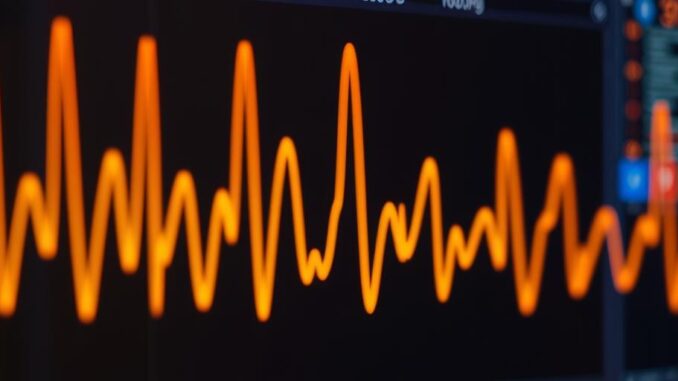
Abstract
Bioimpedance spectroscopy (BIS) is a non-invasive technique that utilizes the electrical properties of biological tissues to assess physiological parameters. This report provides a comprehensive overview of BIS, starting with its fundamental principles based on tissue conductivity and permittivity at different frequencies. It then delves into a wide array of applications, extending beyond body composition analysis and milk flow measurement, including fluid status monitoring, disease detection, and drug delivery evaluation. The report also critically examines the limitations of BIS, such as susceptibility to movement artifacts and the dependence on accurate electrode placement. Furthermore, it explores advanced technologies and algorithms employed for data interpretation, focusing on equivalent circuit modeling and machine learning approaches. Finally, the report addresses the crucial aspects of safety, focusing on minimizing current density and ensuring compliance with regulatory standards. We also consider the ethical implications of using BIS, and present a perspective on the future of BIS as a powerful tool for personalized medicine and proactive healthcare management.
Many thanks to our sponsor Esdebe who helped us prepare this research report.
1. Introduction
Bioimpedance, the opposition to the flow of alternating electrical current through biological tissues, has emerged as a valuable tool for non-invasive physiological monitoring. This phenomenon, exploited in bioimpedance spectroscopy (BIS), provides insights into tissue composition, fluid distribution, and cellular health by analyzing the complex impedance spectrum over a range of frequencies. While the initial applications of bioimpedance were primarily focused on body composition analysis, its potential has expanded significantly in recent years, encompassing a diverse range of medical diagnostics and therapeutic interventions. The core principle lies in the fact that different biological tissues exhibit varying electrical properties due to their composition and structure. For instance, intracellular and extracellular fluids, cell membranes, and connective tissues all contribute uniquely to the overall bioimpedance signal. By carefully analyzing these variations, researchers and clinicians can extract valuable information about the underlying physiological state.
This report aims to provide a comprehensive overview of BIS, covering its fundamental principles, diverse applications, limitations, and future directions. Furthermore, we discuss the safety considerations associated with the use of electrical currents in medical applications, with particular emphasis on the strategies to minimize potential risks. The information provided is aimed at experts in the field, to provide new perspective, discussion and challenges for the technique.
Many thanks to our sponsor Esdebe who helped us prepare this research report.
2. Fundamental Principles of Bioimpedance
2.1 Impedance, Reactance, and Phase Angle
Bioimpedance (Z) is a complex quantity composed of resistance (R) and reactance (X). Resistance represents the opposition to current flow due to the conductive properties of the tissue, primarily influenced by the electrolytes present in intra- and extracellular fluids. Reactance, on the other hand, reflects the capacitive effects arising from cell membranes and other tissue interfaces that store electrical energy. Impedance is formally defined as:
Z = R + jX
where j is the imaginary unit.
Reactance is further divided into capacitive reactance (Xc) and inductive reactance (Xl). In biological tissues, capacitive reactance predominates, arising from the cell membranes acting as capacitors. The capacitive reactance is inversely proportional to the frequency (f) of the applied current, according to the formula:
Xc = -1 / (2πfC)
where C is the capacitance.
The phase angle (θ) between the applied voltage and the resulting current provides valuable information about the relative contributions of resistance and reactance to the overall impedance. It is calculated as:
θ = arctan(X/R)
In biological tissues, the phase angle typically falls between 0 and -90 degrees, with a value closer to 0 indicating a predominantly resistive behavior and a value closer to -90 indicating a predominantly capacitive behavior. Changes in phase angle can therefore indicate changes in tissue composition.
