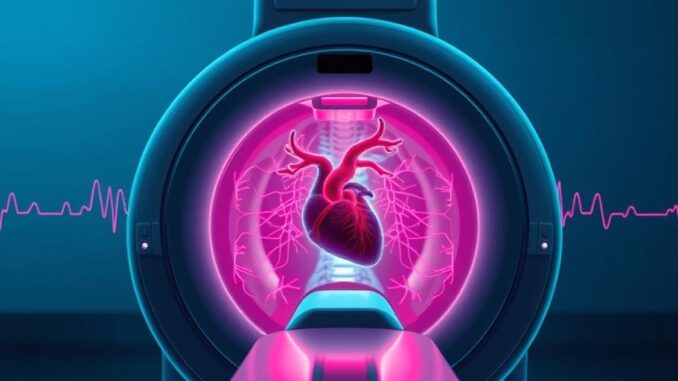
Abstract
4D flow magnetic resonance imaging (MRI), a technique that captures time-resolved, three-dimensional blood flow information, has revolutionized cardiovascular imaging. While its initial applications centered on the assessment of aortic disease and congenital heart defects, the versatility of 4D flow MRI is now extending beyond the traditional boundaries of cardiology. This report explores the emerging applications of 4D flow MRI in diverse physiological systems, including cerebrovascular hemodynamics, liver perfusion, renal flow dynamics, and musculoskeletal biomechanics. We delve into the technical adaptations required for these novel applications, highlighting the advantages offered by 4D flow MRI compared to existing imaging modalities. Furthermore, we address the challenges associated with data acquisition and analysis in these new contexts, considering factors such as patient motion, signal-to-noise ratio, and the complexity of flow patterns. Finally, we examine the potential impact of these emerging applications on clinical diagnosis, treatment planning, and our understanding of human physiology, while also discussing future directions and research opportunities.
Many thanks to our sponsor Esdebe who helped us prepare this research report.
1. Introduction
4D flow MRI is a powerful non-invasive imaging technique that provides a comprehensive assessment of blood flow dynamics. By encoding velocity information in three spatial dimensions over time, it allows for the quantification of various hemodynamic parameters, including velocity, flow rate, wall shear stress (WSS), and pulse wave velocity (PWV). Initially developed for cardiovascular applications, such as evaluating aortic stenosis, congenital heart disease, and pulmonary hypertension [1], its ability to visualize and quantify complex flow patterns has sparked interest in extending its use to other areas of the body. This report aims to explore the expanding horizons of 4D flow MRI, moving beyond the well-established cardiovascular applications. We will examine its potential in various physiological systems and highlight the technological and analytical advancements that are enabling this expansion. We will also address the current limitations and future directions of this evolving field.
Many thanks to our sponsor Esdebe who helped us prepare this research report.
2. Technical Considerations for Non-Cardiovascular Applications
Applying 4D flow MRI to non-cardiovascular systems requires careful consideration of several technical factors to ensure optimal image quality and accurate flow quantification. These considerations often differ significantly from those encountered in cardiovascular imaging.
2.1 Pulse Sequence Optimization
The specific pulse sequence parameters used in 4D flow MRI need to be tailored to the anatomical region being imaged. For example, when imaging the brain, the use of shorter echo times (TE) is crucial to minimize signal loss due to T2* decay. Furthermore, the velocity encoding (Venc) values must be carefully selected to avoid aliasing, which occurs when the actual velocity exceeds the Venc value. In regions with slow or complex flow patterns, lower Venc values may be necessary, while higher Venc values are required for areas with faster flow [2]. Motion-sensitized gradients can also be adjusted to better capture subtle movements within a specific tissue or organ.
