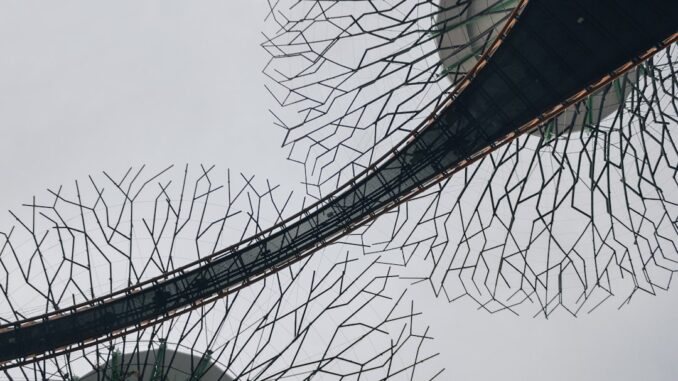
Abstract
Biomimicry, the practice of emulating nature’s strategies and designs to solve human problems, holds immense potential for revolutionizing healthcare. This research report provides a comprehensive exploration of biomimicry’s applications within the medical field, moving beyond simple mimicry to explore the underlying biological principles that can inform innovative medical technologies and therapies. We examine diverse examples, spanning from bio-inspired materials and drug delivery systems to diagnostic tools and regenerative medicine approaches. The report delves into the advantages of biomimicry, including enhanced biocompatibility, improved efficiency, and sustainable design. Furthermore, we critically assess the challenges and opportunities associated with integrating biological principles into medical technology, including regulatory hurdles, ethical considerations, and the need for interdisciplinary collaboration. The report concludes by outlining future research directions and highlighting the transformative potential of biomimicry in shaping the future of healthcare.
Many thanks to our sponsor Esdebe who helped us prepare this research report.
1. Introduction: The Power of Nature’s Design
For millennia, humans have looked to nature for inspiration. Biomimicry, a formalization of this ancient practice, involves consciously emulating nature’s forms, processes, and systems to create sustainable and effective solutions to human challenges. Unlike simply copying natural appearances (biomorphism), biomimicry seeks to understand and replicate the underlying functional principles that drive natural systems. In recent decades, biomimicry has emerged as a powerful paradigm across diverse fields, including engineering, architecture, and materials science. Within healthcare, the application of biomimicry is rapidly gaining traction, offering the potential to develop novel medical devices, therapies, and diagnostic tools that are both innovative and inherently compatible with the human body.
Traditional medical innovation often relies on synthetic materials and reductionist approaches, which can sometimes lead to biocompatibility issues, limited efficacy, and unintended side effects. Biomimicry offers an alternative pathway, leveraging the inherent elegance and efficiency of biological systems that have been refined through millions of years of evolution. By studying and mimicking these systems, researchers can develop solutions that are inherently more biocompatible, adaptable, and sustainable. For example, adhesives inspired by gecko feet are being developed for wound closure, and drug delivery systems based on viral capsids are showing promise for targeted therapy.
This report aims to provide a comprehensive overview of biomimicry in healthcare, exploring the breadth of its applications, analyzing its advantages and limitations, and outlining future research directions. We will move beyond superficial mimicry to delve into the underlying biological principles that drive successful biomimetic designs. This includes a detailed examination of relevant literature, case studies of successful biomimetic medical technologies, and a discussion of the challenges and opportunities associated with integrating biological principles into medical innovation.
Many thanks to our sponsor Esdebe who helped us prepare this research report.
2. Biomimicry in Materials Science and Engineering
Materials science and engineering represent a cornerstone of medical innovation, and biomimicry offers a rich source of inspiration for developing advanced biomaterials with enhanced properties. This section explores several key areas where biomimicry is driving innovation in medical materials.