2.2 Frequency Dependence of Bioimpedance
Bioimpedance measurements are typically performed over a wide range of frequencies, from a few Hertz to several Megahertz. This frequency dependence is crucial for distinguishing between intra- and extracellular compartments. At low frequencies, the applied current primarily flows through the extracellular space because the cell membranes act as insulators, effectively blocking the current from entering the cells. However, as the frequency increases, the capacitive reactance of the cell membranes decreases, allowing the current to penetrate the intracellular space. This phenomenon allows researchers to separate intra- and extracellular impedance contributions. The frequency at which the current begins to penetrate cell membranes, called the characteristic frequency, is tissue-dependent and provides information about cell size and membrane properties.
2.3 Cole Model
The Cole model is a widely used empirical model to describe the frequency dependence of bioimpedance in biological tissues. It is expressed as:
Z(ω) = R∞ + (R0 – R∞) / (1 + (jωτ)^α)
where:
* Z(ω) is the impedance at angular frequency ω.
* R0 is the impedance at zero frequency.
* R∞ is the impedance at infinite frequency.
* τ is the relaxation time constant.
* α is a dispersion parameter (0 < α ≤ 1).
The Cole model provides a mathematical framework for characterizing the bioimpedance spectrum and extracting parameters that are related to tissue properties, such as cellularity and membrane capacitance. While it offers a simplified representation of complex biological tissues, it has proven to be a valuable tool for analyzing and interpreting BIS data.
Many thanks to our sponsor Esdebe who helped us prepare this research report.
3. Applications of Bioimpedance Spectroscopy
3.1 Body Composition Analysis
One of the most established applications of BIS is body composition analysis, specifically the determination of fat-free mass (FFM) and body fat percentage (BF%). BIS estimates FFM based on the principle that FFM is primarily composed of water and electrolytes, while fat tissue is relatively non-conductive. However, standard body composition analysis via BIS, typically uses a single frequency, often 50kHz. In comparison, BIS can more accurately estimate body composition across populations with varying levels of hydration, such as elite athletes, clinical patients and the elderly, than can single-frequency bioelectrical impedance analysis (BIA).
3.2 Fluid Status Monitoring
BIS is highly sensitive to changes in fluid volume and distribution, making it a valuable tool for monitoring fluid status in various clinical settings. An increase in extracellular fluid volume, for example, leads to a decrease in impedance, while dehydration results in an increase in impedance. BIS can be used to assess fluid overload in patients with heart failure or kidney disease, guide fluid resuscitation in critically ill patients, and monitor fluid shifts during dialysis. The ability to differentiate between intra- and extracellular fluid compartments further enhances the diagnostic capabilities of BIS in fluid management.
3.3 Cancer Detection and Monitoring
Malignant tissues often exhibit altered electrical properties compared to normal tissues due to changes in cellular structure, membrane properties, and vascularity. BIS can be used to detect these subtle changes and differentiate between cancerous and healthy tissues. Several studies have shown the potential of BIS for detecting breast cancer, skin cancer, and other types of tumors. BIS can also be used to monitor tumor response to therapy by tracking changes in impedance over time.
The use of BIS to identify cancerous tissue has been demonstrated in both in-vitro and in-vivo settings. For example, BIS has been used to differentiate between cancerous and normal breast tissues based on their impedance characteristics. Additionally, BIS has been used to monitor the effectiveness of chemotherapy treatment in cancer patients by tracking changes in tumor impedance over time. In essence, BIS offers a non-invasive and potentially more cost-effective means of cancer detection and monitoring, complementing traditional methods like biopsies and imaging techniques.
3.4 Lung Function Monitoring
BIS can be used to assess lung function by measuring changes in thoracic impedance during respiration. During inhalation, the air-filled lungs cause an increase in thoracic impedance, while exhalation leads to a decrease in impedance. BIS can provide information about tidal volume, respiratory rate, and other respiratory parameters. It has potential applications in monitoring patients with chronic obstructive pulmonary disease (COPD), asthma, and other respiratory disorders.
3.5 Assessment of Wound Healing
The healing process of wounds involves complex physiological changes, including inflammation, angiogenesis, and tissue remodeling. BIS can be used to monitor these changes by measuring the impedance of the tissue surrounding the wound. As the wound heals, the impedance typically increases due to the formation of new tissue and the decrease in inflammation. BIS can provide valuable information about the progress of wound healing and guide treatment strategies.