2.2 Motion Correction
Motion artifacts are a significant challenge in MRI, particularly in abdominal and pelvic imaging. Respiratory motion, bowel peristalsis, and patient movement can all degrade image quality and introduce errors in flow quantification. Various motion correction techniques can be employed to mitigate these effects. Navigator echoes, which track the position of the diaphragm during respiration, can be used to retrospectively correct for respiratory motion [3]. Prospective motion correction techniques, which adjust the MRI gradients in real-time based on motion information, can also be used. Additionally, breath-hold techniques can be used to acquire images during periods of minimal motion, although this may not be feasible for all patients or anatomical regions.
2.3 Spatial Resolution and Coverage
The required spatial resolution and coverage depend on the size and complexity of the region being imaged. For example, when imaging the liver, a larger field of view (FOV) is necessary to cover the entire organ. However, this may compromise spatial resolution. Optimizing the spatial resolution and coverage is a trade-off that must be carefully considered based on the specific clinical question. Recent advances in compressed sensing and parallel imaging techniques have enabled faster acquisition times and improved spatial resolution [4]. These techniques allow for undersampling of the k-space data, which reduces the scan time, while still maintaining acceptable image quality.
2.4 Data Acquisition and Reconstruction
Advanced data acquisition and reconstruction techniques are crucial for high-quality 4D flow MRI. k-t acceleration methods, such as k-t BLAST and k-t SENSE, can significantly reduce scan times by acquiring fewer data points in both k-space and time [5]. These techniques exploit the spatiotemporal correlation in the data to reconstruct high-resolution images from undersampled data. Furthermore, advanced reconstruction algorithms, such as iterative reconstruction, can improve image quality by reducing artifacts and noise. These advancements are particularly important for non-cardiovascular applications, where scan times can be longer due to the need for larger FOVs and higher spatial resolution.
Many thanks to our sponsor Esdebe who helped us prepare this research report.
3. Applications in Cerebrovascular Hemodynamics
4D flow MRI is emerging as a valuable tool for studying cerebrovascular hemodynamics in both healthy individuals and patients with various neurological disorders. It offers a non-invasive means to assess cerebral blood flow (CBF), intracranial pressure (ICP), and the biomechanics of cerebral aneurysms.
3.1 Cerebral Blood Flow and Intracranial Pressure
Traditional methods for measuring CBF, such as xenon-enhanced CT and PET, are invasive and involve ionizing radiation. 4D flow MRI offers a non-invasive alternative for quantifying CBF in different brain regions [6]. By measuring the velocity and cross-sectional area of major cerebral arteries, such as the internal carotid artery and vertebral artery, it is possible to calculate the total CBF. Furthermore, 4D flow MRI can be used to assess the pulsatility of CBF, which is related to ICP. Elevated ICP is a common complication of traumatic brain injury, hydrocephalus, and other neurological disorders. 4D flow MRI can potentially be used to monitor ICP non-invasively, avoiding the need for invasive pressure monitoring devices [7]. However, the correlation between 4D flow MRI measurements and direct ICP measurements still requires further investigation.
3.2 Cerebral Aneurysms
4D flow MRI is particularly useful for studying the hemodynamics of cerebral aneurysms. Aneurysms are weak spots in the walls of cerebral arteries that can rupture, leading to life-threatening subarachnoid hemorrhage. The risk of rupture is related to the hemodynamics within the aneurysm, including WSS, oscillatory shear index (OSI), and flow impingement [8]. 4D flow MRI can be used to visualize and quantify these hemodynamic parameters, providing valuable information for assessing the risk of rupture and guiding treatment decisions. Studies have shown that aneurysms with high WSS and OSI are more likely to rupture. 4D flow MRI can also be used to evaluate the effectiveness of aneurysm treatments, such as coiling and clipping, by assessing the changes in hemodynamics following treatment [9].
3.3 Arteriovenous Malformations (AVMs)
Cerebral AVMs are abnormal connections between arteries and veins in the brain. These can cause stroke, seizures, or hemorrhage. 4D flow MRI can visualize the complex flow patterns within AVMs and measure blood flow velocity, providing information about the potential for rupture or bleeding [10].
Many thanks to our sponsor Esdebe who helped us prepare this research report.
