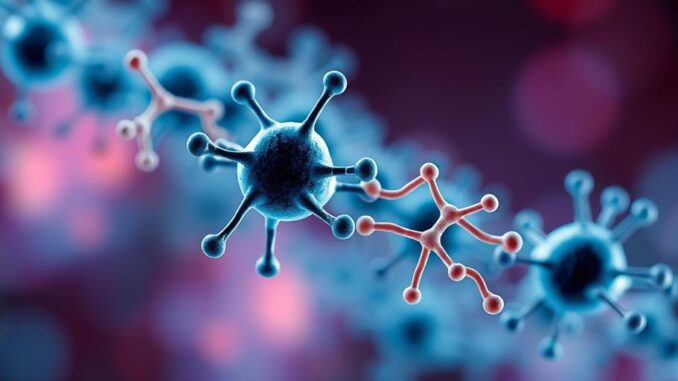
Abstract
Agonists, pharmacological agents that bind to receptors and elicit a biological response, represent a cornerstone of modern therapeutics. This report provides a comprehensive overview of agonist pharmacology, extending beyond the well-trodden path of Glucagon-Like Peptide-1 (GLP-1) agonists to encompass a broader perspective on their diverse mechanisms of action, clinical applications, and emerging research frontiers. We delve into the fundamental principles of receptor activation, signaling pathways, and the factors governing agonist efficacy and potency. The report examines various classes of agonists, including those targeting G protein-coupled receptors (GPCRs), receptor tyrosine kinases (RTKs), and nuclear receptors, highlighting their specific effects on physiological processes and disease states. We analyze clinical trial data and real-world evidence to evaluate the therapeutic potential of agonists in a range of conditions, from metabolic disorders and cardiovascular diseases to neurological disorders and cancer. Furthermore, we discuss the challenges associated with agonist-based therapies, such as receptor desensitization, tolerance, and adverse effects, and explore strategies for optimizing treatment outcomes. Finally, we outline future research directions, including the development of novel agonists with improved selectivity, efficacy, and safety profiles, as well as the exploration of personalized approaches to agonist therapy.
Many thanks to our sponsor Esdebe who helped us prepare this research report.
1. Introduction
In the realm of pharmacology, agonists stand as fundamental tools for modulating cellular function and treating a wide array of diseases. Their ability to bind to specific receptors and trigger downstream signaling cascades makes them invaluable therapeutic agents. While much attention has been focused on specific agonist classes like GLP-1 agonists due to their success in treating diabetes and obesity, a broader understanding of agonist pharmacology is crucial for advancing therapeutic innovation. This report aims to provide a comprehensive overview of agonists, encompassing their mechanisms of action, diverse applications, and future research directions.
At its core, agonist pharmacology revolves around the concept of receptor occupancy and activation. Receptors, typically membrane-bound proteins, serve as the initial point of interaction for agonists. Upon binding, the agonist induces a conformational change in the receptor, initiating a signaling cascade that ultimately leads to a biological response. The magnitude of this response is determined by several factors, including the agonist’s affinity for the receptor, its intrinsic efficacy (the ability to activate the receptor), and the density of receptors present on the target cell. Understanding these fundamental principles is essential for designing and optimizing agonist-based therapies.
This report will delve into the mechanisms of action of various classes of agonists, including those targeting GPCRs, RTKs, and nuclear receptors. Each receptor class possesses unique signaling pathways and downstream effects, which will be thoroughly explored. We will also examine the clinical applications of agonists in a range of diseases, from metabolic disorders and cardiovascular diseases to neurological disorders and cancer. By analyzing clinical trial data and real-world evidence, we will evaluate the therapeutic potential of agonists and identify areas where further research is needed.
Many thanks to our sponsor Esdebe who helped us prepare this research report.
2. Mechanisms of Action: A Deeper Dive
The efficacy of an agonist hinges upon its mechanism of action, specifically its ability to bind to and activate its target receptor. This section dissects the intricacies of receptor activation and the subsequent signaling cascades, highlighting the diverse pathways employed by different classes of agonists.