2.1. Bio-inspired Adhesives:
Nature’s adhesives, such as those found in gecko feet, mussels, and barnacles, exhibit remarkable adhesive strength, biocompatibility, and water resistance. Gecko feet, for example, rely on van der Waals forces generated by millions of microscopic setae, allowing them to adhere to almost any surface. Inspired by this mechanism, researchers have developed synthetic adhesives with hierarchical structures that mimic the setae, enabling strong and reversible adhesion in both dry and wet environments. These adhesives hold immense potential for wound closure, surgical sealants, and drug delivery systems. Mussel-inspired adhesives, on the other hand, utilize the amino acid 3,4-dihydroxyphenylalanine (DOPA) to form strong bonds with a variety of surfaces, including metals, ceramics, and polymers. This property has been exploited to create biocompatible adhesives for tissue engineering and implant coatings. Furthermore, barnacle cement, known for its exceptional underwater adhesion, is being studied to develop robust and durable adhesives for dental implants and bone regeneration.
2.2. Bio-inspired Scaffolds for Tissue Engineering:
Tissue engineering aims to regenerate damaged or diseased tissues by providing a scaffold that supports cell growth and differentiation. Natural extracellular matrices (ECMs) provide an ideal template for tissue regeneration due to their complex architecture, biocompatibility, and ability to interact with cells. Researchers are developing bio-inspired scaffolds that mimic the structure and composition of native ECMs using a variety of materials, including collagen, hyaluronic acid, and chitosan. These scaffolds can be designed with specific pore sizes, mechanical properties, and surface chemistries to promote cell adhesion, proliferation, and differentiation. For example, scaffolds inspired by the architecture of bone trabeculae are being developed to regenerate bone tissue, while scaffolds mimicking the structure of blood vessels are being used to create vascular grafts.
2.3. Bio-inspired Coatings for Implants:
The biocompatibility of medical implants is crucial for their long-term success. Bio-inspired coatings can improve implant biocompatibility by mimicking the surface properties of natural tissues. For example, coatings based on the bone mineral hydroxyapatite (HA) are widely used to enhance osseointegration (bone growth onto the implant surface). These coatings can be further modified with growth factors and other bioactive molecules to promote bone formation and reduce the risk of implant failure. Furthermore, coatings inspired by the slippery surface of pitcher plants are being developed to prevent bacterial adhesion and biofilm formation on implants, reducing the risk of infection.
Many thanks to our sponsor Esdebe who helped us prepare this research report.
3. Biomimicry in Drug Delivery Systems
Targeted drug delivery is a crucial aspect of modern medicine, aiming to minimize side effects and maximize therapeutic efficacy. Biomimicry provides a powerful toolkit for developing innovative drug delivery systems that can selectively target diseased tissues and cells.
3.1. Virus-inspired Nanoparticles:
Viruses have evolved sophisticated mechanisms for entering cells and delivering their genetic material. Researchers are leveraging these mechanisms to develop virus-inspired nanoparticles for targeted drug delivery. These nanoparticles, often based on viral capsids or viral-like particles (VLPs), can be engineered to selectively bind to specific cell types and deliver therapeutic payloads, such as drugs, genes, or proteins. By mimicking the size, shape, and surface properties of viruses, these nanoparticles can effectively evade the immune system and penetrate biological barriers. For example, adeno-associated virus (AAV) vectors, which are derived from a non-pathogenic virus, are widely used in gene therapy due to their ability to efficiently transduce a variety of cell types.
3.2. Cell-inspired Vesicles:
Cells naturally secrete vesicles, such as exosomes and microvesicles, which play a crucial role in intercellular communication. These vesicles contain a variety of bioactive molecules, including proteins, RNA, and lipids, and can be used to deliver therapeutic agents to specific target cells. Researchers are developing cell-inspired vesicles for drug delivery by encapsulating drugs or other therapeutic molecules within these vesicles. These vesicles can be engineered to target specific cell types by modifying their surface proteins or lipids. For example, exosomes derived from cancer cells can be used to deliver anti-cancer drugs specifically to tumor cells, minimizing the toxicity to healthy tissues.