4. Applications in Liver Perfusion
Assessing liver perfusion is crucial for diagnosing and managing various liver diseases, including cirrhosis, hepatocellular carcinoma (HCC), and liver transplantation. 4D flow MRI can provide a comprehensive assessment of liver perfusion, including portal venous flow, hepatic arterial flow, and hepatic venous outflow [11].
4.1 Liver Cirrhosis
In patients with cirrhosis, the liver parenchyma is replaced by fibrotic tissue, which impairs blood flow. 4D flow MRI can be used to quantify the changes in liver perfusion associated with cirrhosis, such as decreased portal venous flow and increased hepatic arterial flow. It can also be used to assess the severity of portal hypertension, a common complication of cirrhosis. By measuring the velocity and cross-sectional area of the portal vein, it is possible to calculate the portal venous pressure [12]. The ability to non-invasively assess the hemodynamic changes associated with cirrhosis is a significant advantage over traditional invasive methods, such as liver biopsy and hepatic venous pressure gradient measurements.
4.2 Hepatocellular Carcinoma
HCC is the most common type of liver cancer. It is typically hypervascular, meaning that it receives a disproportionate amount of blood flow from the hepatic artery. 4D flow MRI can be used to assess the tumor’s blood supply and to evaluate the effectiveness of anti-angiogenic therapies, which aim to reduce the tumor’s blood supply. By measuring the flow velocity and volume within the tumor, it is possible to quantify the tumor’s perfusion [13]. This information can be used to predict the response to therapy and to monitor the effectiveness of treatment.
4.3 Liver Transplantation
4D flow MRI can also be used to assess the function of transplanted livers. By measuring the portal venous flow and hepatic arterial flow, it is possible to assess the viability of the transplanted liver. It can also be used to detect complications, such as hepatic artery thrombosis and portal vein stenosis [14]. Early detection of these complications is crucial for preventing graft failure.
Many thanks to our sponsor Esdebe who helped us prepare this research report.
5. Applications in Renal Flow Dynamics
The kidneys play a crucial role in regulating blood pressure and maintaining fluid and electrolyte balance. Assessing renal flow dynamics is important for diagnosing and managing various kidney diseases, including renal artery stenosis, chronic kidney disease (CKD), and renal transplant rejection.
5.1 Renal Artery Stenosis
Renal artery stenosis (RAS) is a narrowing of the renal artery, which can lead to hypertension and CKD. 4D flow MRI can be used to detect RAS and to assess its severity. By measuring the velocity and flow rate in the renal artery, it is possible to quantify the degree of stenosis [15]. Furthermore, 4D flow MRI can be used to assess the downstream effects of RAS on renal perfusion and function. This information can be used to guide treatment decisions, such as angioplasty or stenting.
5.2 Chronic Kidney Disease
CKD is a progressive loss of kidney function. 4D flow MRI can be used to assess renal perfusion in patients with CKD. Studies have shown that patients with CKD have reduced renal blood flow and increased renal vascular resistance [16]. 4D flow MRI can potentially be used to monitor the progression of CKD and to evaluate the effectiveness of treatments aimed at preserving renal function.
5.3 Renal Transplant Rejection
Renal transplant rejection is a major cause of graft failure. 4D flow MRI can be used to detect early signs of rejection. By measuring renal blood flow and perfusion, it is possible to identify changes that are indicative of rejection [17]. Early detection of rejection is crucial for initiating immunosuppressive therapy and preventing graft loss.
Many thanks to our sponsor Esdebe who helped us prepare this research report.
6. Applications in Musculoskeletal Biomechanics
While less explored than the previously mentioned applications, 4D flow MRI is showing promise in musculoskeletal biomechanics, particularly in understanding blood flow dynamics within muscles during exercise and in assessing the vascularity of bone tumors.
