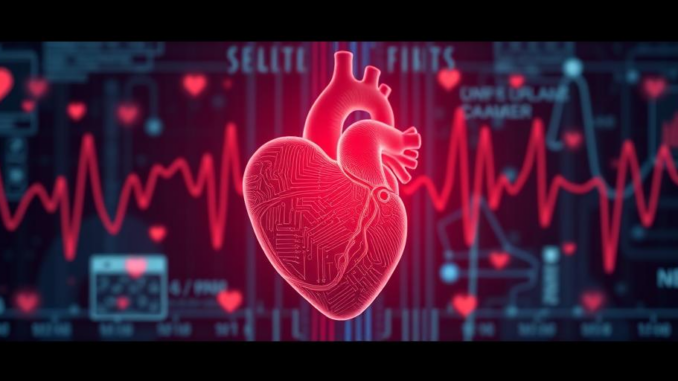
Abstract
Cardiac pacing has revolutionized the treatment of bradyarrhythmias, providing life-sustaining support for millions worldwide. This report provides a comprehensive overview of cardiac pacing technologies, encompassing the evolution from traditional transvenous systems to the cutting-edge bioresorbable pacemakers. We delve into the technological underpinnings of each system, focusing on materials science, energy delivery mechanisms, and leadless designs. A comparative analysis highlights the advantages and disadvantages of each approach, considering factors such as longevity, complication rates, patient suitability, and cost-effectiveness. Furthermore, we explore the regulatory landscape governing the development and approval of cardiac pacing devices and discuss the potential future directions of this rapidly evolving field, including light-activated pacing and wireless energy transfer. The aim is to provide a critical and insightful perspective on the current state and future trajectory of cardiac pacing technology, beneficial to experts in the field.
Many thanks to our sponsor Esdebe who helped us prepare this research report.
1. Introduction
Cardiac pacing is a cornerstone therapy for individuals suffering from bradyarrhythmias and other conduction disturbances. The first implantable pacemaker was a groundbreaking innovation, dramatically improving the quality of life and extending the lifespan of patients with compromised cardiac function. While early devices were rudimentary, the field has witnessed remarkable advancements, progressing from fixed-rate pacing to sophisticated rate-adaptive, multi-chamber, and now leadless and bioresorbable systems. The constant drive for improvement has been fuelled by the desire to minimize complications, enhance device longevity, optimize physiological pacing, and expand the applicability of pacing therapy to a broader patient population.
This report provides a comprehensive overview of the current state-of-the-art in cardiac pacing technology. We will examine the evolution of pacing systems, detailing the specific technologies, materials, and design considerations that characterize traditional, leadless, and bioresorbable pacemakers. A comparative analysis will assess the relative merits and drawbacks of each approach, focusing on clinical performance, potential complications, and economic implications. Furthermore, we will discuss the regulatory framework governing the development and approval of these devices and offer insights into the future directions of cardiac pacing research, including novel energy sources, advanced materials, and innovative pacing modalities.
Many thanks to our sponsor Esdebe who helped us prepare this research report.
2. Historical Perspective of Cardiac Pacing
The history of cardiac pacing is a testament to the ingenuity and perseverance of medical researchers and engineers. The initial attempts at artificial pacing involved external stimulators, which were cumbersome and delivered painful shocks. The development of the first implantable pacemaker in 1958 by Rune Elmqvist and Ake Senning marked a pivotal moment [1]. This device, powered by rechargeable batteries, provided a reliable means of delivering electrical impulses to the heart. Early challenges included battery depletion, lead failure, and device infection.
Throughout the 1960s and 1970s, significant advancements were made in battery technology, moving from rechargeable to mercury-zinc batteries, which offered longer lifespans. Lead technology also improved, with the introduction of silicone rubber insulation and active fixation mechanisms. The development of rate-adaptive pacemakers, which could increase pacing rate in response to physical activity, represented a major step forward in physiological pacing. Dual-chamber pacing, which sequentially stimulated the atrium and ventricle, further enhanced hemodynamic performance by preserving atrioventricular synchrony.