3.3. Bacteria-inspired Drug Delivery:
Certain bacteria exhibit a natural tropism for tumor tissues, making them attractive candidates for targeted drug delivery. Researchers are engineering bacteria to deliver anti-cancer drugs directly to tumor cells. These bacteria can be genetically modified to produce therapeutic proteins or to carry drug-loaded nanoparticles. Furthermore, bacteria can be engineered to respond to specific stimuli within the tumor microenvironment, such as hypoxia or acidity, triggering the release of the therapeutic payload. This approach offers the potential for highly targeted and controlled drug delivery to tumors, minimizing systemic toxicity.
Many thanks to our sponsor Esdebe who helped us prepare this research report.
4. Biomimicry in Diagnostics and Imaging
Early and accurate diagnosis is critical for effective disease management. Biomimicry is inspiring the development of novel diagnostic tools and imaging techniques with improved sensitivity, specificity, and ease of use.
4.1. Bio-inspired Sensors:
Nature provides a wealth of inspiration for developing highly sensitive and specific sensors. For example, the olfactory system of insects can detect minute amounts of chemicals in the air. Researchers are developing bio-inspired sensors that mimic the structure and function of insect olfactory receptors to detect biomarkers of disease in breath, urine, or blood. These sensors can be used to diagnose a variety of diseases, including cancer, diabetes, and infectious diseases. Furthermore, sensors inspired by the electroreceptors of electric fish are being developed to detect subtle changes in electrical fields, which can be indicative of disease.
4.2. Bio-inspired Contrast Agents for Imaging:
Contrast agents are used to enhance the visibility of tissues and organs during medical imaging. Researchers are developing bio-inspired contrast agents that mimic the properties of natural molecules, such as melanin and hemoglobin. These contrast agents offer improved biocompatibility, biodegradability, and targeting capabilities compared to traditional contrast agents. For example, melanin-based nanoparticles are being developed for photoacoustic imaging of tumors, while hemoglobin-based contrast agents are being used for magnetic resonance imaging (MRI) of blood vessels.
4.3. Bio-inspired Microfluidic Devices:
Microfluidic devices, which manipulate fluids at the microscale, are increasingly used for diagnostic applications. Researchers are developing bio-inspired microfluidic devices that mimic the structure and function of natural microvascular networks. These devices can be used to isolate and analyze cells, proteins, and nucleic acids from biological samples. For example, microfluidic devices inspired by the structure of the kidney glomerulus are being developed to filter blood and detect biomarkers of kidney disease.
Many thanks to our sponsor Esdebe who helped us prepare this research report.
5. Biomimicry in Regenerative Medicine
Regenerative medicine aims to repair or replace damaged tissues and organs. Biomimicry offers a powerful approach for developing strategies that promote tissue regeneration and functional recovery.
5.1. Bio-inspired Strategies for Stem Cell Differentiation:
Stem cells have the potential to differentiate into a variety of cell types, making them a promising source for regenerative medicine. Researchers are developing bio-inspired strategies to control stem cell differentiation by mimicking the natural microenvironment of cells. This includes providing stem cells with the appropriate biochemical signals, mechanical cues, and extracellular matrix components. For example, scaffolds inspired by the structure of bone are being used to promote the differentiation of stem cells into bone cells, while scaffolds mimicking the structure of cartilage are being used to promote the differentiation of stem cells into cartilage cells.
5.2. Bio-inspired Approaches for Wound Healing:
Wound healing is a complex process that involves a coordinated series of events, including inflammation, cell migration, and matrix deposition. Researchers are developing bio-inspired approaches to accelerate wound healing by mimicking the natural processes that occur during tissue repair. This includes developing wound dressings that release growth factors, stimulate angiogenesis (blood vessel formation), and promote cell migration. For example, wound dressings inspired by the structure of spider silk are being developed to provide a protective barrier against infection and promote tissue regeneration.
5.3. Bio-inspired Strategies for Organ Regeneration:
Organ regeneration is a major goal of regenerative medicine. Researchers are developing bio-inspired strategies to regenerate damaged or diseased organs by providing a scaffold that supports cell growth and differentiation. This includes using decellularized organs, which are organs that have been stripped of their cells, leaving behind the extracellular matrix. The decellularized organ can then be seeded with cells from the patient, creating a bio-artificial organ that is less likely to be rejected by the immune system. Furthermore, researchers are developing 3D-printed organs that mimic the structure and function of native organs. These organs can be customized to the patient’s specific needs, offering the potential to replace damaged or diseased organs with functional replacements.