6.1 Muscle Perfusion During Exercise
Understanding muscle perfusion during exercise is crucial for understanding muscle fatigue, performance, and adaptation to training. 4D flow MRI can be used to measure muscle blood flow during exercise, providing insights into the regulation of blood flow to active muscles [18]. This could be useful for optimizing training programs and for understanding the pathophysiology of muscle fatigue. However, significant challenges exist in imaging during exercise, including motion artifacts and the limited amount of time available for image acquisition.
6.2 Bone Tumor Vascularity
4D flow MRI can also be used to assess the vascularity of bone tumors. Tumor vascularity is an important prognostic factor and can be used to guide treatment decisions. By measuring the blood flow within the tumor, it is possible to assess the tumor’s aggressiveness and its response to therapy [19]. Differentiating between benign and malignant bone lesions and assessing the degree of angiogenesis within them. Further research is needed to establish the clinical utility of 4D flow MRI in this area.
Many thanks to our sponsor Esdebe who helped us prepare this research report.
7. Limitations and Challenges
Despite its potential, 4D flow MRI faces several limitations and challenges that need to be addressed to realize its full clinical potential.
7.1 Scan Time
4D flow MRI scans can be time-consuming, which can be a limiting factor, particularly in patients who are unable to hold their breath or remain still for extended periods. The long scan times are due to the need to acquire data in three spatial dimensions and over time. Accelerated imaging techniques, such as compressed sensing and parallel imaging, can help to reduce scan times, but these techniques can also introduce artifacts and reduce image quality. Optimizing pulse sequence parameters and using advanced reconstruction algorithms are crucial for minimizing scan times while maintaining acceptable image quality.
7.2 Signal-to-Noise Ratio
The signal-to-noise ratio (SNR) in 4D flow MRI can be low, particularly in regions with slow or complex flow patterns. Low SNR can lead to inaccurate flow quantification and reduced image quality. Increasing the number of signal averages can improve SNR, but this also increases scan time. Using higher field strength MRI scanners (e.g., 3T or 7T) can also improve SNR. Furthermore, advanced post-processing techniques, such as denoising algorithms, can be used to improve SNR.
7.3 Data Processing and Analysis
The data processing and analysis of 4D flow MRI data can be complex and time-consuming. Specialized software is required to reconstruct the 3D velocity field and to calculate hemodynamic parameters. Furthermore, manual correction of artifacts and segmentation of vessels may be necessary. The lack of standardized data processing and analysis protocols is a barrier to the widespread adoption of 4D flow MRI. Developing automated data processing and analysis tools is crucial for improving the efficiency and reproducibility of 4D flow MRI.
7.4 Cost-Effectiveness
The cost of 4D flow MRI can be a barrier to its widespread adoption. The cost of the MRI scanner, the specialized pulse sequences, and the data processing and analysis software can be significant. Furthermore, the time required to perform and interpret 4D flow MRI scans can also contribute to the overall cost. Evaluating the cost-effectiveness of 4D flow MRI compared to other imaging modalities is important for justifying its use in clinical practice. Studies are needed to demonstrate the clinical benefits of 4D flow MRI and to show that it can improve patient outcomes.
Many thanks to our sponsor Esdebe who helped us prepare this research report.
8. Future Directions
The field of 4D flow MRI is rapidly evolving, with ongoing research focused on improving the technique and expanding its applications. Some of the key areas of future research include:
8.1 Development of New Pulse Sequences
Researchers are developing new pulse sequences that can improve image quality, reduce scan time, and enhance the sensitivity to slow flow. Non-Cartesian acquisition schemes, such as spiral and radial acquisitions, can be less sensitive to motion artifacts than traditional Cartesian acquisitions. Furthermore, advanced encoding strategies, such as flow-sensitive alternating inversion recovery (FAIR), can improve the sensitivity to slow flow. Further developments in pulse sequences are needed to overcome the limitations of current 4D flow MRI techniques.