The advent of microelectronics in the 1980s and 1990s revolutionized pacemaker design, allowing for more complex algorithms and programmability. This led to the development of sophisticated features such as mode switching, which automatically altered pacing modes in response to atrial arrhythmias, and rate drop response, which prevented pauses after sudden drops in heart rate. Cardiac resynchronization therapy (CRT), introduced in the late 1990s, provided a new treatment option for patients with heart failure and conduction delays, improving ventricular function and reducing heart failure symptoms.
More recently, the development of leadless pacemakers has addressed some of the limitations associated with traditional transvenous leads. These devices are implanted directly into the right ventricle, eliminating the need for leads and reducing the risk of lead-related complications. The most recent development is the bioresorbable pacemaker, which degrades in the body once it is no longer needed. This can reduce the need for extraction surgery and other complications associated with implanted devices.
Many thanks to our sponsor Esdebe who helped us prepare this research report.
3. Types of Cardiac Pacemakers
Cardiac pacing technology has diversified significantly to address a wide range of clinical needs. The primary types of pacemakers can be broadly categorized as follows:
3.1. Traditional Transvenous Pacemakers
Traditional transvenous pacemakers consist of a pulse generator implanted in the pectoral region and one or more leads that are threaded through the venous system to the heart. These leads deliver electrical impulses to stimulate the atria and/or ventricles. Traditional pacemakers can be single-chamber (pacing either the atrium or ventricle), dual-chamber (pacing both the atrium and ventricle), or biventricular (pacing both ventricles, typically for CRT).
The advantages of traditional transvenous pacemakers include their versatility, programmability, and long-term track record. They can be programmed to provide a wide range of pacing modes, allowing for individualized therapy tailored to the patient’s specific needs. However, traditional pacemakers are associated with a risk of lead-related complications, such as lead dislodgement, lead fracture, infection, and venous thrombosis. These complications can require surgical intervention and can significantly impact patient morbidity.
3.2. Leadless Pacemakers
Leadless pacemakers are self-contained devices that are implanted directly into the right ventricle via a transcatheter approach. These devices eliminate the need for transvenous leads, thereby reducing the risk of lead-related complications. Leadless pacemakers are typically smaller than traditional pacemakers and are powered by batteries with a finite lifespan.
The primary advantage of leadless pacemakers is the reduced risk of lead-related complications. Clinical trials have demonstrated that leadless pacemakers are associated with a lower incidence of lead dislodgement, lead fracture, and infection compared to traditional transvenous pacemakers [2]. However, leadless pacemakers have some limitations. They are currently limited to single-chamber ventricular pacing and are not suitable for patients who require atrial pacing or CRT. Furthermore, the battery life of leadless pacemakers is typically shorter than that of traditional pacemakers, and device retrieval can be challenging. Also, the lack of backwards compatibility with existing pacemaker technology requires clinicians to consider potential challenges when future devices might be needed.
3.3. Bioresorbable Pacemakers
Bioresorbable pacemakers represent a novel approach to cardiac pacing, offering the potential to eliminate the need for permanent implants. These devices are constructed from biodegradable materials that dissolve harmlessly within the body after a predetermined period. This avoids the risk of long-term complications associated with permanent implants, such as infection, erosion, and the need for extraction. Early bioresorbable pacemakers are designed for temporary pacing, such as after cardiac surgery or during recovery from an acute myocardial infarction.
The advantages of bioresorbable pacemakers are clear: they eliminate the need for device retrieval and reduce the risk of long-term complications. However, bioresorbable pacemakers are still in the early stages of development and have several limitations. The battery life is limited and the materials need to be carefully selected to be biocompatible and ensure proper degradation. Further research is needed to improve the performance, longevity, and reliability of these devices before they can be widely adopted. In addition, the regulatory pathway for these novel devices is still evolving, and significant clinical data will be required to demonstrate their safety and efficacy.