3.6 Drug Delivery Evaluation
BIS can be used to evaluate the effectiveness of drug delivery systems by monitoring the changes in tissue impedance following drug administration. For example, BIS can be used to track the penetration of drugs into tissues, assess the release of drugs from implanted devices, and monitor the local tissue response to drug delivery. This application is particularly relevant for targeted drug delivery strategies, where the goal is to deliver drugs selectively to specific tissues or cells.
3.7 Non-invasive Glucose Monitoring
While not yet widely adopted, there is ongoing research into using BIS for non-invasive glucose monitoring in diabetic patients. The principle relies on the fact that changes in blood glucose levels can affect the electrical conductivity of the blood and surrounding tissues. However, this application faces significant challenges due to the small impedance changes associated with glucose variations and the influence of other physiological factors. Advances in sensor technology and data analysis algorithms are needed to improve the accuracy and reliability of BIS-based glucose monitoring.
Many thanks to our sponsor Esdebe who helped us prepare this research report.
4. Limitations of Bioimpedance Spectroscopy
4.1 Sensitivity to Movement Artifacts
BIS measurements are highly sensitive to movement artifacts, which can introduce significant errors in the impedance data. Even small movements of the body or limbs can alter the electrode-tissue contact and change the measured impedance. This limitation is particularly relevant in ambulatory monitoring applications, where the subject is free to move around.
4.2 Dependence on Electrode Placement
The accuracy of BIS measurements is highly dependent on the accurate and consistent placement of electrodes. Small variations in electrode position can significantly affect the measured impedance, especially at higher frequencies. Therefore, standardized electrode placement protocols are essential to ensure the reproducibility and comparability of BIS data. This is more problematic in home use scenarios where it is harder to enforce these protocols.
4.3 Influence of Hydration Status
Hydration status has a significant impact on bioimpedance measurements, as water is the primary conductor of electrical current in the body. Dehydration leads to an increase in impedance, while overhydration leads to a decrease in impedance. Therefore, it is essential to control for hydration status when interpreting BIS data, particularly in studies involving body composition analysis or fluid status monitoring.
4.4 Skin Impedance
Skin impedance can significantly influence BIS measurements, particularly at low frequencies. The stratum corneum, the outermost layer of the skin, acts as a significant barrier to electrical current flow. The impedance of the stratum corneum can vary depending on skin hydration, temperature, and other factors. Therefore, it is important to minimize skin impedance by using proper skin preparation techniques, such as gentle abrasion or the application of conductive gel. This also adds to the preparation time when undertaking BIS measurements and can be problematic in some clinical settings.
4.5 Individual Variability
Biological tissues exhibit inherent variability in their electrical properties due to differences in body size, composition, and physiological state. This individual variability can make it challenging to establish universal reference values for BIS measurements. Therefore, it is important to consider individual factors when interpreting BIS data and to establish subject-specific baseline values whenever possible.
4.6 Ethical considerations
Although considered a safe and non-invasive technique, ethical considerations still need to be taken into account with BIS. Current leakage, or other electrical faults, while rare, can cause burns or even cardiac arrest. Therefore, proper design and safety checks are essential to minimize this risk. Moreover, the data generated by BIS can be sensitive and should be handled with care to protect patient privacy. Finally, it is important to obtain informed consent from patients before performing BIS measurements and to explain the potential risks and benefits of the procedure.
Many thanks to our sponsor Esdebe who helped us prepare this research report.
5. Technologies and Algorithms for Data Interpretation
5.1 Equivalent Circuit Modeling
Equivalent circuit modeling is a common approach for interpreting BIS data. This involves representing the biological tissue as an electrical circuit consisting of resistors, capacitors, and inductors. The values of these circuit elements are then estimated by fitting the model to the measured impedance data. The circuit elements are typically associated with specific tissue components, such as intracellular fluid, extracellular fluid, and cell membranes. Equivalent circuit modeling provides insights into the underlying physiological properties of the tissue.
A commonly used equivalent circuit is the Cole model, as discussed earlier. More complex models can be created to account for additional tissue components or effects, such as electrode polarization. However, more complex models require more parameters to be estimated, which can lead to overfitting and reduced accuracy. Finding the right balance between model complexity and accuracy is crucial for successful equivalent circuit modeling.
