potential benefits and limitations, the possible outcomes (positive, negative, or uncertain results), the risks associated with testing (e.g., anxiety, emotional distress), and the implications for the patient and their family. Informed consent must be obtained from the patient (if capable) or their legal guardian, documenting that the individual understands the information provided and voluntarily agrees to undergo testing. Special considerations apply to pediatric populations, where the child’s assent should be obtained whenever possible, even if parental consent is the primary requirement. Particular attention should be given to potential privacy concerns regarding data security and the possible impact on insurability or future employment opportunities.
3.2. Sample Collection and Processing
The type of sample required for genetic testing depends on the specific test being performed. Common sample types include blood, saliva, and buccal swabs. In some cases, tissue biopsies (e.g., muscle biopsy for mitochondrial disorders) or cerebrospinal fluid (CSF) may be necessary. Proper sample collection, handling, and storage are critical to ensure the accuracy and reliability of the test results. DNA is extracted from the sample using standard laboratory protocols. The extracted DNA is then prepared for sequencing, which may involve library preparation, target enrichment (e.g., exome capture), and amplification.
3.3. Sequencing and Data Analysis
Sequencing is performed using various platforms, depending on the type of test being conducted. Sanger sequencing is used for targeted single-gene testing, while NGS technologies are employed for gene panels, exome sequencing, and genome sequencing. The raw sequencing data is then processed using bioinformatics pipelines, which involve alignment to a reference genome, variant calling (identifying differences between the individual’s DNA sequence and the reference genome), and variant annotation (providing information on the location, frequency, and predicted functional impact of each variant). Variant filtering is performed to prioritize variants that are more likely to be disease-causing, based on factors such as variant frequency, predicted pathogenicity, and co-segregation with the phenotype in affected family members.
3.4. Variant Interpretation and Reporting
Variant interpretation is a critical step in the genetic testing process. Variants are classified according to the American College of Medical Genetics and Genomics (ACMG) guidelines into five categories: pathogenic, likely pathogenic, variant of uncertain significance (VUS), likely benign, and benign. This classification is based on multiple lines of evidence, including variant frequency in the general population, predicted functional impact (e.g., missense, frameshift, splice site), segregation with the phenotype in families, and functional studies. Variants classified as pathogenic or likely pathogenic are considered causative for the disorder. VUS are variants for which there is insufficient evidence to determine their pathogenicity. These variants require further investigation, and reclassification may be possible as new evidence becomes available. The genetic testing report summarizes the findings, including the identified variants, their classification, and the clinical implications. The report should be clear, concise, and easy to understand for both clinicians and patients.
3.5. Post-Test Counseling and Genetic Counseling
Post-test counseling is essential to discuss the test results with the patient and their family. For positive results, the implications of the diagnosis are explained, including the prognosis, available treatment options, and the risk of recurrence in future pregnancies. For negative results, the possibility of other genetic causes, non-genetic causes, or the limitations of the test are discussed. For VUS, the need for further investigation and the possibility of reclassification in the future are explained. Genetic counseling is a critical component of post-test counseling. Genetic counselors provide information on the genetic basis of the disorder, inheritance patterns, reproductive options, and psychosocial support. They also help families navigate the emotional and ethical challenges associated with genetic testing.
Many thanks to our sponsor Esdebe who helped us prepare this research report.
4. Costs Associated with Genetic Testing
The cost of genetic testing can vary widely depending on the type of test, the laboratory performing the test, and the insurance coverage. Targeted single-gene testing is typically the least expensive, while genome sequencing is the most expensive. Gene panels and exome sequencing fall in between. The cost of genetic testing includes the cost of sample collection and processing, sequencing, data analysis, variant interpretation, and reporting. In addition to the cost of the test itself, there may be additional costs associated with pre-test and post-test counseling. The high cost of genetic testing can be a barrier to access, particularly for families with limited resources or inadequate insurance coverage. Efforts are underway to reduce the cost of genetic testing and to improve access for all patients who could benefit.
Many thanks to our sponsor Esdebe who helped us prepare this research report.