Many thanks to our sponsor Esdebe who helped us prepare this research report.
4. Technology Behind Pacemakers
The technology behind pacemakers encompasses a wide range of engineering and scientific disciplines, including microelectronics, materials science, and signal processing. The key technological components of a pacemaker include:
4.1. Power Source
The power source is a critical component of any pacemaker, determining its longevity and reliability. Traditional pacemakers typically use lithium-iodide batteries, which offer a high energy density and a long lifespan. Leadless pacemakers also use lithium-based batteries, but their smaller size limits their energy capacity. Bioresorbable pacemakers may use a variety of power sources, including biodegradable batteries or wireless energy transfer systems. The advancement of micro-battery technology is crucial for the development of long-lasting, minimally invasive pacemakers.
4.2. Sensing and Pacing Circuitry
The sensing and pacing circuitry is responsible for detecting intrinsic cardiac activity and delivering electrical impulses to stimulate the heart. This circuitry typically includes amplifiers, filters, and microprocessors that process the electrical signals from the heart and control the timing and amplitude of the pacing pulses. In rate-adaptive pacemakers, accelerometers or other sensors are used to detect physical activity and adjust the pacing rate accordingly. The miniaturization and integration of these circuits are essential for the development of smaller, more efficient pacemakers. The sensitivity of the sensing circuitry is also crucial for proper function, avoiding over- or under-sensing of cardiac events.
4.3. Leads (Traditional Pacemakers)
Leads are the wires that connect the pulse generator to the heart, delivering electrical impulses and transmitting cardiac signals back to the generator. Leads are typically insulated with silicone rubber or polyurethane and are designed to be flexible and durable. The tip of the lead is designed to make secure contact with the heart tissue, either through active fixation (using a screw or tine) or passive fixation (using a mesh or porous surface). Lead design is crucial to minimize the risk of dislodgement, fracture, and infection. Research into new lead materials and designs is ongoing, with the goal of improving lead reliability and reducing the incidence of lead-related complications. Advanced lead designs also focus on steroid elution to reduce inflammation at the lead-tissue interface, improving pacing thresholds and reducing the risk of micro-dislodgement.
4.4. Materials for Bioresorbable Pacemakers
The development of bioresorbable pacemakers relies on the use of biocompatible and biodegradable materials. These materials must be able to perform their intended function for a specified period and then degrade harmlessly within the body. Common materials used in bioresorbable pacemakers include polymers, metals, and ceramics. For example, Polylactic acid (PLA), Polyglycolic acid (PGA), and their copolymers (PLGA) are commonly used biodegradable polymers. Magnesium and zinc are biodegradable metals. The choice of materials depends on the specific application and the desired degradation rate. The mechanical and electrical properties of these materials must also be carefully considered to ensure proper device function. Moreover, ensuring that the degradation products are non-toxic and readily cleared by the body is paramount [3].
4.5 Light Activated Pacemakers
Light-activated pacemakers are an emerging technology that uses optogenetics to control heart rhythm. Optogenetics involves genetically modifying heart cells to express light-sensitive proteins called channelrhodopsins. When these proteins are exposed to light, they open ion channels, allowing ions to flow into the cell and trigger an action potential. A light-activated pacemaker would deliver pulses of light to the heart, stimulating the genetically modified cells and pacing the heart.
Light-activated pacemakers offer several potential advantages over traditional pacemakers. They could provide more precise and physiological pacing, as the light pulses can be targeted to specific cells or regions of the heart. They also avoid the need for electrical leads, which can be a source of complications. However, light-activated pacemakers are still in the early stages of development, and several challenges need to be addressed before they can be used in humans. These challenges include developing efficient and safe gene delivery methods, optimizing the light delivery system, and ensuring the long-term stability of the genetically modified cells. While gene therapy still faces significant regulatory hurdles, the potential of light-activated pacing is a very exciting field [4].