5.2 Machine Learning Approaches
Machine learning algorithms, such as support vector machines, neural networks, and random forests, are increasingly being used to analyze BIS data. These algorithms can learn complex patterns and relationships in the impedance data and can be used for classification, regression, and prediction tasks. For example, machine learning can be used to differentiate between cancerous and healthy tissues, predict fluid overload, or estimate body composition. The advantage of machine learning is that it does not require a priori assumptions about the underlying tissue properties. However, machine learning algorithms require large datasets for training and validation to avoid overfitting and ensure generalizability.
5.3 Finite Element Analysis
Finite element analysis (FEA) is a computational technique used to simulate the distribution of electrical current in biological tissues. FEA models can incorporate detailed anatomical information and tissue-specific electrical properties. By simulating the current distribution, FEA can provide insights into the sensitivity of BIS measurements to different tissue compartments and electrode configurations. FEA can also be used to optimize electrode placement and to correct for geometric effects.
5.4 Multi-Frequency Bioimpedance Analysis (MFBIA)
MFBIA involves measuring bioimpedance at multiple frequencies and analyzing the resulting impedance spectrum to obtain information about tissue composition and fluid distribution. This approach can provide more detailed information than single-frequency BIA. Different frequencies penetrate tissues to varying degrees, allowing for the characterization of both intracellular and extracellular compartments. However, MFBIA requires more sophisticated instrumentation and data analysis techniques.
Many thanks to our sponsor Esdebe who helped us prepare this research report.
6. Safety Aspects of Bioimpedance
6.1 Current Density Limits
The primary safety concern with bioimpedance measurements is the potential for electrical stimulation and tissue damage due to the applied current. To minimize this risk, it is essential to adhere to strict current density limits. Regulatory standards, such as those established by the International Electrotechnical Commission (IEC), specify maximum allowable current densities for medical devices. The current density should be kept well below the threshold for nerve stimulation or tissue heating.
6.2 Electrode Material and Design
The choice of electrode material and design is crucial for minimizing skin irritation and preventing electrode polarization. Electrodes should be made of biocompatible materials, such as silver-silver chloride (Ag/AgCl), and should have a large surface area to minimize current density. Electrode gel should be used to improve electrode-skin contact and reduce skin impedance. The design of the electrode should ensure uniform current distribution and prevent current concentration at specific points.
6.3 Isolation and Leakage Current
Medical devices that apply electrical currents to the body must be properly isolated from the mains power supply to prevent electrical shock hazards. Isolation transformers and other safety features are used to ensure that the patient is protected from high-voltage currents. Leakage current, the current that flows through the insulation to ground, should be kept below specified limits to prevent electrical shock hazards. Regular testing of leakage current is essential to ensure the safety of medical devices.
6.4 Considerations for Specific Patient Populations
Specific patient populations, such as pregnant women and individuals with implanted electronic devices (e.g., pacemakers), may require special considerations when performing bioimpedance measurements. In pregnant women, the electrical conductivity of the amniotic fluid can affect the impedance measurements. In individuals with pacemakers, the applied current could potentially interfere with the device function. Therefore, it is important to consult with a physician before performing bioimpedance measurements on these populations.
Many thanks to our sponsor Esdebe who helped us prepare this research report.
7. Future Directions and Conclusions
Bioimpedance spectroscopy is a rapidly evolving field with tremendous potential for advancing medical diagnostics and personalized healthcare. Several key areas of research and development are expected to drive future progress:
- Miniaturization and Wearable Sensors: The development of miniaturized and wearable BIS sensors will enable continuous, real-time monitoring of physiological parameters in ambulatory settings. These sensors could be integrated into smartwatches, patches, or clothing to provide continuous insights into fluid status, body composition, and other health indicators.
- Advanced Data Analysis Algorithms: The application of advanced data analysis algorithms, such as machine learning and deep learning, will enhance the accuracy and reliability of BIS measurements. These algorithms can learn complex patterns and relationships in the impedance data and can be used for disease detection, prediction, and personalized therapy.
- Integration with Other Sensing Modalities: Integrating BIS with other sensing modalities, such as electrocardiography (ECG), photoplethysmography (PPG), and accelerometry, will provide a more comprehensive assessment of physiological state. This multi-modal approach can improve the accuracy and robustness of diagnostic and monitoring applications.
- Point-of-Care Diagnostics: The development of point-of-care BIS devices will enable rapid and convenient testing in various clinical settings, such as emergency rooms, clinics, and home healthcare. These devices could be used for triage, diagnosis, and monitoring of various medical conditions.