2.1 G Protein-Coupled Receptors (GPCRs)
GPCRs, the largest family of membrane receptors, represent a major target for agonist-based therapies. Upon agonist binding, GPCRs undergo a conformational change that activates intracellular G proteins. These G proteins, in turn, modulate the activity of downstream effector proteins, such as adenylyl cyclase and phospholipase C, leading to changes in intracellular cAMP and calcium levels. These second messengers trigger a cascade of phosphorylation events, ultimately altering gene expression and cellular function. The complexity of GPCR signaling extends beyond the canonical G protein-dependent pathways, with evidence suggesting that GPCRs can also activate other signaling molecules, such as β-arrestins, which can mediate both signaling and receptor internalization. An example of this is beta adrenergic receptors which are activated by beta-adrenergic agonists. The nature of these interactions is an active area of research. Some of the mechanisms behind the interaction are not well understood. The implication of GPCRs in a vast array of physiological processes, from neurotransmission to hormone secretion, underscores their importance as therapeutic targets.
2.2 Receptor Tyrosine Kinases (RTKs)
RTKs are transmembrane receptors that possess intrinsic tyrosine kinase activity. Agonist binding to RTKs induces receptor dimerization and autophosphorylation of tyrosine residues. These phosphorylated tyrosine residues serve as docking sites for intracellular signaling proteins, such as SH2 domain-containing proteins, which activate downstream signaling pathways like the Ras-MAPK and PI3K-Akt pathways. These pathways play crucial roles in cell growth, proliferation, and differentiation. RTKs are frequently dysregulated in cancer, making them attractive targets for targeted therapies. Epidermal growth factor receptor (EGFR) and vascular endothelial growth factor receptor (VEGFR) are two key examples of RTKs that are often targeted in cancer treatment. For example, anti-EGFR agents such as gefitinib act as EGFR inhibitors, not agonists. However, the study of native ligands and downstream signalling from RTK stimulation is vital for the future development of agonist therapies in this area.
2.3 Nuclear Receptors
Nuclear receptors are intracellular receptors that regulate gene expression. Agonists for nuclear receptors, such as steroid hormones and thyroid hormones, diffuse across the cell membrane and bind to their respective receptors in the cytoplasm or nucleus. The agonist-receptor complex then translocates to the nucleus and binds to specific DNA sequences called hormone response elements (HREs), modulating the transcription of target genes. Nuclear receptors play critical roles in development, metabolism, and reproduction. The mechanisms of action of agonists of nuclear receptors is therefore very important in the treatment of several metabolic and endocrine diseases.
2.4 Receptor Desensitization and Tolerance
Prolonged exposure to agonists can lead to receptor desensitization and tolerance, diminishing the therapeutic efficacy of these drugs. Receptor desensitization occurs when the receptor becomes less responsive to agonist stimulation, often due to phosphorylation or internalization of the receptor. Tolerance, on the other hand, refers to a decrease in the overall response to the agonist, which can be caused by a reduction in receptor expression or changes in downstream signaling pathways. Understanding the mechanisms underlying receptor desensitization and tolerance is crucial for developing strategies to overcome these limitations. Strategies such as intermittent dosing, the use of biased agonists (which selectively activate certain signaling pathways), and the development of allosteric modulators (which alter receptor conformation and responsiveness) are being actively explored.
Many thanks to our sponsor Esdebe who helped us prepare this research report.
3. Agonist Classes: A Comparative Analysis
This section provides a comparative analysis of different classes of agonists, highlighting their specific mechanisms of action, clinical applications, and advantages and disadvantages.
3.1 Beta-Adrenergic Agonists
Beta-adrenergic agonists, such as albuterol and salmeterol, stimulate beta-adrenergic receptors, which are GPCRs that activate adenylyl cyclase and increase intracellular cAMP levels. These agonists are primarily used to treat asthma and chronic obstructive pulmonary disease (COPD) by relaxing airway smooth muscle and opening up the airways. While effective in relieving bronchospasm, beta-adrenergic agonists can cause side effects such as tachycardia, tremors, and anxiety. Additionally, chronic use of beta-adrenergic agonists can lead to receptor desensitization and reduced efficacy.