Many thanks to our sponsor Esdebe who helped us prepare this research report.
5. Regulatory Landscape
The development and approval of cardiac pacemakers are subject to rigorous regulatory oversight in most countries. In the United States, the Food and Drug Administration (FDA) regulates medical devices under the Federal Food, Drug, and Cosmetic Act. Pacemakers are typically classified as Class III devices, which require premarket approval (PMA) before they can be marketed. The PMA process involves a thorough review of the device’s safety and effectiveness, including preclinical and clinical data. The FDA also requires manufacturers to comply with good manufacturing practices (GMP) to ensure the quality and consistency of their products. The regulatory pathway for bioresorbable pacemakers is still evolving, and the FDA is likely to require extensive clinical data to demonstrate their safety and efficacy. Similar regulatory frameworks exist in other countries, such as the European Union, where medical devices are regulated under the Medical Device Regulation (MDR). The MDR requires manufacturers to obtain a CE mark before they can market their devices in the EU. The regulatory landscape for medical devices is constantly evolving, and manufacturers must stay abreast of the latest requirements to ensure compliance. The complexities of regulatory approval often drive up the cost of developing and commercializing new pacemaker technologies, posing a challenge for smaller companies and innovators.
Many thanks to our sponsor Esdebe who helped us prepare this research report.
6. Comparative Analysis of Pacemaker Technologies
Each type of pacemaker technology has its own set of advantages and disadvantages. A comparative analysis is essential for understanding the trade-offs involved in selecting the appropriate pacing system for a given patient.
| Feature | Traditional Pacemaker | Leadless Pacemaker | Bioresorbable Pacemaker | Light-Activated Pacemaker |
|———————-|———————–|——————–|————————–|—————————|
| Pacing Modes | Single, Dual, Biventricular | Single Ventricular | Single Ventricular | Potentially Versatile |
| Lead-Related Complications | Yes | No | No | No |
| Longevity | 5-10 years | 5-8 years | Weeks/Months | Potentially Long-lasting |
| Patient Suitability | Broad | Limited | Temporary Pacing Only | Limited (Research Only) |
| Cost | Moderate | High | High (Early Stage) | High (Early Stage) |
| Retrieval | Lead Extraction Required | Complex | Not Required | N/A |
| MRI Compatibility | Variable | Generally Safe | Not Applicable | N/A |
| Power Source | Lithium Battery | Lithium Battery | Biodegradable Battery/Wireless | Optical Source |
The choice of pacemaker technology should be based on a careful assessment of the patient’s clinical needs, risk factors, and preferences. Traditional pacemakers remain the workhorse of cardiac pacing, offering versatility and long-term reliability. Leadless pacemakers provide an alternative for patients who are at high risk of lead-related complications. Bioresorbable pacemakers offer a promising solution for temporary pacing needs, eliminating the need for device retrieval. The advent of leadless pacemakers has shifted the treatment landscape, highlighting the importance of patient-specific factors such as anatomy and comorbidities in device selection. As bioresorbable and light-activated technologies mature, they may offer even more personalized and less invasive pacing options.
Many thanks to our sponsor Esdebe who helped us prepare this research report.
7. Future Directions
The field of cardiac pacing is constantly evolving, with ongoing research focused on developing new and improved pacing technologies. Some of the key areas of future research include:
- Wireless Energy Transfer: Wireless energy transfer could eliminate the need for batteries in pacemakers, extending their lifespan and reducing the risk of battery depletion. This technology involves transmitting energy wirelessly from an external source to the pacemaker, using inductive coupling or other methods. Advanced wireless power transfer techniques, such as focused ultrasound, could further improve efficiency and range.
- Advanced Materials: Research into new materials is crucial for improving the biocompatibility, durability, and functionality of pacemakers. This includes the development of new biodegradable polymers, conductive polymers, and nanomaterials. The integration of flexible and stretchable electronics could also enable the creation of more conformable and less invasive pacemakers.