5. Interpretation of Results
Interpreting the results of genetic testing in pediatric neurological disorders is complex and requires expertise in genetics, neurology, and bioinformatics. Several factors can complicate variant interpretation:
5.1. Variants of Uncertain Significance (VUS)
The identification of VUS is a common challenge in genetic testing. VUS are variants for which there is insufficient evidence to determine their pathogenicity. VUS can cause anxiety and uncertainty for patients and families. Further investigation is often required to determine the significance of VUS, which may involve segregation analysis in families, functional studies, and collaboration with other researchers. As new evidence becomes available, VUS can be reclassified as pathogenic, likely pathogenic, likely benign, or benign.
5.2. Phenotypic Heterogeneity and Variable Expressivity
Many neurological disorders exhibit phenotypic heterogeneity, meaning that individuals with the same genetic mutation can present with different symptoms or severity. Variable expressivity refers to the degree to which a phenotype is manifested in different individuals with the same mutation. These factors can complicate the interpretation of genetic test results and make it challenging to predict the clinical outcome. Modifier genes, environmental factors, and epigenetic modifications can all contribute to phenotypic heterogeneity and variable expressivity.
5.3. De Novo Mutations
De novo mutations are genetic changes that occur spontaneously in the egg or sperm or during early embryonic development. De novo mutations are a common cause of sporadic genetic disorders, meaning that the disorder is not inherited from the parents. Identifying de novo mutations requires careful analysis of the patient’s DNA sequence and comparison with the parents’ DNA sequences. Confirmation of de novo status is crucial for accurate diagnosis and genetic counseling.
5.4. Mosaicism
Mosaicism refers to the presence of two or more genetically distinct cell populations in an individual. Mosaicism can result from post-zygotic mutations, meaning that the mutation occurs after fertilization. The proportion of cells carrying the mutation can vary between tissues, leading to variable expressivity. Detecting mosaicism requires sensitive sequencing techniques and analysis of multiple tissue samples. Mosaicism can complicate genetic testing and interpretation of results.
Many thanks to our sponsor Esdebe who helped us prepare this research report.
6. Ethical Considerations
Genetic testing in pediatric populations raises several ethical considerations.
6.1. Informed Consent and Assent
Obtaining informed consent from parents or legal guardians is essential for genetic testing in children. However, children also have the right to participate in the decision-making process, and their assent should be obtained whenever possible. The child’s ability to understand the information and express their wishes should be taken into consideration. Parents may have different motivations for pursuing genetic testing than the child, and it is important to ensure that the child’s best interests are prioritized.
6.2. Incidental Findings
Incidental findings are genetic variants that are unrelated to the primary reason for testing but may have implications for the individual’s health or the health of their family members. The ACMG recommends that laboratories report a minimum set of actionable incidental findings, which are variants that have a high likelihood of causing a serious health condition and for which there is a proven intervention. However, the decision to report incidental findings is controversial, as it may raise ethical concerns about the right to know and the potential for psychological distress. Parents should be informed about the possibility of incidental findings before genetic testing and given the opportunity to opt out of receiving this information.
6.3. Genetic Discrimination
Genetic discrimination refers to the unfair treatment of individuals based on their genetic information. Genetic information can be used to discriminate against individuals in employment, insurance, and other contexts. The Genetic Information Nondiscrimination Act (GINA) in the United States prohibits genetic discrimination in health insurance and employment. However, GINA does not protect against genetic discrimination in life insurance, disability insurance, or long-term care insurance. Concerns about genetic discrimination can deter individuals from undergoing genetic testing.
6.4. Data Privacy and Security
Genetic information is highly sensitive and personal, and it is important to protect the privacy and security of this data. Genetic data should be stored securely and accessed only by authorized personnel. Patients should have control over their genetic data and the ability to access and correct their information. Data breaches and unauthorized access to genetic data can have serious consequences for individuals and their families.
Many thanks to our sponsor Esdebe who helped us prepare this research report.
7. Advancements in Genetic Testing Technologies
Several advancements in genetic testing technologies are improving the diagnosis and management of pediatric neurological disorders.