Many thanks to our sponsor Esdebe who helped us prepare this research report.
6. Advantages of Biomimicry in Healthcare
The adoption of biomimicry in healthcare offers several compelling advantages over traditional approaches:
- Enhanced Biocompatibility: Biomimetic materials and devices are often inherently more biocompatible because they are based on biological principles and materials that the body recognizes and tolerates. This reduces the risk of adverse immune responses and improves the long-term performance of medical implants and devices.
- Improved Efficiency: Natural systems have evolved to be incredibly efficient, optimizing resource use and minimizing waste. By mimicking these systems, researchers can develop medical technologies that are more efficient in terms of energy consumption, resource utilization, and therapeutic efficacy.
- Sustainable Design: Biomimicry promotes sustainable design principles by encouraging the use of renewable resources, minimizing waste, and creating closed-loop systems. This is particularly important in healthcare, where the environmental impact of medical waste and resource consumption can be significant.
- Novel Functionality: Nature has evolved a vast array of solutions to complex problems. Biomimicry can unlock novel functionalities that are not readily achievable through traditional engineering approaches. This can lead to the development of entirely new medical devices and therapies.
- Reduced Development Time and Cost: While initial research may be intensive, leveraging already-existing, proven biological mechanisms can, in some cases, reduce the overall development time and cost associated with bringing new medical technologies to market. This is because the fundamental design principles have already been validated by nature.
Many thanks to our sponsor Esdebe who helped us prepare this research report.
7. Challenges and Opportunities
Despite its immense potential, biomimicry faces several challenges in its integration into mainstream healthcare:
- Complexity of Biological Systems: Nature is incredibly complex, and fully understanding and replicating biological systems can be challenging. This requires interdisciplinary collaboration between biologists, engineers, and clinicians.
- Regulatory Hurdles: Biomimetic medical devices and therapies may face regulatory hurdles due to their novelty and the lack of established regulatory pathways. Clear and predictable regulatory guidelines are needed to facilitate the development and commercialization of biomimetic technologies.
- Ethical Considerations: The use of biological materials and the manipulation of biological systems raise ethical considerations that need to be addressed. This includes issues related to informed consent, patient safety, and the potential for unintended consequences.
- Scalability and Manufacturing: Scaling up the production of biomimetic materials and devices can be challenging. Cost-effective and scalable manufacturing processes are needed to make these technologies widely accessible.
- Public Perception and Acceptance: Public perception and acceptance of biomimetic medical technologies can be influenced by concerns about safety, efficacy, and ethical implications. Effective communication and education are needed to build public trust and promote the adoption of these technologies.
However, these challenges also present significant opportunities:
- Interdisciplinary Collaboration: The need to understand and replicate biological systems fosters interdisciplinary collaboration between biologists, engineers, clinicians, and other experts. This collaboration can lead to new insights and innovations that would not be possible through traditional disciplinary approaches.
- Development of New Regulatory Frameworks: The emergence of biomimetic medical technologies is driving the development of new regulatory frameworks that are tailored to the unique characteristics of these technologies. This will facilitate the development and commercialization of biomimetic technologies while ensuring patient safety.
- Increased Public Awareness: The growing interest in biomimicry is raising public awareness of the importance of nature-inspired innovation. This can lead to increased funding for research and development in this area.
- Sustainable Healthcare Solutions: Biomimicry offers the potential to develop sustainable healthcare solutions that are both effective and environmentally friendly. This is particularly important in the face of growing healthcare costs and environmental challenges.
Many thanks to our sponsor Esdebe who helped us prepare this research report.
8. Future Directions
The future of biomimicry in healthcare is bright, with numerous avenues for further research and development:
- Advanced Materials: Developing new bio-inspired materials with improved mechanical properties, biocompatibility, and functionality.