- Closed-Loop Pacing: Closed-loop pacing systems use physiological sensors to continuously monitor the patient’s condition and adjust the pacing parameters accordingly. This can optimize pacing therapy and improve patient outcomes. Examples of closed-loop pacing systems include those that monitor blood pressure, respiration, or metabolic demand.
- Personalized Pacing Algorithms: The development of personalized pacing algorithms that are tailored to the individual patient’s needs could further improve the effectiveness of pacing therapy. This involves using data analytics and machine learning to optimize pacing parameters based on the patient’s clinical characteristics and response to therapy. The integration of artificial intelligence could enable the development of adaptive pacing algorithms that learn from patient data and continuously improve their performance.
- Miniaturization and Integration: Further miniaturization and integration of pacemaker components could lead to the development of even smaller and less invasive devices. This includes the development of micro-scale sensors, actuators, and power sources. The integration of these components into a single chip could enable the creation of truly implantable and self-contained pacing systems. Micro-electromechanical systems (MEMS) technology is also playing an increasingly important role in developing smaller and more sophisticated sensors for cardiac pacing.
Many thanks to our sponsor Esdebe who helped us prepare this research report.
8. Conclusion
Cardiac pacing technology has undergone remarkable advancements, transforming the treatment of bradyarrhythmias. From the early days of fixed-rate pacing to the current era of leadless and bioresorbable systems, the field has consistently pushed the boundaries of innovation. Traditional transvenous pacemakers remain a mainstay of therapy, offering versatility and long-term reliability. Leadless pacemakers provide a valuable alternative for patients at risk of lead-related complications. Bioresorbable pacemakers hold promise for temporary pacing needs, and light-activated pacemakers represent a potentially revolutionary approach to cardiac stimulation. As research continues, we can expect to see further advancements in pacing technology, leading to more personalized, less invasive, and more effective therapies for patients with heart rhythm disorders. The development of these advanced pacing technologies requires a multidisciplinary approach, involving collaboration between engineers, clinicians, and scientists. Furthermore, ethical considerations surrounding the use of these devices, such as data privacy and equitable access, must be carefully addressed as the technology continues to evolve.
Many thanks to our sponsor Esdebe who helped us prepare this research report.
References
[1] Furman, S., & Schwedel, J. B. (1959). An Intracardiac Pacemaker for Stokes-Adams Seizures. New England Journal of Medicine, 261(19), 943-948.
[2] Reddy, V. Y., et al. (2015). Transcatheter pacing system vs. traditional transvenous pacing for bradycardia. Circulation, 131(21), 1856-1864.
[3] Kang, S. K., et al. (2016). Dissolvable electronics for transient biomedical implants. Nature materials, 15(3), 316-322.
[4] Bruegmann, D., & Schneider, J. W. (2020). Optogenetic control of cardiac rhythm. Journal of the American College of Cardiology, 75(15), 1825-1835.
Wireless energy transfer? So, no more panicking when the pacemaker battery indicator starts blinking? Sign me up for the beta testing!
That’s exactly the hope! Wireless energy transfer could really change the game, making those battery anxieties a thing of the past. We’re still in the early stages, but the potential is definitely there to improve device longevity and patient peace of mind. Thanks for highlighting that!
Editor: MedTechNews.Uk
Thank you to our Sponsor Esdebe
Light-activated pacemakers, eh? So, finally, we can say our hearts are *literally* glowing with health. Wonder if they’ll come with a dimmer switch for romantic moments?
That’s a fun thought! A dimmer switch might be a bit much, but the potential for finely tuned control with light-activated pacemakers is definitely there. Imagine personalized adjustments based on activity level or even emotional state. It opens up some exciting possibilities for truly responsive cardiac care.
Editor: MedTechNews.Uk
Thank you to our Sponsor Esdebe