- Personalized Medicine: BIS can be used to personalize medical treatment by tailoring therapy to individual patient characteristics. For example, BIS can be used to guide fluid management in patients with heart failure or kidney disease, or to monitor tumor response to therapy in cancer patients.
In conclusion, Bioimpedance Spectroscopy (BIS) is a promising non-invasive technique with a wide range of applications in physiological monitoring. Its ability to provide valuable information about tissue composition, fluid distribution, and cellular health makes it a powerful tool for medical diagnostics and personalized healthcare. While BIS has limitations, such as sensitivity to movement artifacts and dependence on electrode placement, ongoing research and technological advances are addressing these challenges and expanding the capabilities of the technology. The future of BIS is bright, with the potential to revolutionize healthcare by enabling continuous, real-time monitoring of physiological parameters and personalized medical treatment.
Many thanks to our sponsor Esdebe who helped us prepare this research report.
References
- Grimnes, S., & Martinsen, Ø. G. (2015). Bioimpedance and Bioelectricity Basics (3rd ed.). Academic Press.
- Kyle, U. G., Bosaeus, I., De Lorenzo, A. D., Deurenberg, P., Elia, M., Gómez, J. M., … & Pichard, C. (2004). Bioelectrical impedance analysis—part I: review of principles and methods. Clinical Nutrition, 23(5), 1226-1243.
- Lukaski, H. C., Johnson, P. E., Bolonchuk, W. W., & Lykken, G. I. (1985). Assessment of fat-free mass using bioelectrical impedance measurements of the human body. The American Journal of Clinical Nutrition, 41(4), 810-817.
- Gabriel, S., Lau, R. W., & Gabriel, C. (1996). The dielectric properties of biological tissues: III. Parametric models for the dielectric spectrum. Physics in Medicine & Biology, 41(11), 2271.
- Jaffrin, M. Y., & Morel, H. (2008). Body fluid volumes measurement by impedance: A review. Medical Engineering & Physics, 30(10), 1257-1269.
- Chung, S., Hong, S. H., & Kim, S. H. (2017). Cancer detection using bioelectrical impedance spectroscopy: A review. Journal of Electrical Engineering & Technology, 12(1), 1-13.
- Cornish, B. H., Chapman, M., Hirst, N. G., & Ward, L. C. (2008). Early diagnosis of secondary lymphedema after breast cancer treatment using multiple frequency bioimpedance. Breast Cancer Research and Treatment, 110(3), 421-427.
- Holder, D. S. (2005). Electrical impedance tomography: methods, history, and applications. CRC press.
- IEC 60601-1: Medical electrical equipment – Part 1: General requirements for basic safety and essential performance. International Electrotechnical Commission.
Given the dependence of BIS accuracy on consistent electrode placement, how feasible is the development of automated or guided electrode placement systems to mitigate user error, particularly in home-use scenarios, and what technological hurdles remain?
That’s a great point! Automated electrode placement is definitely a key area for improving BIS, especially for home use. Miniaturization of sensors and integration with AI for real-time feedback are exciting possibilities, but ensuring patient comfort and affordability will be crucial technological challenges.
Editor: MedTechNews.Uk
Thank you to our Sponsor Esdebe
Given the sensitivity of BIS to hydration status, could longitudinal studies incorporating BIS measurements offer insights into the efficacy of different rehydration strategies for athletes or individuals with chronic conditions affecting fluid balance?
That’s an excellent question! Absolutely, longitudinal studies using BIS to monitor hydration could be incredibly valuable. Imagine tracking athletes’ fluid balance throughout training, or customizing rehydration plans for individuals with conditions like kidney disease based on real-time BIS data. It could really refine personalized hydration strategies!
Editor: MedTechNews.Uk
Thank you to our Sponsor Esdebe
The discussion of ethical considerations is vital. As BIS technology advances, how can we ensure data privacy and prevent potential misuse of physiological data collected through wearable or point-of-care devices, especially with the increasing integration of AI in data interpretation?
That’s a fantastic point! With more data being collected via wearable BIS devices, securing data privacy is paramount. Perhaps anonymization techniques coupled with blockchain for enhanced security could be a viable solution? Further discussion on robust regulatory frameworks to prevent misuse is definitely needed as AI gets more involved.
Editor: MedTechNews.Uk
Thank you to our Sponsor Esdebe
Ethical considerations are key! Could BIS data, combined with AI, predict health risks before they manifest? Imagine the proactive possibilities, but also the responsibility to avoid biases and ensure equitable access. Food for thought!