7.1. Long-Read Sequencing
Long-read sequencing technologies, such as those developed by Pacific Biosciences and Oxford Nanopore Technologies, can sequence DNA fragments that are much longer than those sequenced by traditional NGS methods. Long-read sequencing can improve the accuracy of variant calling, particularly in regions of the genome that are difficult to sequence with short-read technologies, such as repetitive sequences and regions with high GC content. Long-read sequencing can also facilitate the detection of structural variants and complex genomic rearrangements.
7.2. RNA Sequencing
RNA sequencing (RNA-Seq) is a technique used to measure the levels of gene expression in a sample. RNA-Seq can identify changes in gene expression that are associated with neurological disorders. RNA-Seq can also detect alternative splicing events and novel transcripts. RNA-Seq can be used to complement DNA sequencing and to provide additional insights into the molecular mechanisms underlying neurological disorders.
7.3. Epigenetic Analysis
Epigenetics refers to changes in gene expression that are not caused by changes in the DNA sequence. Epigenetic modifications, such as DNA methylation and histone modifications, can influence gene expression and contribute to the development of neurological disorders. Epigenetic analysis can identify changes in DNA methylation patterns and histone modifications that are associated with neurological disorders. Epigenetic analysis can also be used to study the effects of environmental factors on gene expression.
7.4. Single-Cell Sequencing
Single-cell sequencing allows for the analysis of gene expression and other molecular features in individual cells. Single-cell sequencing can be used to study the cellular heterogeneity of neurological disorders and to identify disease-specific cell types. Single-cell sequencing can also be used to study the effects of genetic mutations on individual cells.
Many thanks to our sponsor Esdebe who helped us prepare this research report.
8. Conclusion
Genetic testing has become an indispensable tool in the diagnosis and management of pediatric neurological disorders. Advances in genetic testing technologies, such as NGS, long-read sequencing, and epigenetic analysis, are improving the accuracy and efficiency of genetic testing. However, the interpretation of genetic test results is complex and requires expertise in genetics, neurology, and bioinformatics. Ethical considerations, such as informed consent, incidental findings, and genetic discrimination, must be carefully addressed. Continued research and development are needed to improve our understanding of the genetic basis of pediatric neurological disorders and to develop more effective diagnostic and therapeutic strategies. Equitable access to genetic testing for all children, irrespective of their race or socioeconomic status, is crucial to ensure optimal outcomes and reduce health disparities.
Many thanks to our sponsor Esdebe who helped us prepare this research report.
References
- American College of Medical Genetics and Genomics (ACMG).
- Genetic Information Nondiscrimination Act (GINA).
- National Human Genome Research Institute (NHGRI).
- Posey, J. E., & Rosenfeld, J. A. (2020). Diagnostic exome sequencing in pediatrics. Seminars in Perinatology, 44(1), 151177.
- Manolio, T. A., et al. (2009). Finding the missing heritability of complex diseases. Nature, 461(7265), 747-753.
- Lupski, J. R., et al. (2010). Clinical genome sequencing for diagnosis of Mendelian disorders. Science Translational Medicine, 2(31), 31ra13.
- Kingsmore, S. F., et al. (2012). Next-generation DNA sequencing of a single patient to identify an etiology for a rare disease. Genome Research, 22(11), 2227-2236.
- Reuter, C. M., et al. (2017). Diagnostic yield of exome sequencing in children with neurological disorders. European Journal of Human Genetics, 25(4), 431-439.
- Lionel, A. C., et al. (2018). Exome sequencing and implications for autism spectrum disorder. Expert Reviews in Molecular Medicine, 20, e14.
- Splinter, K., et al. (2018). Effect of genetic diagnosis on families with severely affected children. JAMA Pediatrics, 172(1), 23-29.
- Wright CF, FitzPatrick DR, Firth HV. Paediatric diagnostics: why genetics is mainstream. Arch Dis Child. 2018 Dec;103(12):1177-1181. doi: 10.1136/archdischild-2017-313856. Epub 2017 Oct 20. PMID: 29056510; PMCID: PMC6255197.
- Dharajiya SG, Kingsmore SF. Clinical application of whole-genome sequencing in children with developmental delay. Transl Sci Rare Dis. 2018 May 24;3(1-2):1-12. doi: 10.3233/TRD-180019. PMID: 30410997; PMCID: PMC6212346.
Be the first to comment