3.2 Opioid Agonists
Opioid agonists, such as morphine and fentanyl, bind to opioid receptors in the brain and spinal cord, producing analgesia and euphoria. These agonists are widely used to manage acute and chronic pain. However, opioid agonists are highly addictive and can cause serious side effects such as respiratory depression and constipation. The opioid crisis has highlighted the urgent need for safer and more effective pain management strategies.
3.3 Dopamine Agonists
Dopamine agonists, such as levodopa and pramipexole, stimulate dopamine receptors in the brain, improving motor function in patients with Parkinson’s disease. Levodopa is a precursor to dopamine that can cross the blood-brain barrier and be converted into dopamine in the brain. Pramipexole, on the other hand, directly stimulates dopamine receptors. Dopamine agonists can cause side effects such as nausea, hallucinations, and impulse control disorders.
3.4 Glucocorticoid Agonists
Glucocorticoid agonists, such as prednisone and dexamethasone, bind to glucocorticoid receptors in the cytoplasm and regulate gene expression. These agonists are used to treat a wide range of inflammatory and autoimmune diseases by suppressing the immune system. However, glucocorticoid agonists can cause serious side effects such as weight gain, osteoporosis, and increased susceptibility to infection. These side effects are often dose and duration-dependent, emphasizing the need for careful monitoring and dose titration.
3.5 GLP-1 Receptor Agonists
GLP-1 receptor agonists, such as semaglutide and liraglutide, stimulate GLP-1 receptors in the pancreas, brain, and gastrointestinal tract. These agonists enhance insulin secretion, suppress glucagon secretion, slow gastric emptying, and reduce appetite. GLP-1 receptor agonists are primarily used to treat type 2 diabetes and obesity. Recent studies have also suggested that GLP-1 receptor agonists may have neuroprotective effects and could potentially reduce the risk of dementia. However, GLP-1 receptor agonists can cause side effects such as nausea, vomiting, and diarrhea. While generally well-tolerated, rare but serious side effects such as pancreatitis have also been reported.
3.6 Agonist Choice: Context Matters
The selection of an appropriate agonist for a given clinical scenario is highly dependent on several factors including the receptor target, disease context, and patient-specific characteristics. For example, the choice between a short-acting and long-acting beta-adrenergic agonist for asthma management depends on the frequency and severity of symptoms, as well as patient adherence. Similarly, the selection of an opioid agonist for pain management should take into account the severity of pain, the risk of addiction, and the patient’s medical history. Understanding the pharmacological properties of different agonists, as well as the individual needs of the patient, is essential for optimizing treatment outcomes.
Many thanks to our sponsor Esdebe who helped us prepare this research report.
4. Clinical Applications of Agonists
Agonists play a pivotal role in treating a wide spectrum of diseases, from metabolic disorders to neurological conditions. This section explores the diverse clinical applications of agonists, highlighting their therapeutic benefits and limitations.
4.1 Metabolic Disorders
Agonists are widely used in the treatment of metabolic disorders such as diabetes and obesity. As discussed earlier, GLP-1 receptor agonists have revolutionized the treatment of type 2 diabetes by improving glycemic control, reducing body weight, and potentially offering cardiovascular benefits. Other agonists used in the treatment of metabolic disorders include thiazolidinediones (TZDs), which are PPARγ agonists that improve insulin sensitivity. However, TZDs have been associated with side effects such as weight gain, edema, and heart failure. The relative benefits and risks of different agonists must be carefully weighed when making treatment decisions.
4.2 Cardiovascular Diseases
Agonists are also used in the treatment of cardiovascular diseases such as heart failure and hypertension. Beta-adrenergic agonists, such as dobutamine, can be used to increase cardiac output in patients with heart failure. However, chronic use of beta-adrenergic agonists can lead to receptor desensitization and increased mortality. Alpha-adrenergic agonists, such as clonidine, can be used to lower blood pressure in patients with hypertension. However, alpha-adrenergic agonists can cause side effects such as drowsiness and dry mouth. Angiotensin II receptor blockers (ARBs) are another class of drugs that are widely used to treat hypertension and heart failure. While not strictly agonists, ARBs block the effects of angiotensin II by binding to its receptor, effectively preventing its activation. They are often preferred over ACE inhibitors due to a lower risk of cough.