8.2 Artificial Intelligence and Machine Learning
Artificial intelligence (AI) and machine learning (ML) are being used to automate data processing and analysis, improve image quality, and develop new diagnostic tools. AI algorithms can be trained to automatically segment vessels, correct for artifacts, and calculate hemodynamic parameters. ML algorithms can be used to predict the risk of disease progression or treatment response based on 4D flow MRI data. The integration of AI and ML into 4D flow MRI has the potential to significantly improve its efficiency and clinical utility.
8.3 Multimodal Imaging
Combining 4D flow MRI with other imaging modalities, such as PET, CT, and ultrasound, can provide complementary information and improve diagnostic accuracy. For example, combining 4D flow MRI with PET can provide information about both blood flow and metabolism. Combining 4D flow MRI with CT can provide information about both hemodynamics and anatomical structure. Multimodal imaging has the potential to provide a more comprehensive assessment of disease and to improve patient outcomes.
8.4 Point-of-Care Applications
Developing point-of-care 4D flow MRI applications could significantly expand its accessibility and impact. Portable MRI scanners and simplified data processing algorithms could enable the use of 4D flow MRI in resource-limited settings and in emergency situations. Point-of-care 4D flow MRI could be used to rapidly assess stroke, trauma, and other critical conditions. This requires miniaturization and automation of the technology, along with robust algorithms capable of operating with lower SNR data.
Many thanks to our sponsor Esdebe who helped us prepare this research report.
9. Conclusion
4D flow MRI is a versatile and powerful imaging technique that is rapidly expanding beyond its traditional cardiovascular applications. Its ability to visualize and quantify complex flow patterns has made it a valuable tool for studying cerebrovascular hemodynamics, liver perfusion, renal flow dynamics, and musculoskeletal biomechanics. While significant challenges remain, ongoing research is focused on improving the technique and expanding its clinical utility. With continued advancements in pulse sequence design, data processing, and AI/ML integration, 4D flow MRI has the potential to revolutionize the diagnosis and management of a wide range of diseases. Its non-invasive nature, combined with its comprehensive hemodynamic assessment capabilities, positions it as a key imaging modality for future clinical and research applications. The cost-effectiveness of the technique will need to be shown with more research and analysis.
Many thanks to our sponsor Esdebe who helped us prepare this research report.
References
[1] Markl, M., Frydrychowicz, A., Kozerke, S., Hope, M., & Wieben, O. (2012). 4D flow MRI. Journal of Magnetic Resonance Imaging, 36(5), 1015-1036.
[2] Dyverfeldt, P., Hope, M. D., Wieben, O., & Markl, M. (2015). Quantitative cardiovascular magnetic resonance 4D flow analysis: Principles and clinical applications. Journal of Cardiovascular Magnetic Resonance, 17(1), 1-18.
[3] Larson, A. C., White, R. D., Laub, G., McVeigh, E. R., & Li, D. (2004). Self-gated cardiac cine MRI. Magnetic Resonance in Medicine, 51(1), 93-102.
[4] Lustig, M., Donoho, D., & Pauly, J. M. (2007). Sparse MRI: The application of compressed sensing for rapid MR imaging. Magnetic Resonance in Medicine, 58(6), 1182-1195.
[5] Tsao, J., Boesiger, P., & Pruessmann, K. P. (2003). k-t BLAST and k-t SENSE for highly accelerated time-resolved MRI. Magnetic Resonance in Medicine, 50(5), 1031-1042.
[6] Baledent, O., Mary, F., Serres, I., & Pelage, A. (2001). Velocity encoded cine MR imaging of cerebral blood flow: Feasibility and normal values. Journal of Magnetic Resonance Imaging, 13(4), 568-576.
[7] Alperin, N. J., Lee, S. H., Bagci, A. M., & Loevner, L. A. (2000). MR-measured dynamic compliance of the intracranial space. American Journal of Neuroradiology, 21(8), 1391-1402.
[8] Cebral, J. R., Castro, M. A., Putman, C. M., Raschi, M., & Burgess, J. E. (2011). Characterization of hemodynamics in cerebral aneurysms from 3D rotational angiography. American Journal of Neuroradiology, 32(2), 256-264.