- Personalized Medicine: Tailoring biomimetic medical devices and therapies to the specific needs of individual patients based on their genetic profile, medical history, and lifestyle.
- Artificial Intelligence: Using artificial intelligence to analyze biological data and identify new opportunities for biomimicry in healthcare.
- Integration of Multiple Biomimetic Principles: Combining multiple biomimetic principles to create more complex and sophisticated medical technologies.
- Education and Training: Developing educational programs and training opportunities to equip the next generation of scientists and engineers with the skills needed to advance biomimicry in healthcare.
Many thanks to our sponsor Esdebe who helped us prepare this research report.
9. Conclusion
Biomimicry offers a transformative approach to medical innovation, providing a pathway to develop more biocompatible, efficient, and sustainable healthcare solutions. By studying and emulating nature’s designs, researchers can unlock novel functionalities and address unmet medical needs. While challenges remain in integrating biological principles into medical technology, the potential benefits are immense. As we deepen our understanding of natural systems and refine our ability to replicate them, biomimicry will play an increasingly important role in shaping the future of healthcare, leading to innovative therapies, diagnostic tools, and regenerative medicine strategies that improve human health and well-being.
Many thanks to our sponsor Esdebe who helped us prepare this research report.
References
- Bhushan, B. (2009). Biomimetics: lessons from nature–an overview. Philosophical Transactions of the Royal Society A: Mathematical, Physical and Engineering Sciences, 367(1893), 1445-1486.
- Fink, J., & van de Ven, A. (2020). Design lessons from nature: biomimicry, innovation and the evolution of design thinking. Journal of Engineering Design, 31(12), 327-347.
- Hsu, C. H., Ali, M. A., Chen, Y. L., Chen, T. J., & Lin, C. H. (2022). Biomimicry in tissue engineering: Trends, challenges, and future directions. Journal of Biomedical Materials Research Part A, 110(3), 749-771.
- Meyers, M. A., Chen, P. Y., Lin, A. Y. M., & Seki, Y. (2008). Biological materials: structure and mechanical properties. Progress in Materials Science, 53(1), 1-206.
- Rao, S., Desai, T. A., & Bhatia, S. N. (2010). Micro- and nanoscale devices in tissue engineering and regenerative medicine. Advanced Drug Delivery Reviews, 62(3), 339-355.
- Vogel, S. (2013). Cats’ paws and catapults: Mechanical worlds of nature and people. WW Norton & Company.
- Vincent, J. F. V., Bogatyreva, O., Pahl, A. K., & Curry, M. W. (2006). Biomimetics: its practice and theory. Journal of the Royal Society Interface, 3(9), 471-482.
- Wolf, F., Helfrich, A., Salber, J., Dringen, R., Reske, D., & Bechmann, I. (2014). Cell-based delivery of nanoparticles to the brain. Advanced Drug Delivery Reviews, 71, 77-100.
The discussion around bio-inspired coatings for implants raises an interesting point. Could we see coatings that not only improve biocompatibility and prevent infection but also dynamically respond to the body’s changing needs, perhaps releasing drugs or signaling molecules on demand?
That’s a fantastic point! The potential for dynamic, responsive coatings is truly exciting. Imagine coatings that adapt to inflammation by releasing anti-inflammatory agents, or promote bone growth factors based on detected stress levels. The possibilities for personalized implant integration are vast. Thanks for sparking this discussion!
Editor: MedTechNews.Uk
Thank you to our Sponsor Esdebe
So, we’re using nature’s blueprints now? Does this mean I can finally blame evolution for my terrible handwriting when the AI-powered doctor asks for a sample on my next check up? Maybe nature can inspire a better filing system for my tax receipts too!
That’s a hilarious take! I hadn’t considered blaming evolution for handwriting! On a serious note, the way nature optimizes processes could definitely revolutionize our organizational systems. Imagine AI learning from ant colony optimization to manage tax filings! The possibilities are endless.
Editor: MedTechNews.Uk
Thank you to our Sponsor Esdebe