4.3 Neurological Disorders
Agonists are used in the treatment of neurological disorders such as Parkinson’s disease, Alzheimer’s disease, and multiple sclerosis. Dopamine agonists, as discussed earlier, are used to improve motor function in patients with Parkinson’s disease. Acetylcholinesterase inhibitors, such as donepezil, increase the levels of acetylcholine in the brain and can improve cognitive function in patients with Alzheimer’s disease. Interferon beta, an agonist for interferon receptors, is used to reduce the frequency and severity of relapses in patients with multiple sclerosis. The effectiveness of these agonists can vary depending on the specific disease and the individual patient. Furthermore, many neurological disorders are complex and multifactorial, requiring a combination of pharmacological and non-pharmacological interventions.
4.4 Cancer
Agonists also have applications in cancer therapy. For example, tamoxifen, a selective estrogen receptor modulator (SERM), acts as an estrogen receptor agonist in some tissues and an estrogen receptor antagonist in others. It is used to treat breast cancer by blocking the effects of estrogen on breast cancer cells. Gonadotropin-releasing hormone (GnRH) agonists, such as leuprolide, are used to treat prostate cancer by suppressing the production of testosterone. Agonists can also be used to stimulate the immune system to fight cancer cells. For example, interferon alpha is used to treat certain types of cancer by activating immune cells and enhancing their ability to kill cancer cells. The role of agonists in cancer therapy is constantly evolving as new targets and strategies are discovered.
4.5 Considerations for Long-Term Agonist Use
The long-term use of agonists can be associated with a variety of potential benefits and risks. On the one hand, long-term agonist therapy can provide sustained relief from symptoms and improve quality of life for patients with chronic diseases. On the other hand, long-term agonist use can lead to receptor desensitization, tolerance, and adverse effects. It is therefore essential to carefully weigh the potential benefits and risks of long-term agonist therapy and to monitor patients closely for signs of adverse effects. Strategies to mitigate the risks of long-term agonist use include intermittent dosing, the use of lower doses, and the development of alternative therapies.
Many thanks to our sponsor Esdebe who helped us prepare this research report.
5. Challenges and Future Directions
While agonists have revolutionized the treatment of many diseases, several challenges remain. Receptor desensitization and tolerance, as discussed earlier, can limit the long-term efficacy of agonists. Furthermore, many agonists have off-target effects that can lead to adverse effects. Developing more selective and effective agonists is a major goal of drug discovery research.
5.1 Biased Agonism
Biased agonism, a phenomenon where agonists selectively activate certain signaling pathways downstream of a receptor, represents a promising avenue for developing safer and more effective drugs. By selectively activating beneficial signaling pathways while avoiding those associated with adverse effects, biased agonists can offer improved therapeutic profiles. Research in this area is rapidly advancing, with the identification of biased agonists for a variety of receptors, including GPCRs and RTKs.
5.2 Allosteric Modulation
Allosteric modulators are compounds that bind to a receptor at a site distinct from the orthosteric (agonist-binding) site, altering the receptor’s conformation and responsiveness to agonists. Allosteric modulators can either enhance (positive allosteric modulators) or inhibit (negative allosteric modulators) the effects of agonists. Allosteric modulation offers several advantages over traditional orthosteric agonists, including the ability to fine-tune receptor activity and the potential for greater receptor selectivity. This is an emerging area of research.
5.3 Personalized Agonist Therapy
Personalized medicine, tailoring treatment to the individual patient based on their genetic makeup, lifestyle, and environmental factors, is transforming healthcare. In the context of agonist therapy, personalized medicine involves identifying patients who are most likely to benefit from a particular agonist, as well as predicting their risk of adverse effects. Pharmacogenomics, the study of how genes affect a person’s response to drugs, plays a crucial role in personalized agonist therapy. By identifying genetic variations that influence drug metabolism, receptor expression, and signaling pathways, clinicians can optimize agonist dosing and selection to maximize therapeutic benefits and minimize risks. For example, variations in genes encoding drug-metabolizing enzymes, such as cytochrome P450 enzymes, can significantly affect drug levels and efficacy. Personalized agonist therapy is an exciting area of research with the potential to improve treatment outcomes and reduce healthcare costs.