[9] Janiga, G., & Behr, M. (2012). Patient-specific computational hemodynamics for the treatment of intracranial aneurysms. International Journal for Numerical Methods in Biomedical Engineering, 28(6-7), 714-733.
[10] Schnell, S., Geiger, J., Markl, M., Herzer, L., Berkefeld, J., & Bendszus, M. (2014). Intracranial arteriovenous malformations: Influence of angioarchitecture and hemodynamics on clinical presentation. Neuroradiology, 56(4), 253-260.
[11] Ehman, E. C., Riederer, S. J., Felmlee, J. P., & Grimm, R. C. (1990). Flow imaging of the portal venous system with gradient-recalled echo techniques. Radiology, 176(1), 205-210.
[12] Lalwani, N., McCullough, L. B., Sirlin, C. B., & Loomba, R. (2013). Noninvasive assessment of portal hypertension: Current and emerging imaging modalities. World Journal of Gastroenterology, 19(26), 4009.
[13] Sahani, D. V., Kalva, S. P., Hahn, P. F., & Saini, S. (2007). Advanced MR imaging of the liver. Radiology, 245(1), 13-27.
[14] Hussain, S. M., Semelka, R. C., van den Bosch, M. A., Cho, K. J., & Aisen, A. M. (2004). Postoperative assessment of liver transplants. Radiology, 231(2), 275-290.
[15] Kim, D. W., Prince, M. R., & Inglese, G. (2003). Renal artery stenosis: Diagnosis by spiral CT angiography versus Doppler US. Radiology, 227(1), 99-106.
[16] Tanaka, A., Fujita, T., Hayashida, Y., Yoshimitsu, K., & Honda, H. (2016). Renal perfusion assessed by arterial spin labeling MRI in patients with chronic kidney disease. European Radiology, 26(7), 2218-2225.
[17] Hosseini-Nik, A., Keogh, C. F., Sadowski, E. A., Kennedell, J. S., Shapiro, B., & Grist, T. M. (2011). The role of magnetic resonance angiography in the evaluation of renal transplant complications. Transplantation, 92(7), 731-740.
[18] Tschakovsky, M. E., & Sheriff, D. D. (2004). Dynamic control of skin blood flow. Journal of Applied Physiology, 96(3), 1061-1070.
[19] Van Rijswijk, C. S., Geirnaerdt, M. J., Hogendoorn, P. C., Kroon, H. M., Taminiau, A. H., & Bloem, J. L. (2003). Magnetic resonance imaging in the assessment of bone tumors. Skeletal Radiology, 32(1), 1-17.
Considering the challenges of long scan times, are there specific strategies for improving patient comfort and compliance during 4D flow MRI, especially when imaging areas prone to motion artifacts, such as the abdomen or during musculoskeletal assessments?
That’s a great point! Patient comfort is key. Beyond accelerated sequences, techniques like providing clear instructions, using comfortable positioning aids, and even incorporating short breaks can really help. For abdominal imaging, respiratory training before the scan can also improve compliance and reduce motion artifacts. Has anyone had success with other strategies?
Editor: MedTechNews.Uk
Thank you to our Sponsor Esdebe
The discussion of AI and ML integration is particularly exciting. How might these technologies be used to optimize pulse sequence parameters in real-time based on patient-specific factors, further reducing scan times and improving image quality in 4D flow MRI?
That’s a fantastic question! The potential for AI/ML to personalize pulse sequences in real-time is truly game-changing. Imagine AI analyzing initial scan data to adjust parameters on-the-fly, optimizing for each patient’s unique physiology. This could lead to faster, more accurate 4D flow MRI, ultimately improving patient care.
Editor: MedTechNews.Uk
Thank you to our Sponsor Esdebe
The report mentions challenges with long scan times. Could advancements in parallel imaging and compressed sensing, alongside AI-driven reconstruction, further reduce acquisition times for non-cardiovascular applications, and how might this impact clinical adoption?