5.4 Novel Delivery Systems
Novel drug delivery systems, such as nanoparticles, liposomes, and microencapsulation, can improve the bioavailability, targeting, and controlled release of agonists. These delivery systems can protect agonists from degradation, enhance their penetration into target tissues, and prolong their duration of action. Nanoparticles, in particular, offer great promise for targeted drug delivery to specific cells or tissues. By functionalizing nanoparticles with targeting ligands, such as antibodies or peptides, it is possible to deliver agonists selectively to cancer cells or immune cells, minimizing off-target effects. This can lead to the use of much lower doses of the drug, which is essential for agonists with serious side effects at normal doses.
Many thanks to our sponsor Esdebe who helped us prepare this research report.
6. Conclusion
Agonists represent a cornerstone of modern therapeutics, offering a diverse array of mechanisms for modulating cellular function and treating a wide range of diseases. This report has provided a comprehensive overview of agonist pharmacology, encompassing their mechanisms of action, clinical applications, and emerging research frontiers. While agonists have revolutionized the treatment of many diseases, challenges remain in overcoming receptor desensitization, tolerance, and off-target effects. However, ongoing research in areas such as biased agonism, allosteric modulation, personalized medicine, and novel delivery systems holds great promise for developing safer and more effective agonist-based therapies. The future of agonist pharmacology is bright, with the potential to transform the treatment of many diseases and improve the lives of millions of people.
Many thanks to our sponsor Esdebe who helped us prepare this research report.
References
- Kenakin, T. P. (2019). Pharmacology in Drug Discovery and Development: Understanding Drug Response. Academic Press.
- Limbird, L. E. (2012). Medical Physiology: A Cellular and Molecular Approach. Lippincott Williams & Wilkins.
- Rang, H. P., Dale, M. M., Ritter, J. M., & Flower, R. J. (2015). Rang and Dale’s Pharmacology. Churchill Livingstone.
- Rosenbaum, D. M., Rasmussen, S. G., & Kobilka, B. K. (2009). GPCR structure and function. Nature, 459(7245), 356-363.
- Schwartz, T. W., Holst, B., Rosenkilde, M. M., & Unett, B. (2010). Biased agonism: an emerging paradigm for drug discovery. Nature Reviews Drug Discovery, 9(3), 205-222.
- Stern, A. M., & Flockhart, D. A. (2017). Pharmacogenomics in the management of cardiovascular disease. Nature Reviews Cardiology, 14(1), 1-16.
- Thal, D. M., & Valant, C. (2016). Allosteric drug discovery targeting G protein-coupled receptors. Trends in Pharmacological Sciences, 37(11), 974-986.
- Travers, J. B. (2017). Glucocorticoids: mechanisms of action. UpToDate. Retrieved from [UpToDate website]
- Wexler, I. D. (2010). Review of dopamine agonists in Parkinson’s disease. Neurologic Clinics, 28(4), 841-853.
The section on personalized agonist therapy highlights the potential of pharmacogenomics. Exploring how AI and machine learning can accelerate the identification of relevant genetic markers for individualizing treatment regimens would be a valuable area for further investigation.
That’s a great point! The integration of AI and machine learning could definitely revolutionize the field of personalized agonist therapy. Imagine the possibilities of rapidly screening vast datasets to pinpoint those crucial genetic markers. It opens up exciting avenues for more precise and effective treatment strategies. Thanks for highlighting this important aspect!
Editor: MedTechNews.Uk
Thank you to our Sponsor Esdebe
So, agonists are like the key to a car, but sometimes the car is already running and the key just makes it stall? Is that why “receptor desensitization” is a thing? Are we looking at keys that only work on Tuesdays next?
That’s a fantastic analogy! You’re right, sometimes adding an agonist when the system is already highly active can indeed lead to a paradoxical effect, like stalling the engine. Receptor desensitization is one reason, as the system tries to maintain balance. The concept of keys that only work on Tuesdays brings to mind the complex temporal dynamics of receptor expression and signaling!
Editor: MedTechNews.Uk
Thank you to our Sponsor Esdebe